Advancements in Knockout Mice Models for Research
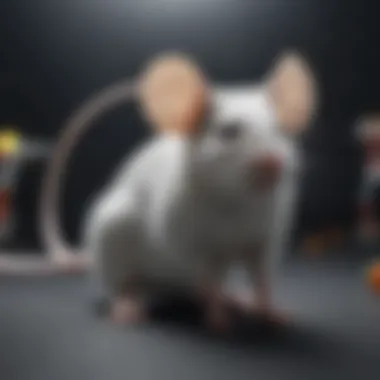
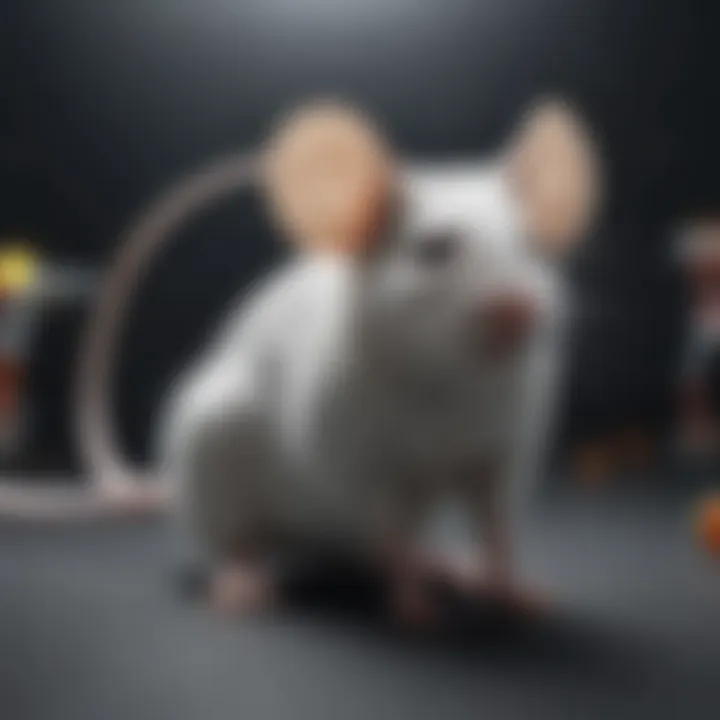
Intro
The world of genetic research stands on the shoulders of innovative methodologies, one of which is the knockout mice model. Delving into the realm of gene function through targeted gene deletion offers profound insights that can shift our understanding of biological systems and disease mechanisms. This article will navigate the landscape of knockout mice — from their creation techniques to the various biomedical applications they support, while also contemplating the ethical dilemmas that their use brings to the forefront.
Knockout mice models serve as a vital tool to mimic human diseases, thereby bridging the gap between basic research and clinical application. By removing specific genes, scientists can observe the resulting phenotypic changes, allowing for a clearer picture of how certain genes contribute to health and disease. The ramifications are significant: insights gained from these models can drive therapeutic innovations, inform drug discovery, and contribute to the development of gene therapies.
"Knockout mice have fundamentally reshaped our understanding of genetics and disease, providing both a canvas and a mirror to explore the complexities of living organisms."
As we embark on this exploration, we will highlight the key findings from studies utilizing knockout mice and discuss their relevance in today’s scientific landscape. A closer look will also be given to the methodologies employed in these studies, emphasizing the intricacies involved in creating and utilizing these genetic models.
Research Overview
Summary of Key Findings
Knockout mice have notably contributed to advancements in various fields such as cancer research, neurobiology, and immunology. By knocking out specific genes, researchers have uncovered crucial pathways and molecular targets that are implicated in disease processes. For example, targeting the p53 gene, a well-known tumor suppressor, has paved the way for a deeper understanding of cancer metastasis and the mechanisms of drug resistance. Moreover, knockout mice have become fundamental in drug testing, providing a platform that mirrors human physiology more closely than traditional cell cultures.
Key findings resulting from knockout studies can include:
- Identification of gene functions crucial to cellular processes.
- Discovery of potential drug targets for numerous diseases.
- Insights into developmental processes and genetic disorders.
Importance of the Research
The knock-out mouse model holds immense significance in modern biomedical research. It enables scientists to conduct experiments that were previously thought impossible. The ability to dissect specific genes in a controlled environment allows researchers to:
- Develop targeted therapies that are tailored to genetic profiles.
- Understand the genetic basis of diseases, aiding in the development of preventive measures.
- Explore gene-environment interactions and their implications for health.
Conversely, the use of knockout mice is not without its challenges. Ethical considerations around animal research are paramount, invoking discussions about humane treatment and the overall goals of such studies. Navigating these waters is essential to ensure that scientific advancement does not come at the cost of ethical integrity.
Methodology
In examining the knockout mice model, understanding the study design and the data collection techniques is crucial.
Study Design
Creating a knockout mouse typically involves sophisticated genetic engineering techniques, predominantly CRISPR-Cas9, which has simplified the process significantly. The design revolves around removing or altering specific genes to assess their function and contribution to diseases. Using precise targeting, scientists can create models that reflect specific genetic conditions, providing invaluable insights into the mechanisms of disease.
Data Collection Techniques
Data collection in studies utilizing knockout mice employs various techniques to capture the complexities of gene expression and phenotypes. Methods may encompass:
- Genotyping to confirm the knockout at the DNA level.
- Behavioral assessments to observe alterations in activity, cognition, and social behaviors.
- Physiological measurements, including blood tests and imaging, to track health parameters.
In summary, the knockout mice model not only exemplifies a pivotal shift in genetic research but also showcases the delicate balance between innovation and ethics in the pursuit of knowledge.
Prelims to Knockout Mice
The knockout mice model has emerged as a cornerstone of genetic research, providing valuable insights into the functioning of genes. The ability to deliberately alter genetic composition enables researchers to analyze and interpret the roles of specific genes in various biological pathways. This discussion lays the groundwork for understanding the broad implications of knockout mice in biomedical research, including their significant contributions to gene function analysis and disease modeling.
Creating knockout mice involves intentionally disabling specific genes, an approach that allows for a controlled study of the resulting physiological and behavioral changes. This targeted deletion provides a clear picture of how genes influence health and disease, making knockout mice an indispensable resource in laboratories worldwide.
Definition and Development
Knockout mice are genetically modified organisms where certain genes have been deactivated or "knocked out." The process typically entails sophisticated genetic engineering techniques. These methods ensure that the selected gene’s function can be investigated through observation of the resultant phenotypic changes. The term "knockout" specifically refers to the intentional gene disruption aimed at revealing its normal function.
Development of knockout mice has evolved significantly since the first successful attempts in the late 1980s. The introduction of techniques like homologous recombination paved the way for precise gene targeting. For instance, using this technique, researchers can replace a gene with a non-functional version. More recent innovations, such as CRISPR-Cas9, further enhanced the ability to create knockout models with unprecedented speed and efficiency. This evolution reflects a growing understanding of genetic manipulation and its profound implications in science.
Historical Context
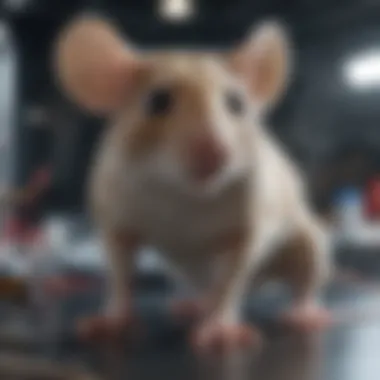
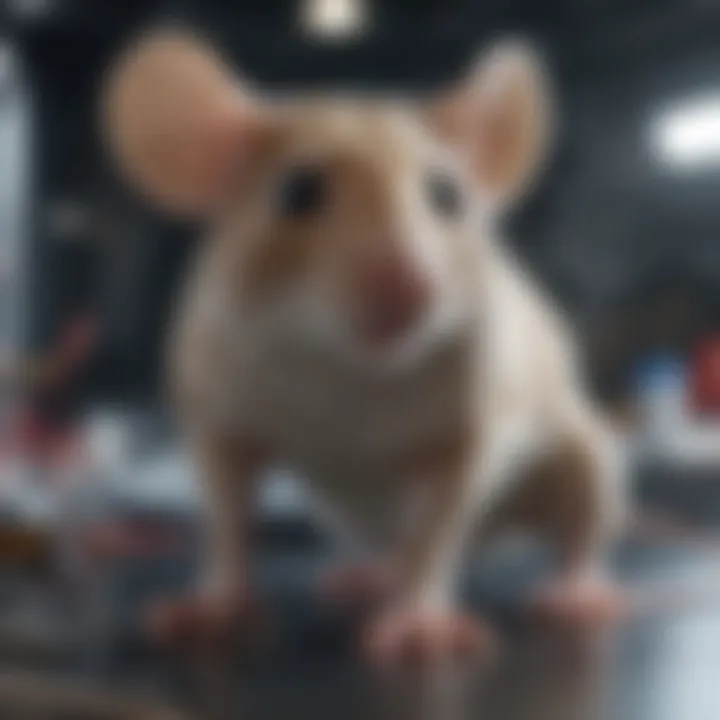
The historical backdrop of knockout mice dates back to the mid-20th century, when the principles of genetics started finding their way into practical application. Initially, researchers relied heavily on conventional breeding methods to study gene effects, but these approaches were often insufficient for isolating specific gene functions. With technological advancements, particularly in molecular biology, the development of knockout mice provided a novel avenue for exploration.
The first knockout mouse was reported in 1989, representing a pivotal moment in genetic research. Philibert and his team at the University of California performed a landmark experiment that established the feasibility of gene targeting in mice. This advance sparked a surge of interest in transgenic organisms and led to an increased understanding of various diseases, including cancer and neurological disorders.
The creation of knockout mice has helped bridge the gap between fundamental genetic research and practical medical applications. Over the years, these models have become essential in elucidating complex genetic diseases and stress the importance of genetic factors in human health, thereby shaping the future of biomedical research.
The Science Behind Knockout Models
Understanding the science behind knockout models is crucial in appreciating their role in modern genetics and biomedical research. These methods allow for precise modifications of the genomes of living organisms, especially mice, which are frequently used as model organisms. Knockout models facilitate an in-depth exploration of genetic functions and disease mechanisms. This section elaborates on pivotal genetic engineering techniques and the processes involved in selecting target genes, each contributing to the overarching goals in biomedical advancements.
Genetic Engineering Techniques
CRISPR-Cas9 Methodology
The CRISPR-Cas9 methodology has revolutionized genetic engineering and stands out for its precision and efficiency. This technique enables researchers to edit specific sequences of DNA within organisms by employing a guide RNA to direct the Cas9 enzyme to the exact location in the genome. One of the key characteristics that make CRISPR-Cas9 so advantageous is its adaptability—researchers can easily modify the system to target a wide variety of genes. Moreover, the simplicity of design and rapid results make CRISPR a popular choice in knockout studies.
However, the technique's unique ability also comes with its downsides. Off-target effects, where the enzyme inadvertently modifies unintended areas of the genome, can occur and may lead to misleading results or complications in interpreting data. Still, the benefits significantly outweigh the drawbacks, and its wide adoption across various research disciplines attests to its importance.
Transgenic Approaches
Transgenic approaches involve introducing foreign DNA into the genome of mice, which can also include the knockout of specific genes. A key characteristic of this method is its ability to create stable lines of mice that consistently express or lack a particular gene. Because it allows for the exploration of gene function in a living organism over multiple generations, it becomes a beneficial choice for understanding complex genetic interactions.
One of the unique features of transgenic approaches is their versatility; they can be employed to study a myriad of conditions, from metabolic diseases to behaviors related to neurological disorders. However, they also present challenges, such as the lengthy process of establishing stable lines and the potential for unpredictable integration sites, which might confound results. Nonetheless, the insight gained from these models contributes greatly to the field of genetics.
Homologous Recombination
Homologous recombination is a technique that underpins traditional knockout models created prior to the rise of CRISPR. This method relies on the natural process of genetic recombination to replace a target gene with a non-functional sequence. The primary characteristic of homologous recombination is its ability to induce specific and targeted gene modifications, offering a reliable way to study gene function.
The process requires a fair bit of finesse and logistical planning, making it less popular compared to newer methods. However, it has its own virtues, including the potential to create precise knockouts without undesirable external insertions. One drawback, however, is the less predictable efficiency, as achieving recombination can be quite variable.
Target Selection and Design
Identifying Genes of Interest
Identifying genes of interest is a foundational element in developing successful knockout models. This process involves pinpointing the genes that are believed to play significant roles in specific diseases or biological processes. The ability to choose which genes to target provides researchers the unique advantage of delving deeper into understanding gene functions and their contributions to various conditions.
One of the notable aspects of this step is its reliance on bioinformatics tools and databases that help elucidate gene function. While the resources available make this selection process increasingly efficient, it can still be a daunting task due to the sheer volume of genetic information and the complexity of gene interactions within pathways. Therefore, thorough analysis is crucial to ensure that the selected gene truly warrants further exploration.
Specificity and Efficiency of Knockouts
The specificity and efficiency of knockouts are paramount to the success of knockout models. Achieving a high level of specificity means that the gene of interest is accurately targeted, reducing the likelihood of unwanted effects that could skew results. High efficiency is equally important, as it determines how successfully a knockout can be created across a population of mice.
One key element to improving specificity is the careful design of guide RNAs in CRISPR applications, ensuring they bind only to the desired genes. However, while advancements have been made, maintaining high efficiency and specificity simultaneously can be complex.
The challenge lies in the balance; a method that excels in efficiency may sacrifice specificity, leading to unintended phenotypic changes. Observing these changes can complicate the interpretation of results and might mask the potential therapeutic benefits that knockout models are meant to elucidate.
In summary, the science behind knockout models encapsulates complex methodologies and the significance of careful planning and execution. The integration of various genetic engineering techniques combined with meticulous target selection ultimately strengthens the potential for groundbreaking discoveries in biomedicine.
Applications in Biomedical Research
Understanding the applications of knockout mice in biomedical research is crucial, as these models offer profound insights into gene function and disease mechanisms. Their use spans various scientific domains, providing vital contributions to our knowledge of biological processes and potential therapeutic interventions. Knockout models, by eliminating specific genes, help researchers dissect the roles of those genes in health and disease. This section delves into the diverse applications, from gene function analysis to modeling complex human diseases, and highlights their significance in drug development and testing.
Gene Function Analysis
Knockout mice serve as an invaluable tool for gene function analysis. By selectively disabling a particular gene, scientists can observe the resultant phenotypic changes, thus revealing the gene's role in various biological pathways. This not only aids in validating the function of hypothetical genes but also establishes connections between genetic mutations and specific traits.
For example, if researchers knock out the Bdnf gene, known to be involved in neurodevelopment, they might observe modifications in behavior, cellular signaling, and anatomical structure in the brain. Such insights are not just critical for academic research; they also pertain significantly to understanding genetic underpinnings of diseases.
Modeling Human Diseases
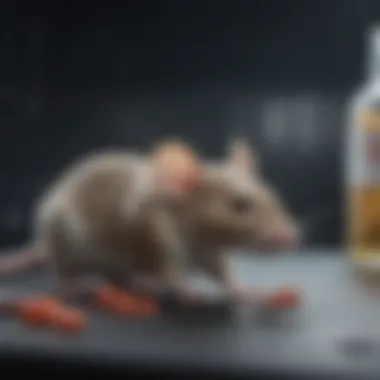
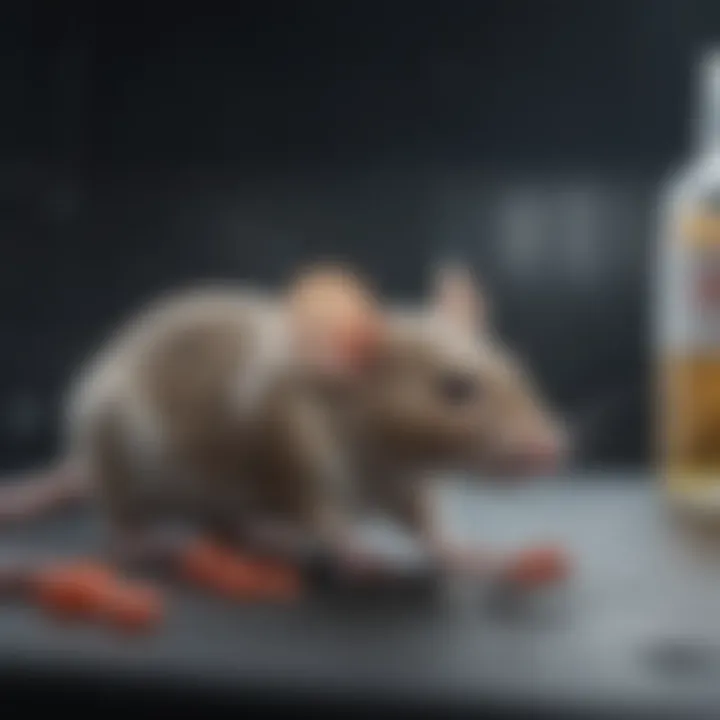
Modeling human diseases through knockout mice provides researchers with a lens to explore the complexities of conditions that afflict humans. The versatility of knockout models allows for the simulation of a variety of illnesses, giving researchers the opportunity to study disease progression, underlying mechanisms, and response to treatment. Below, we dive into specific areas of concern:
Cancer Research
Cancer research is particularly prominent in the context of knockout mice models. By disabling genes related to tumor suppression or those known to drive cancer progression, researchers can better understand the molecular alterations that lead to malignancies. A notable feature is the ability to study gene-environment interactions, which often play a critical role in cancer etiology. The establishment of orthotopic models enables a homely environment for tumors, providing insight into natural tumor behavior and potential metastasis patterns.
However, while valuable, these models can sometimes fail to replicate all aspects of human cancers due to species differences, leading to potential misinterpretations of therapeutic efficacy. Despite this challenge, the contribution to understanding cancer biology is immense.
Neurological Disorders
The realm of neurological disorders benefits significantly from knockout models, allowing for the exploration of conditions like Alzheimer’s or Parkinson’s disease. When genes implicated in neurodegeneration are knocked out, researchers can observe the consequences on cognitive functions, synaptic plasticity, and overall brain architecture. The APP knockouts for Alzheimer's disease research exemplify this approach, shedding light on amyloid plaque formation and cognitive decline over time.
The unique advantage here lies in the ability to evaluate behavioral changes alongside biochemical and histological analyses, providing a multi-faceted view of the disorder. However, a limitation is posed by the complexities of human neurobiology, sometimes making it tricky to thoroughly model more intricate aspects of human conditions.
Metabolic Disorders
Metabolic disorders, ranging from obesity to diabetes, are also effectively modeled using knockout mice. By targeting genes that regulate metabolic pathways—like Lepr for leptin signaling—researchers can investigate how disruptions in these pathways lead to disease states. This area shines due to the straightforwardness of measuring metabolic phenotypes, making it easier for researchers to correlate genetic changes with physiological outcomes.
While the benefits of such models are weighty, they also face scrutiny. The metabolic responses in mice can vary significantly from humans because of differences in lifestyle, diet, and metabolism, leading to potential discrepancies in therapeutic outcomes. Nevertheless, the clarity of understanding gene contributions to metabolism through these models is irrefutable.
Drug Development and Testing
Beyond fundamental research, knockout mice have pivotal roles in drug development and testing. By analyzing how deleting certain genes affects drug metabolism and response, researchers can tailor therapies to enhance efficacy and minimize side effects. Knockout models also aid in early-phase testing by predicting human responses from animal data, though the translation remains an ongoing area of refinement.
For instance, in pharmacogenomics, knockout mice are used to identify how particular genetic variations influence drug metabolism. This enables the development of personalized medicine approaches, where treatments can be optimized on an individual basis, based on genetic backgrounds.
Knockout models not alone broaden the understanding of fundamental biological processes but also directly inform the development pipelines for new therapeutics, making their applications in biomedical research not just significant, but indispensable.
Ethical Considerations
The use of knockout mice in biomedical research opens a can of worms when it comes to ethical implications. The very nature of altering an organism at the genetic level raises significant questions about the morality of such procedures. Ethical considerations should not just be an afterthought; they must be a fundamental part of the discourse around knockout models, guiding the practices and policies of researchers. This section rounds up the crucial elements of ethics that significantly shape the knockout mice narrative.
Animal Welfare
When it boils down to the ethical discussion, animal welfare is the centerpiece. The principle of minimizing suffering is paramount. Researchers are tasked with ensuring that the processes involved in the creation and maintenance of knockout mice do not cause undue stress or pain.
One key consideration is the conditions under which these mice are housed. Labs are required to provide environments that cater to the natural behaviors and needs of these animals. This means keeping them in spacious cages, ensuring they have social interactions, and providing appropriate bedding and nutrition. Moreover, the process of creating knockout mice often involves surgical procedures, such as gene editing, which could introduce pain.
Researchers need to balance the scientific gains against the potential harm to these animals. Often, a cost-benefit analysis is employed. It’s essential for scientists to question if their research truly justifies the changes they enact on animal subjects. The compassion shown for animal life must be evident and integrated into the research methodology.
Additionally, continuous monitoring is vital. There's a pressing need to routinely check on the health and behavior of knockout mice. If they exhibit signs of distress or an unfavorable quality of life, researchers are compelled to take appropriate actions, whether that means medical intervention or even euthanasia to prevent suffering.
"Ethics in research isn’t just about following rules; it’s about embedding compassion within scientific inquiry."
Regulatory Frameworks
Alongside animal welfare, stringent regulatory frameworks govern the use of knockout mice. These regulations are born out of necessity, aiming to set the standard for humane treatment and effective oversight of animal research. Each country has its own guidelines that dictate how researchers must conduct their work concerning animal welfare.
In the U.S., for instance, the Animal Welfare Act outlines the legal obligations research institutions have regarding the use of animals, including knockout mice. This act ensures that any research proposal must detail how the animal's well-being will be respected. Proposals undergo rigorous scrutiny by an Institutional Animal Care and Use Committee (IACUC), which evaluates both the justification for using animals and the methods proposed for minimizing pain and distress.
Moreover, researchers must adhere to the principles of the 3Rs: Replacement, Reduction, and Refinement. Each of these principles emphasizes a commitment to finding ways to use fewer animals, replacing animal models with alternatives when possible, and refining experimental techniques to reduce suffering.
In the European Union, regulations are even more stringent. The Directive 2010/63/EU, for example, governs the ethical use of animals in research, ensuring high standards of animal care and encouraging the use of non-animal technologies.
This regulatory landscape is not just red tape; it ensures accountability and transparency in research practices. Compliance guarantees that research can proceed ethically while upholding scientific integrity. The evolving nature of these regulations also reflects changing societal attitudes toward animal research, indicating that ethical reflection in science is a continuous journey, rather than a destination.
Limitations of Knockout Models
Despite their profound utility in understanding biological processes, knockout models come with a set of limitations that researchers must navigate with care. Acknowledging these limitations not only informs the scientific community about the boundaries of current methodologies but also fosters advancements in the field. These setbacks can hinder the expected outcomes in experiments and necessitate a critical examination of alternatives. Below, we look into some prominent limitations of knockout models that mandatorily require robust consideration during experimental design.
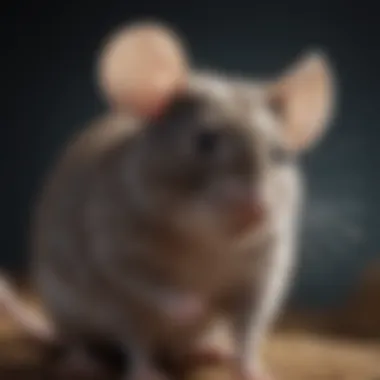
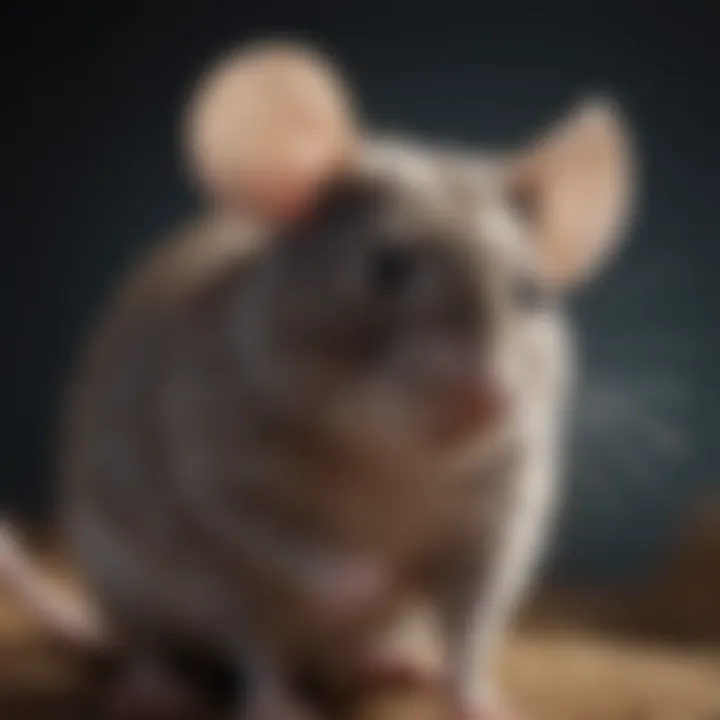
Challenges in Gene Targeting
Achieving precise gene targeting is often more challenging than it may initially appear. While technologies like CRISPR have improved the ability to create knockout mice, the process is laden with potential pitfalls. For instance, off-target effects where unintended genes are altered can lead to confounding results. Researchers may anticipate a straightforward gene knockout, yet experience complications when genes exhibit unexpected redundancy. This redundancy in genetic pathways means that knocking out one gene may not yield observable phenotypic changes if other genes can compensate for its loss.
- Technical difficulties: The efficiency of gene targeting remains inconsistent. Even with sophisticated tools, targeting specific loci can vary significantly between models.
- Complexity of genetic interactions: Understanding the nuanced interactions between genes can complicate predictions about phenotypic outcomes.
The challenge lies not only in precision targeting but also in evaluating how removed genes influence other systems in the organism. These hurdles underscore the need for continual refinement of techniques and greater understanding of genetic networks in mice.
Interpreting Phenotypic Outcomes
Interpreting the results from knockout models can be a real brain teaser. Phenotypic outcomes may not be straightforward or directly linked to expected results. Sometimes, researchers find that the anticipated traits do not manifest—or worse, that unexpected traits arise. This incongruity can occur due to genetic redundancy, compensatory mechanisms, or the complex interplay of gene networks. Researchers might plan a study hypothesizing a clear genetic response, only to be met with a murky array of outcomes that blur the line between cause and effect.
- Variability in expression: Different environmental factors, like diet or stress, can affect gene expression in knockout models, resulting in a diverse range of observed phenotypes.
- Limitation in extrapolation: Results from mice may not fully translate to humans or other species, complicating the application of findings in broader contexts.
Interpreting knockout mouse outcomes requires careful consideration of underlying genetic architecture and environmental interactions.
This complexity can make it easy to misidentify the importance of a particular gene in disease models, leading to potential misinterpretations in the field. To sum it up, the limitations present in knockout models remind us that while they are powerful tools in biomedical research, they are not panaceas for all genetic inquiries. As research progresses, so too must our approaches to these intricacies, ensuring rigorous analysis of results and mindful acknowledgment of their limitations.
Future Directions
The exploration of knockout mice models is an evolving field with tremendous implications for scientific advancement. As researchers continue to refine techniques and methodologies, the potential applications of these genetic tools are expanding rapidly. The future directions highlighted here will illustrate key areas in which knockout models can enhance research capabilities, streamline collaborative efforts, and pave new paths for understanding complex biological systems.
Enhancing Knockout Techniques
Advancements in genetic engineering techniques hold the key to more effective knockout mice. Technologies such as CRISPR-Cas9 have revolutionized the ability to induce targeted genetic alterations with precision. This robustness allows scientists to knock out multiple genes simultaneously, leading to models that better reflect the multifactorial nature of diseases. Enhanced precision means fewer off-target effects and more reliable results.
Moreover, there is potential for the development of more sophisticated targeting approaches. For instance, researchers are investigating the utility of inducible knockout systems, where gene deletion can be controlled at specific stages in an organism's development or in response to environmental triggers. Such flexibility would afford dynamic experimental designs, yielding richer insights into gene functions across various developmental windows.
Integration with Other Models
Integrating knockout mice with other experimental models can provide a multi-faceted view of biological problems. For example, combining knockout models with cell culture approaches or organoid systems allows researchers to study gene function within relevant tissue architectures. This interconnected strategy can reveal how gene deletions impact not just individual cells, but entire biological systems.
Additionally, the amalgamation of knockout models with computational modeling can lead to more robust predictions. Understanding the interactions between knocked-out genes and other biological components can be enhanced through simulations, creating a feedback loop between experimental results and theoretical frameworks. Such integration seems promising for defining pathways involved in diseases more clearly.
Expanding Research Horizons
As the capabilities of knockout mice evolve, so too do the fields of research in which they can be utilized. The exploration of non-traditional therapeutic areas, such as understanding microbial interactions in the gut, is becoming increasingly relevant. By knocking out specific genes linked to immune response or metabolism, researchers can uncover the underlying mechanisms of health and disease, particularly in chronic conditions.
Furthermore, there's a growing interest in using knockout mice to study behavioral changes linked to genetic alterations. This area of research is poised to provide insights into how genes influence behavior and cognitive functions, opening new avenues in psychology and neurology.
"The ability to precise genetic changes in model organisms marks a significant stride in biomedical research, unlocking potentials previously thought unattainable."
In summary, the future of knockout mice models is ripe with possibilities. By enhancing knockout techniques, integrating them with other models, and expanding horizons into new research territories, scientists are not only generating novel insights but also pushing the boundaries of our understanding in numerous scientific fields.
Summary and Closure
The knockout mice model stands as a cornerstone of modern genetic research, proving invaluable in our quest to untangle the complexities of gene function and its broader implications in biomedicine. In this article, we have traversed the intricate methodologies involved in the creation of these models, their diverse applications across various medical fields, and the ethical considerations that come into play with their use. By elucidating both the triumphs and the tribulations linked to knockout models, we have underscored their critical importance in advancing our understanding of biological processes and the mechanisms of disease.
Recapitulation of Key Insights
Throughout the discourse, we revisited several core insights:
- Definition and Relevance: Knockout mice are specially engineered animals that have had specific genes intentionally disabled, allowing researchers to observe the effects of gene loss in real-time.
- Technological Advances: The advent of CRISPR-Cas9 technology has revolutionized gene editing, enabling more precise and efficient knockout models than ever before.
- Broad Applications: These models have become indispensable tools in understanding diseases, especially in areas like cancer and neurological disorders, where they facilitate the modeling of pathological processes.
- Ethics and Oversight: The necessity of balancing scientific advancement with ethical responsibilities is an ongoing challenge that requires adherence to rigorous regulatory frameworks to ensure animal welfare.
Through these points, it is clear that knockout mice not only serve as models for studying gene functions but also act as critical platforms for developing new therapeutic strategies, enriching our comprehension of human biology.
Implications for Future Research
Looking forward, the implications of knockout mice in the realm of biomedical research are profound. Some key areas where future research may focus include:
- Enhancement of Gene Editing Techniques: Innovations in knockout methodologies are likely to evolve, further refining precision and reducing unintended effects.
- Integration with Other Models: There is potential for knockout mice to be combined with other genetic models, including transgenic and conditional knockout systems, to create more sophisticated platforms for studying gene interactions and compensatory mechanisms.
- Expansive Research Horizons: As we deepen our understanding of the genome and its complexities, the scope of research utilizing knockout mice is set to widen, potentially leading to breakthroughs in gene therapy and personalized medicine.
"Continued exploration and adaptation of knockout mice models will not only streamline our approach to biomedical research but may also hasten the development of groundbreaking therapies that address some of humanity's most pressing health challenges."
As the field of genetic research develops, knockout mice will remain at the forefront, symbolizing the profound intersection of science, ethics, and innovation. It is essential for researchers to remain engaged with the evolving landscape of genetic research, using knockout models to illuminate pathways that hold promise for future discoveries.