In-Depth Insights into Trypsin Enzyme Functions
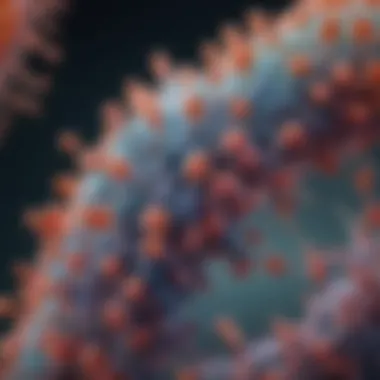
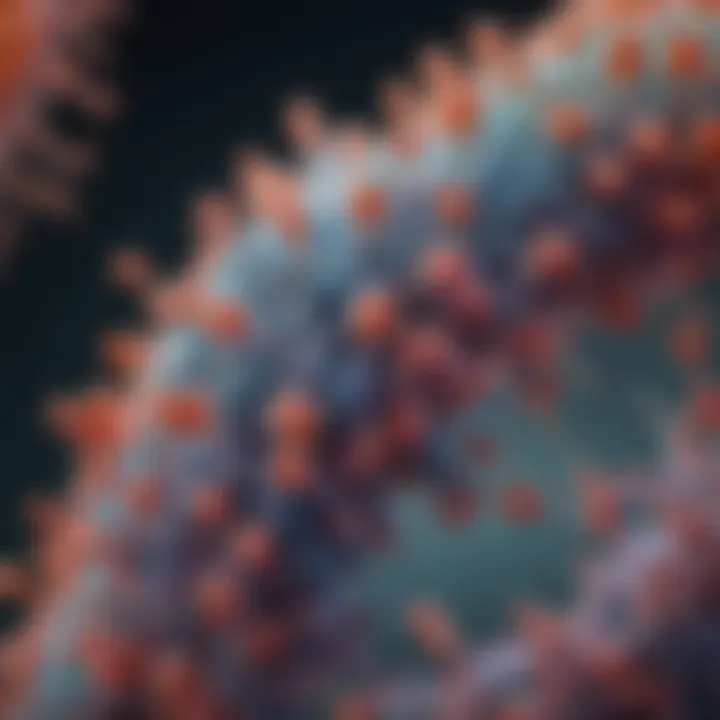
Intro
Trypsin is an essential serine protease enzyme that plays a crucial role in the digestive system. It is primarily produced in the pancreas and secreted into the small intestine, where it facilitates the breakdown of proteins into amino acids. Understanding trypsin involves delving into its structure, mechanism of action, regulation, and its applications in various scientific and medical domains. This enzyme not only illustrates fundamental biochemical processes but also emerges as a pertinent topic across diverse fields including healthcare, biotechnology, and nutrition.
The significance of trypsin arises from its multifaceted roles, especially in digesting dietary proteins. By hydrolyzing peptide bonds, it aids in nutrient absorption, which is vital for maintaining physiological functions. Moreover, its relevance extends beyond mere digestion, influencing areas such as enzyme replacement therapies and food processing. This comprehensive analysis aims to provide a detailed understanding of trypsin, its essential functions, mechanisms, and varied applications.
In this article, we will explore the biochemical intricacies of trypsin, offering insights for students, researchers, educators, and professionals engaged in related fields. By examining its structure and interaction with substrates, we can elucidate trypsin's significance in both physiological and experimental contexts, ultimately highlighting the enzyme's vital roles.
Intro to Trypsin
Trypsin plays a crucial role in the digestive system as an important enzyme that helps in breaking down proteins. Understanding trypsin is fundamental for several reasons. Firstly, it serves as a model for studying enzyme function and mechanisms. This informs a broader understanding of proteases, proteins that play a vital role in various biological processes. Secondly, the implications of trypsin research extend to medical and industrial applications, making it a topic of significant interest outside purely academic circles.
The exploration of trypsin encompasses various facets like its biochemical properties, physiological role, and its regulation. Each of these elements contributes to its importance as a study subject. Moreover, comprehending trypsin can aid in the development of therapeutic enzymes and enhance processes in biotechnology and pharmacology.
Overview of Proteases
Proteases, orproteinases, are enzymes that catalyze the hydrolysis of peptide bonds in proteins. They are essential for many biological functions, including digestion, cell signaling, and immune response. Proteases can be classified based on their structure and function, but they generally fall into two main categories: serine proteases and cysteine proteases. Trypsin belongs to the serine proteases group, characterized by using serine residues for their catalytic activity.
The specificity of proteases determines their role in physiological processes. For instance, trypsin has a strong preference for cleaving peptide bonds at the carboxyl side of the amino acids lysine and arginine. This specificity is crucial for protein digestion and regulation of various metabolic pathways.
Historical Context of Trypsin Research
The study of trypsin has a long history that dates back to the early 20th century. Initial research focused on the discovery and characterization of this enzyme, shedding light on its properties and functions in digestion. Early experiments demonstrated its ability to hydrolyze proteins, leading to greater insight into its role in the gastrointestinal tract.
Since then, research on trypsin has evolved significantly. Advances in molecular biology and biochemistry have allowed scientists to delve into its structure-function relationships. This includes determining the amino acid sequence of trypsin and further exploring its active site configuration. Major breakthroughs have also been made in understanding how trypsin interacts with various substrates, which has profound implications for both health and disease.
In summary, the study of trypsin not only enhances our understanding of proteases but also has widespread applications in medicine and biotechnology. This background sets the stage for a comprehensive analysis of trypsin, covering its unique properties and the various roles it plays in different fields.
Biochemical Properties of Trypsin
Understanding the biochemical properties of trypsin is crucial. These properties provide insight into how this enzyme operates within the digestive system and other applications. The composition and configuration of trypsin directly influence its functionality. Knowledge in this area facilitates advancements in medical and industrial applications. A comprehensive grasp of trypsin's biochemical properties informs researchers and practitioners alike about its potential uses and limitations.
Molecular Structure
The molecular structure of trypsin is fundamentally important to its function. It consists of a specific arrangement of amino acids that contribute to its enzymatic capabilities.
Amino Acid Composition
Trypsin is made up of a unique combination of amino acids. This composition is essential for maintaining the enzyme's stability and functionality in various environments. For example, the presence of serine as a key amino acid plays a crucial role in its catalytic function.
One key characteristic is that trypsin typically contains more than 200 amino acids. This length allows for complex folding, which provides the three-dimensional shape necessary for its biological activity. The highly conserved regions among serine proteases, like trypsin, enhance its recognition and reaction with peptide substrates.
The advantage of this amino acid composition is that it enables trypsin to maintain activity within a range of physiological pH levels, making it suitable for digestive processes. However, this stability can sometimes result in challenges related to inhibitor development when trypsin's activity needs to be regulated.
Active Site Configuration
The active site configuration of trypsin is another pivotal aspect that defines its activity. The arrangement within the active site is exquisitely tailored to bind specific substrates.
A notable characteristic of the active site is the presence of a catalytic triad formed by serine, histidine, and aspartate residues. This arrangement facilitates nucleophilic attack on peptide bonds, making trypsin highly effective at cleaving proteins. The specificity of the active site plays a vital role in ensuring that trypsin selectively cleaves at basic amino acids.
One unique feature of trypsin's active site is its ability to accommodate larger peptide substrates. This offers an advantage when digesting a wide variety of dietary proteins. However, this broad specificity can also be a disadvantage when trypsin interacts with unintended substrates, leading to possible side effects in further biochemical processes.
Enzymatic Activity
The enzymatic activity of trypsin consists of various mechanisms that drive its function as a digestive enzyme. Understanding its activity is essential for leveraging its potential in scientific and industrial contexts.
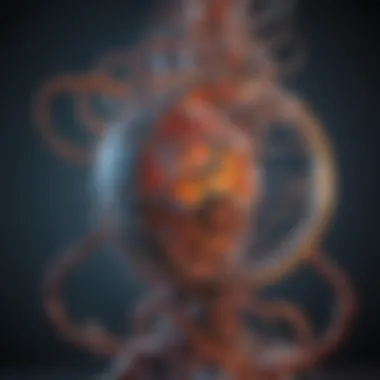
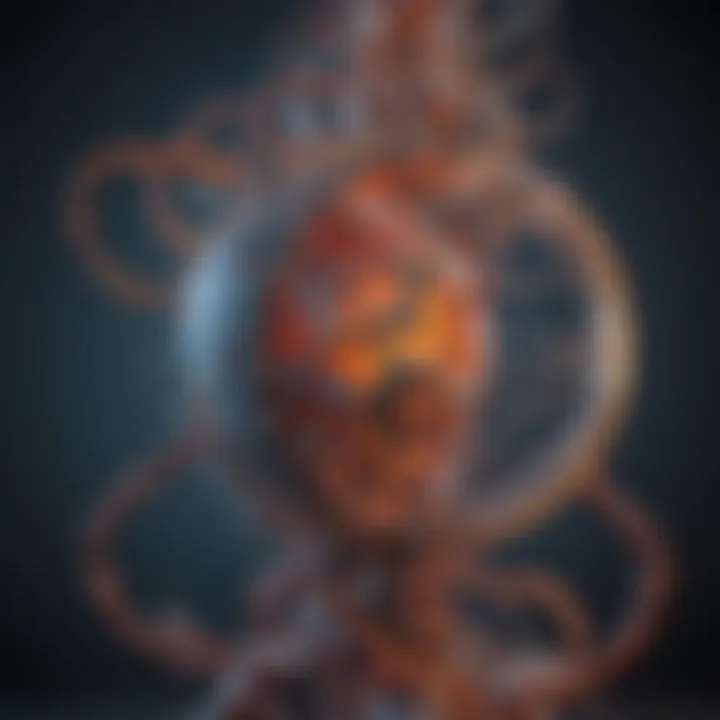
Substrate Specificity
Substrate specificity is a critical element of trypsin's enzymatic activity. It refers to the enzyme's ability to recognize and act on certain peptide substrates. One key characteristic is that trypsin predominantly targets peptide bonds on the carboxyl side of lysine and arginine residues, ensuring effective protein digestion.
This specificity allows trypsin to play an important role in the gastrointestinal process by ensuring complete protein breakdown into absorbable amino acids. This precision also makes trypsin a popular choice in biotechnological applications where targeted cleavage is needed.
The unique feature of this specificity is the evolutionary advantage it provides for trypsin to efficiently process a variety of proteins found in different diets. However, it also poses a limitation in terms of regulating its action when unwanted cleavage of proteins occurs.
Catalytic Mechanism
The catalytic mechanism employed by trypsin is another important aspect of its enzymatic activity. This mechanism involves several steps that contribute to its high efficiency. One key characteristic of trypsin’s catalytic mechanism is the formation of an acyl-enzyme intermediate during the reaction with its substrates.
This intermediate plays a crucial role in stabilizing the transition state, thereby lowering the activation energy necessary for the reaction to proceed. As a result, trypsin can catalyze peptide bond hydrolysis at an effective rate, crucial for digestive functions.
A unique feature of this catalytic mechanism is its ability to operate under various physiological conditions. This versatility is beneficial, allowing trypsin to maintain activity across different environments. However, variations in temperature and pH can result in fluctuations in activity, posing challenges in controlling trypsin's effectiveness in specific applications.
The Role of Trypsin in Digestion
The presence of trypsin in the digestive system is essential. It plays a crucial role in breaking down proteins into smaller peptides. This process is vital for nutrient absorption. Trypsin is produced in an inactive form, trypsinogen, to prevent damaging the cells that produce it. The regulation of its activation and function is intricate, enhancing the body's ability to efficiently digest food.
Synthesis and Activation
Production in the Pancreas
The pancreas synthesizes trypsinogen, the inactive precursor of trypsin. This synthesis occurs in the pancreas's acinar cells. A key characteristic of this process is that it ensures the enzyme is not active during its production. This is critical to prevent self-digestion of pancreatic tissue. The production of trypsinogen is influenced by dietary intake and hormonal signals, such as cholecystokinin. It is a beneficial choice for digestion because its quick activation maintains digestive efficiency. In terms of advantages, the pancreas's production of trypsinogen avoids premature activity, which could be harmful. However, if production is insufficient, protein digestion may be compromised.
Activation from Trypsinogen
Activation of trypsinogen occurs in the small intestine, mainly through the action of enterokinase. This process converts trypsinogen into active trypsin. A critical aspect of this activation is its resulting cascade effect; activated trypsin can catalyze the conversion of more trypsinogen to trypsin, amplifying digestion. This step is beneficial as it accelerates protein breakdown during digestion. A unique feature is that trypsin can also activate other proteolytic enzymes, enhancing the overall digestive process. However, if the activation is excessive, it can lead to pancreatitis, a condition characterized by inflammation of the pancreas. This caution illustrates the importance of tight regulation in the digestive system.
Function in the Gastrointestinal Tract
Protein Digestion
Trypsin's primary function is protein digestion, breaking down peptide bonds at specific sites on proteins. It recognizes substrates based on their amino acid sequences, primarily targeting lysine and arginine residues. A key characteristic here is its specificity, allowing it to effectively cleave proteins into smaller peptides. This function is beneficial because it facilitates further breakdown by other enzymes, improving the efficiency of nutrient uptake. However, if trypsin is not functioning optimally, it may lead to incomplete protein digestion, impacting nutrient absorption and overall health.
Interaction with Other Enzymes
Trypsin does not work alone in the gastrointestinal tract. It interacts with various other enzymes such as chymotrypsin and carboxypeptidase. This interaction is crucial for a coordinated digestive response. A key characteristic is that trypsin activates these other enzymes, creating a network of enzymatic activity that enhances protein breakdown. This collaborative effort is beneficial, as it ensures a comprehensive approach to digestion. An advantage of this synergistic relationship is that it maximizes nutrient absorption. However, if one enzyme in the chain is inhibited or dysfunctional, the entire process can be affected, demonstrating the interdependence of digestive enzymes.
Regulation of Trypsin Activity
The regulation of trypsin activity is crucial for maintaining proper digestive function. Trypsin, a serine protease, is responsible for breaking down proteins into smaller peptides. However, its activity must be tightly controlled. Excessive trypsin activity could lead to autodigestion of the pancreas and other tissues, making regulation essential. This section covers various factors influencing trypsin activity, including inhibitors, activators, and physiological conditions.
Inhibitors and Activators
Endogenous Inhibitors
Endogenous inhibitors play a significant role in regulating trypsin activity. These molecules are produced within the body and help prevent uncontrolled enzyme activity. One key characteristic of endogenous inhibitors is their ability to quickly bind to trypsin, effectively reducing its capacity to digest proteins.
These inhibitors can be very beneficial in maintaining homeostasis in the digestive system. For example, they prevent premature activation of trypsinogen, the inactive form of trypsin. The advantage of using endogenous inhibitors is that they act naturally and work to ensure that enzymes are only active when needed. However, if these inhibitors are imbalanced, it may lead to digestive issues.
Hormonal Regulation
Hormonal regulation also contributes to the control of trypsin activity. Hormones such as cholecystokinin (CCK) and secretin are released from the gut in response to food intake. The key characteristic of hormonal regulation is its ability to modulate enzyme secretion and activity based on dietary needs.
This regulation is beneficial as it allows for a dynamic response to changes in nutrient availability. It ensures that trypsin is produced and activated at the right moment. Nevertheless, hormonal imbalance can lead to either insufficient or excessive trypsin activity, which could have negative effects on digestion and health.
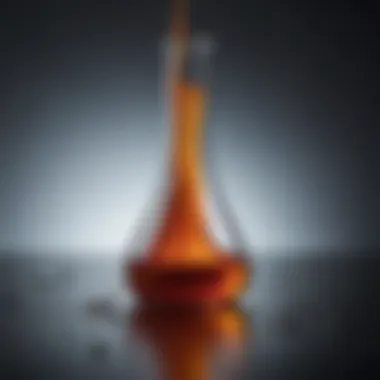
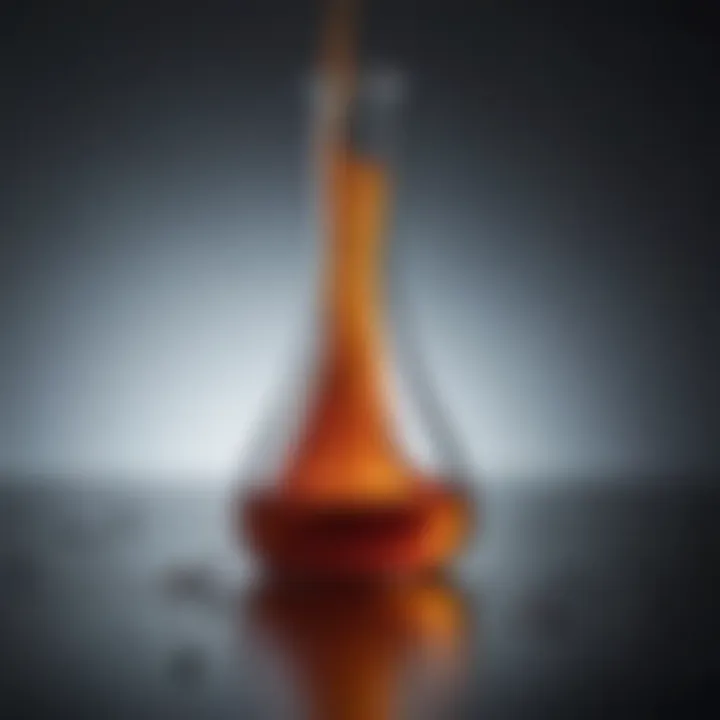
Physiological Conditions
pH Levels
pH levels are another critical factor affecting trypsin activity. Trypsin functions optimally within a specific pH range, typically around 7.5 to 8.5, which corresponds to the alkaline environment of the small intestine. The key characteristic of pH levels is that they can significantly influence enzyme conformation and, subsequently, its activity.
Maintaining proper pH levels is crucial for trypsin to effectively cleave peptide bonds in dietary proteins. If the pH deviates from the optimal range, it can result in reduced enzymatic activity. This is a critical consideration in both digestive health and experimental settings, as fluctuations in pH can impact research outcomes.
Presence of Cofactors
Cofactors also influence trypsin's enzymatic activity. These may be ions or small organic molecules that assist the enzyme in its function. A key characteristic of cofactors is their role in facilitating catalytic processes and enhancing the efficiency of trypsin.
The presence of specific cofactors, such as calcium ions, can stabilize the enzyme structure, allowing it to function optimally. Including appropriate cofactors in research or industrial applications can enhance the success of processes involving trypsin. However, the absence of necessary cofactors may hinder trypsin activity, making it crucial to consider these elements in relevant applications.
In summary, the regulation of trypsin activity involves a complex interplay of endogenous inhibitors, hormonal influences, pH levels, and the presence of cofactors. Understanding these factors is vital for harnessing the full potential of trypsin in both physiological and experimental contexts.
Applications of Trypsin
The applications of trypsin are vast and varied, making it an essential enzyme in numerous fields including medicine, biotechnology, and industry. Understanding these applications helps to appreciate not only the functional significance of trypsin but also its potential in innovative solutions.
In Medical Research
Diagnostic Applications
Trypsin plays a critical role in diagnostic applications, particularly in the development of assays and tests. It is utilized in proteomics to identify and quantify proteins found in biological samples. One notable aspect is its effectiveness in digesting proteins into peptides, which allows for easier analysis through mass spectrometry. This methodology makes trypsin a popular choice in clinical diagnostics. However, the specificity of trypsin can be both advantageous and limiting. While it is effective for proteins that are rich in lysine and arginine, its inability to cleave other sequences may yield incomplete analyses.
Additionally, trypsin's efficiency in procedures such as enzyme-linked immunosorbent assays (ELISAs) highlights its versatility. The reliability of trypsin in these settings reinforces why it is a crucial enzyme in medical diagnostics.
Therapeutic Uses
In therapeutic contexts, trypsin shows promise in treating various conditions. For example, enteric-coated formulations of trypsin are geared towards improving protein digestion in patients with pancreatic insufficiency. The ability of trypsin to break down larger protein molecules into smaller peptides enhances nutrient absorption, which is vital for patient recovery.
While advantageous for therapeutic purposes, some challenges exist. The stability of trypsin in the gastrointestinal tract can be variable, influenced by pH levels and other factors. Ongoing research will allow further exploration of how to optimize its delivery and efficacy, establishing trypsin as not just a tool but as a potential treatment modality.
In Biotechnology
Protein Isolation
Protein isolation is another important application for trypsin in the biotech field. Its specificity allows for the selective digestion of proteins, facilitating their extraction from complex mixtures. Trypsin's action can isolate target proteins through its predilection for cleaving at specific amino acid residues, simplifying downstream processing.
Nevertheless, the requirement for very specific conditions can be a limitation. The ideal environments for trypsin activity must be meticulously maintained to avoid denaturation or inactivation. Overall, though, the efficiency of trypsin in protein isolation remains a significant reason for its adoption in research and industrial settings.
Cell Culture Techniques
Trypsin's role in cell culture techniques is yet another application of importance. It is widely used in detaching adherent cells from culture surfaces. Its utility simplifies the passaging process, essential for maintaining cell lines. The key characteristic that underscores its popularity is its effectiveness in detaching cells without significant damage.
However, the potential drawback is the possibility of over-digestion. If cells are left in trypsin too long, it may lead to detrimental effects on cell viability. This consideration makes it essential for researchers to monitor trypsin exposure closely to maintain cell health.
Industrial Applications
Food Processing
In the food industry, trypsin finds various applications. It is especially significant in processes like meat tenderization, where its ability to break down muscle proteins is utilized. The key characteristic of trypsin in this context is its ability to enhance texture and flavor, improving the overall quality of food products.
However, care must be taken regarding food safety and regulatory measures. Enzyme residues and their effects on final products must be considered to meet safety standards.
Waste Treatment
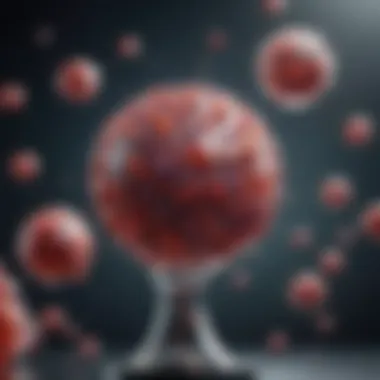
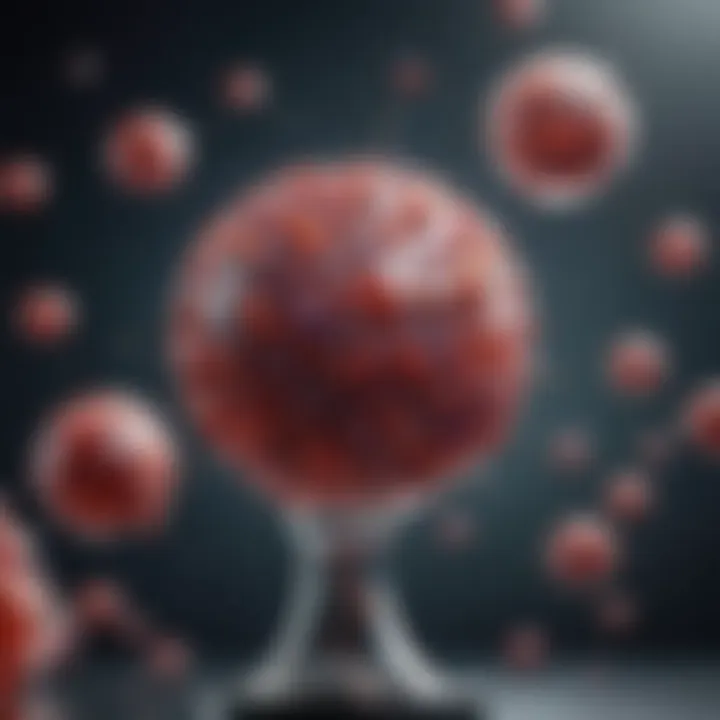
The use of trypsin in waste treatment processes demonstrates its versatility. Trypsin can aid in the breakdown of organic waste materials, promoting more efficient waste management. Its natural origin makes it an attractive option, as it may have fewer environmental impacts than synthetic alternatives. The advantage here is its effectiveness in promoting microbial growth, facilitating the breakdown of complex organic compounds in waste.
However, scaling up trypsin use for large-scale waste treatment can present challenges. The cost of enzyme purification must be balanced against the benefits offered in treatment efficiency. Therefore, further studies are essential to establish protocols that maximize trypsin's advantages while ensuring economic viability.
In summary, trypsin is an enzyme of considerable importance across various applications. Its role in medical diagnostics, therapeutic use, biotechnology, and industrial practices showcases its potential, although challenges remain in some areas. Continued research will undoubtedly yield new insights into optimizing its use, enhancing efficacy and broadening its applications.
Challenges in Trypsin Research
Understanding the challenges in trypsin research is crucial for advancing both fundamental and applied sciences. Despite the extensive study of trypsin, various hurdles still impede progress. Researchers must navigate these complexities to fully leverage the enzyme's potential in diverse applications. This section will delve into specific obstacles, such as inhibitory mechanisms and the differences between in vivo and in vitro studies, that define the current landscape of trypsin research.
Inhibitory Mechanisms
Inhibitory mechanisms pose significant challenges when studying trypsin. The presence of protease inhibitors can interfere with trypsin's activity, complicating experimental results. These inhibitors may be endogenous, originating from the body to regulate digestion or prevent excessive proteolysis. Common examples include pancreatic trypsin inhibitor and α1-antitrypsin. Both play crucial roles in maintaining a balance within the gastrointestinal tract.
When conducting research, recognizing these inhibitors is essential. For accurate assessments, researchers must design experiments that account for varying inhibitor levels. This can involve using specific protease inhibitors or adjusting conditions to minimize their effects. Failure to acknowledge inhibitory mechanisms can lead to misleading outcomes and hinder our understanding of trypsin's true physiological roles.
In vivo vs. In vitro Studies
The choice between in vivo and in vitro studies represents another critical challenge. In vitro studies allow for controlled experiments, facilitating the investigation of trypsin's interactions with specific substrates or inhibitors in a simplified environment. However, these studies may not always reflect the complexity of biological systems.
In contrast, in vivo studies provide insights into trypsin's role in the intact organism. They deliver a comprehensive view of how trypsin functions in digestion, regulation, and interaction with other enzymes. Yet, these studies can introduce variables that are difficult to control, complicating data interpretation.
Researchers often face a trade-off between the precision of in vitro studies and the ecological validity of in vivo studies. Finding a balance that yields meaningful results while acknowledging each study's limitations is continually evolving.
"The interplay between in vitro and in vivo studies is central to deepening our understanding of trypsin and its applications in health and disease."
Navigating these challenges is integral for future advancements in trypsin research. Fostering a nuanced understanding of these obstacles will enhance the design and interpretation of experiments, ultimately leading to more effective applications in biotechnology and medicine.
Future Directions in Trypsin Research
Future research on trypsin holds considerable promise, as it explores innovative ways to harness this enzyme's capabilities. The crucial role of trypsin in biological processes and various applications makes its continued study significant. Understanding how trypsin can be optimized and adapted for different uses is essential for advancements in medical research, biotechnology, and industry. As scientists uncover more about the enzyme's mechanisms and interactions, they can uncover new applications and improve existing methodologies.
Genetic Engineering Approaches
Genetic engineering offers an avenue for enhancing trypsin's properties and functions. By manipulating the genetic material responsible for producing trypsin, researchers can tailor its enzymatic characteristics. For instance, site-directed mutagenesis allows specific amino acid changes. This can lead to improved stability, altered substrate specificity, or enhanced catalytic efficiency. Such modifications may expand the enzyme's use in industrial applications, such as in detergents or food processing, where effectiveness under varying conditions is crucial.
Moreover, synthetic biology can create novel trypsin variants. These could exhibit property enhancements not found in nature. For example, engineered trypsins could have increased resistance to inhibitors that typically reduce their activity. This resilience could pave the way for more efficient processes in clinical and industrial settings.
Innovation in Applications
The potential for trypsin applications continues to expand with ongoing innovation. One promising area is its use in personalized medicine. By understanding individual variations in digestion, healthcare providers may utilize trypsin in tailored therapies. Customized enzyme treatments could optimize protein digestion in patients with specific disorders.
Another innovation is in biotechnology. Enhanced trypsin formulations are increasingly valuable within cell culture processes. They facilitate better isolation and purification of proteins. Improved trypsin products can lead to higher yields and better quality in therapeutic proteins, which is influential in pharmaceutical developments.
Additionally, the food industry seeks better trypsin applications. Process optimization through genetic engineering can address dietary needs and safety concerns while improving nutritional profiles. This could lead to more effective protein sources designed for specific consumer markets.
In summary, the future directions in trypsin research not only promise advancements in biological understanding, but they also offer exciting possibilities for practical applications that can significantly impact medicine and industry.
Epilogue
The conclusion is a crucial part of this discourse, synthesizing the key elements discussed throughout the article. It encapsulates the intricate role trypsin plays not only in digestion but also in broader biomedical and industrial applications. By summarizing vital concepts, this section aids in reinforcing the significance of trypsin in physiological processes and its nuanced mechanisms within various fields of study.
Summary of Key Points
In reviewing the comprehensive analysis of trypsin, several critical points stand out:
- Biochemical Properties: Trypsin is characterized by its specific molecular structure, which plays a fundamental role in its enzymatic activity. Its unique amino acid composition and active site configuration facilitate substrate specificity and catalysis.
- Role in Digestion: The synthesis of trypsin occurs primarily in the pancreas, where it is activated from trypsinogen. Understanding its functionality within the gastrointestinal tract is vital, highlighting its cooperative interactions with other digestive enzymes.
- Regulation: The activity of trypsin is governed through various inhibitors and physiological conditions, such as pH levels and hormonal influences. This regulation ensures proper digestive function and minimizes potential adverse effects within the body.
- Applications: The diverse applications of trypsin stem from its enzymatic capabilities. In medical research, it serves diagnostic and therapeutic purposes. Moreover, in biotechnology, it is essential for protein isolation and cell culture techniques, while its industrial applications extend into food processing and waste treatment.
Implications for Future Research
Future research into trypsin presents numerous pathways. Genetic engineering offers a promising avenue, potentially leading to enhanced enzyme efficiency and specificity. Innovations can further redefine its applications.
- Investigating New Inhibitors: Gaining insights into novel inhibitors could improve therapeutic strategies in treating disorders related to trypsin activity.
- Exploring Modifications: Utilizing recombinant DNA technology may result in modified trypsin variants with tailored properties for specific applications.
- Clinical Applications Expansion: As understanding of trypsin’s biochemical roles deepens, its use in innovative therapeutic approaches may expand, particularly in areas such as cancer treatment and tissue engineering.