Exploring Circular Polarization of Light: Principles & Uses
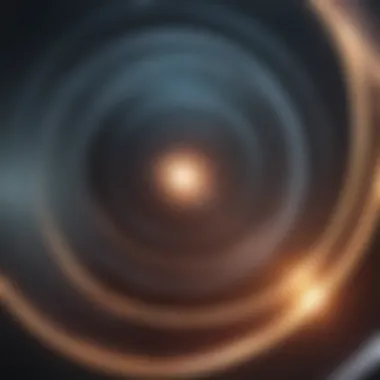
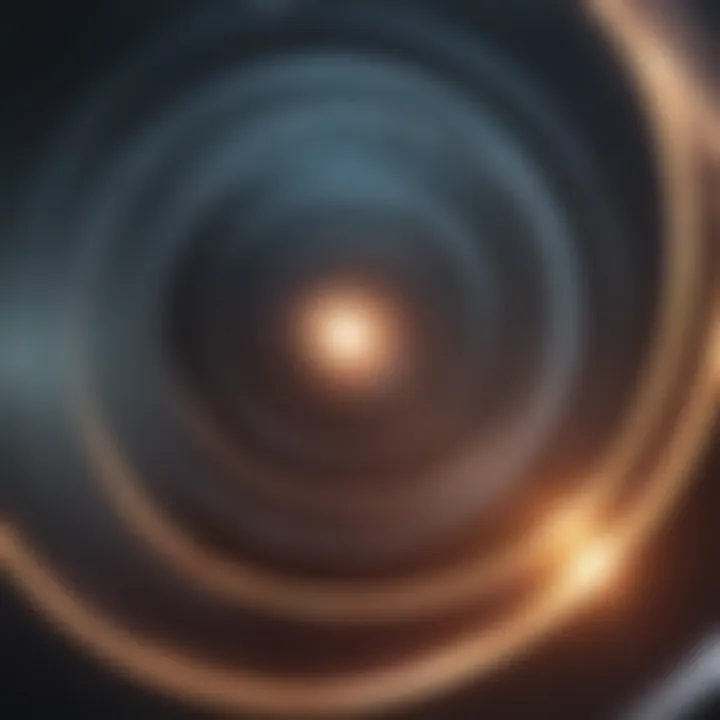
Intro
Circular polarization of light is an essential concept that permeates various scientific and engineering domains. Understanding how this phenomenon works not only enriches our knowledge of fundamental physics but also unveils innovative applications across many industries. From telecommunications to imaging technologies, the implications of circularly polarized light extend far beyond theoretical discussions. This article aims to clarify these concepts, enabling readers to appreciate the significance of circular polarization in modern science and technology.
Research Overview
Summary of Key Findings
Several studies have diligently investigated circular polarization, revealing critical insights:
- Circular polarization consists of two components: left-handed and right-handed polarization, which rotate in a helical fashion.
- Mathematical models, such as Jones calculus and Stokes parameters, provide a framework for describing the behavior and properties of circularly polarized light.
- Recent advancements in materials science, particularly metamaterials, have allowed for more efficient generation and manipulation of circularly polarized light.
These findings underscore not just the mechanics of circular polarization but also its practical relevance to different sectors.
Importance of the Research
Understanding circular polarization is pivotal for several reasons:
- Optics and Telecommunications: Circularly polarized light is crucial in the development of optical fibers and wireless communication technologies, providing enhanced signal clarity and stability.
- Quantum Computing: Applications in quantum technology utilize circular polarization for encoding information, representing a step forward in computational efficiency.
- Imaging Techniques: In medical and environmental imaging, circular polarization aids in better contrast and detail, facilitating improved analysis and diagnosis.
By examining these various applications, researchers can develop novel solutions that harness circular polarization effectively.
Methodology
Study Design
Research on circular polarization typically involves a mix of theoretical and experimental approaches. Theoretical models allow researchers to predict behaviors, while experimental validations help refine these predictions through real-world observations.
Data Collection Techniques
Data collection in this field often utilizes several methods:
- Spectroscopy: Analysis of how different materials interact with circularly polarized light can yield insights into their properties.
- Imaging Systems: Using specialized cameras that can detect circularly polarized light, researchers gather data on material surfaces or biological samples.
- Numerical Simulations: Software tools simulate how circularly polarized light interacts with various material structures, helping in understanding complex systems.
Such methodologies provide a comprehensive understanding of circular polarization, laying the groundwork for innovative applications.
Intro to Polarization
Polarization of light is a fundamental concept in optics that describes the orientation of light waves. It plays a crucial role in various scientific and industrial applications. Understanding polarization helps in harnessing light's properties effectively, influencing how we use optical devices and technologies in fields ranging from telecommunications to materials science. This section will introduce the concept of polarization, laying the groundwork for a more detailed exploration of circular polarization.
Defining Polarization
Light generally oscillates in many directions; however, when it becomes polarized, the waves are restricted to a specific direction. This definition highlights the significance of polarization in designing optical systems. Polarization affects how light interacts with materials and devices. For instance, polarized light can reduce glare and enhance visibility, which is pivotal for sunglasses and photonic applications. The understanding of polarization is vital for anyone studying light properties or applying them technologically.
Types of Polarization
There are several types of polarization, each with distinct characteristics. Grasping these types aids in comprehending the applications of circular polarization.
- Linear Polarization: This type of polarization occurs when light waves oscillate in a single plane. A key characteristic of linear polarization is its simplicity, which makes it easy to generate and control. In terms of benefits, linear polarization has broad applications, such as in LCD screens and photography, where controlling glare is essential. However, it can be limited when dealing with three-dimensional objects or more complex optical systems.
- Circular Polarization: Here, the electric field vectors rotate in a circular manner as the light travels. Its defining feature is that it can be left-handed or right-handed, which is crucial for various applications. Circular polarization is popular in fields like telecommunications and quantum computing because it can efficiently encode information. Its unique property makes it effective for reducing interference effects in transmission environments, although some consider it more challenging to generate compared to linear polarization.
- Elliptical Polarization: This form is a general case of polarization where the electric field describes an ellipse. The important aspect of elliptical polarization is its versatility. It can represent linear and circular polarization as specific cases. While it is beneficial for comprehensive applications, it may prove complex to analyze mathematically, which could be a disadvantage in certain practical scenarios.
Understanding these types provides essential context when discussing circular polarization and its unique contributions. Each form of polarization offers various benefits and limitations, valuable for future explorations in this article.
Fundamental Concepts of Circular Polarization
Circular polarization of light is a vital concept in optics, science, and technology. Understanding its fundamental aspects is essential for both theoretical studies and practical applications. Circular polarization occurs when light waves propagate in a circular motion. This intrinsic property influences how light interacts with matter, making it essential for various fields including telecommunications, imaging, and biological research.
Definition and Characteristics
Circular polarization can be defined as the condition where the electric field of light waves rotates in a circular pattern as the wave travels. This rotation can occur in a clockwise or counterclockwise direction, generating two types: right-handed and left-handed polarization.
One important characteristic of circularly polarized light is that it can pass through certain materials, like quarter-wave plates, which transform linearly polarized light into circularly polarized light. This transformation requires precise alignment and understanding of the material properties involved.
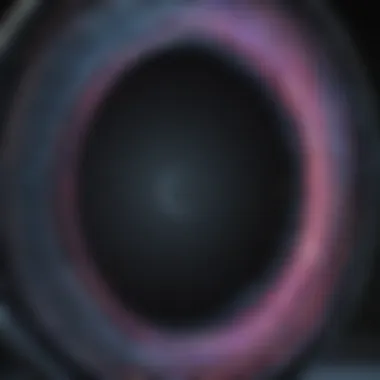
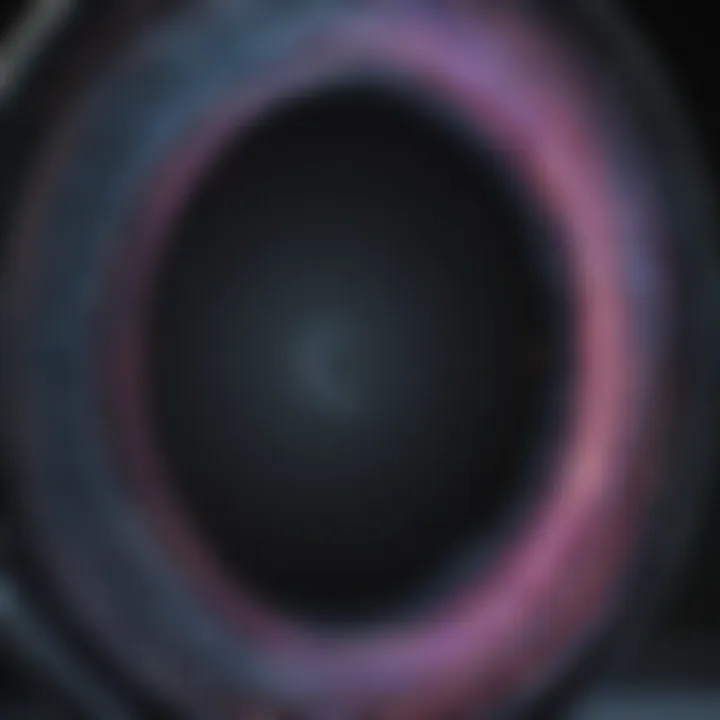
The unique aspect of circular polarization is its ability to maintain its state when reflected or transmitted through materials. It is less susceptible to scattering effects compared to linear polarization. This property enhances its usefulness in many applications, especially in optical systems where stability of polarization is critical.
Mathematical Representation
Circular polarization can be represented through mathematical formulations that describe its behavior quantitatively. Two primary methods of representation are through complex numbers and phasor representation, both contributing to a clearer understanding of the phenomenon.
Complex Numbers
Complex numbers offer a powerful way to express and manipulate circular polarization mathematically. They consist of a real part and an imaginary part, allowing representation of wave functions. For circular polarization, the electric field can be expressed using complex exponential functions. This approach simplifies calculations involved in interference and diffraction methods.
Key characteristics: The significant benefit of using complex numbers is their ability to easily represent oscillatory phenomena, such as the circular motion of the electric field. They succinctly encapsulate amplitude and phase, which are crucial for evaluating interference patterns.
However, this representation demands a good mathematical foundation. Its complexity might pose a challenge for novice learners.
Phasor Representation
Phasor representation is another method that effectively illustrates circular polarization. In this context, a phasor is a complex number that conveys the amplitude and phase angle of a sinusoidal function. When visualized on a complex plane, circular polarization stands out distinctly, showing how the electric field vector rotates around a center point.
Key characteristics: One advantage of phasor representation is its capacity for visual interpretation. It clearly demonstrates the phase relationship between different circularly polarized light waves. This visual clarity aids in understanding phenomena such as superposition and interference.
Phasor representation is usually more intuitive for students and researchers who prefer visual learning methods. However, its reliance on ideal conditions may not encompass real-world complexities, which can sometimes limit its applicability.
In essence, both complex numbers and phasor representation provide different yet complementary ways to understand and describe circular polarization, each with its own strengths and challenges.
Generation of Circularly Polarized Light
Generating circularly polarized light is a crucial process in understanding its unique properties and applications. Circular polarization is vital in many scientific disciplines, including optics and telecommunications. There are two main sources for generating this type of light: natural and artificial.
Natural Sources
Sunlight
Sunlight is one of the most prevalent natural sources of circularly polarized light. When sunlight interacts with certain surfaces, it can become circularly polarized. This phenomenon is significant in nature, influencing biological processes and the behavior of various materials. The key characteristic of sunlight is its broad spectrum, which makes it a rich source of energy. It can provide not only visible light but also ultraviolet and infrared wavelengths.
The unique feature of sunlight is its natural circular polarization, which occurs in certain atmospheric conditions. One advantage of using sunlight is that it is abundantly available and free. However, its polarization can vary significantly depending on environmental factors, which may limit its consistency in experimental settings.
Crystal Refraction
Crystal refraction is another important source of circularly polarized light. When light passes through certain types of crystals, it can emerge as circularly polarized light due to the crystal's inherent optical properties. This process is particularly crucial in applications involving optical filters and specialized lenses. The key characteristic of crystal refraction is its ability to create controlled polarization states.
The remarkable aspect of using crystal refraction is the precision with which it can generate circular polarization. Crystals like quartz and calcite are known to provide reliable results. However, one disadvantage is that the availability of specific crystals and the precision processing can be challenging and costly, limiting their widespread use.
Artificial Sources
Polarizers
Polarizers are essential devices for generating circularly polarized light artificially. They work by allowing light waves of a specific orientation to pass while blocking others. This selectivity is fundamental to the creation of polarized light. The key characteristic of polarizers is their ability to control the polarization state of light precisely. This control is advantageous for experiments requiring specific light conditions.
One unique feature of polarizers is that they can be designed in various forms, including thin films and crystal structures. These designs provide flexibility in applications across different fields. However, a downside to using polarizers is that they may introduce losses in light intensity, which can be a concern in high-precision applications.
Wave Plates
Wave plates are optical devices specifically designed to alter the phase of polarized light. They achieve this by introducing a specific optical path length difference between the light waves. This phase difference is essential for converting linearly polarized light into circularly polarized light. The critical aspect of wave plates is their ability to create desired polarization states with precise control.
One unique feature of wave plates is that they can be tailored to specific wavelengths and applications. This customization enhances their effectiveness in specialized fields, like telecommunications and microscopy. However, wave plates can be sensitive to alignment and environmental conditions, which may introduce challenges during use.
In summary, the generation of circularly polarized light is fundamental for understanding its characteristics and applications in various fields. Through both natural and artificial means, circular polarization provides valuable insights and capabilities in scientific research and technology.
Measurement Techniques for Circular Polarization
Measuring circular polarization is a vital aspect of optics and photonics. This area of study has implications for various applications, such as telecommunications, imaging, and quantum computing. Understanding these measurement techniques allows researchers and professionals to harness circular polarization's unique properties effectively. The ability to accurately measure circular polarization leads to improved optical systems and better data interpretation.
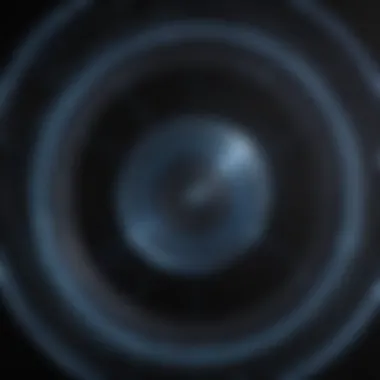
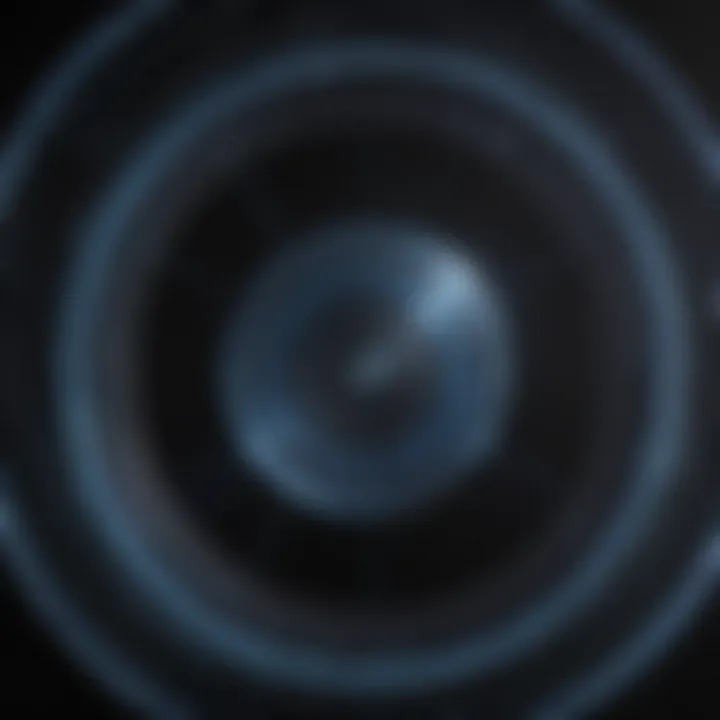
Polarimetry
Polarimetry is one of the primary techniques used to measure the polarization state of light, including circular polarization. It involves using specialized instruments called polarimeters to assess how light interacts with materials. The principal focus of polarimetry is to determine the polarization degree and the specific state of polarization.
Different types of polarimeters exist. A common method uses a polarizer in combination with a detector to analyze transmitted light. In this arrangement, the polarizer filters the incoming light, allowing only specific polarization states to pass through. This is essential for distinguishing between circular and linear polarizations.
Polarimetry provides precise metrics about the properties of light, crucial for various scientific and industrial applications.
The application of polarimetry can be seen in biological research, where it helps to assess molecular structures. Furthermore, advanced polarimetric techniques can discern between various circular polarizations, enabling researchers to extract valuable information about sample characteristics. This method has a significant role in optical imaging, pharmaceuticals, and liquids.
Interferometry
Interferometry is another powerful measurement technique employed in the analysis of circular polarization. It uses the principle of interference of light waves to gather information about their properties, including polarization states. In this method, coherent light waves are divided into two beams which then recombine to create an interference pattern.
These interference patterns are highly sensitive to changes in light's phase, which can be affected by its polarization state. By analyzing the resulting fringe patterns, one can deduce detailed characteristics of circularly polarized light. Variations in the patterns can indicate differences in circular polarization handedness or changes in the optical path length between beams.
Interferometry is particularly useful for high-precision measurements. Applications include optical sensing and the examination of thin films and coatings. This technique is also a critical tool in various research areas such as quantum optics, where understanding the quantum state of light is essential.
In summary, both polarimetry and interferometry play crucial roles in measuring circular polarization. Their complementary nature enriches our understanding of this phenomenon in practical applications, driving advancements in diverse fields.
Applications of Circular Polarization
Circular polarization of light holds significant relevance across various fields. Its unique properties allow for enhanced performance in optical systems, biological research, and emerging technologies in quantum computing. Understanding these applications will illuminate the benefits and considerations linked to circular polarization.
In Optical Systems
Optical Imaging
Optical imaging stands out in the realm of circular polarization application. This method utilizes the specific characteristics of circularly polarized light to improve image clarity and contrast. One key aspect is its ability to mitigate glare caused by reflections on surfaces, which often obscures details in conventional imaging techniques. This makes it a highly beneficial choice for fields like microscopy and photography.
The unique feature of optical imaging that employs circular polarization is its capacity to distinguish different textures and features in objects. By utilizing light that rotates in a circular manner, imaging systems can reveal hidden details not visible under regular light conditions. An advantage of this approach is the improved quality of images captured, enhancing analysis in scientific research and practical applications. However, one downside could be the additional complexity and cost associated with polarized imaging systems.
Telecommunications
In the telecommunications sector, circular polarization provides clear benefits for signal transmission. One fundamental aspect is its efficacy in reducing interference from different sources, such as atmospheric effects. This quality makes it a popular choice for satellite communications and radar systems.
The unique feature of using circularly polarized signals in telecommunications is their consistent performance in transmitting data regardless of the receiver's orientation. This reliability in data integrity stands as a significant advantage over standard linear polarization. However, implementing systems to utilize circular polarization can pose challenges, including compatibility with existing infrastructure.
In Biological Research
Circular Dichroism
Circular dichroism (CD) is pivotal in biological research, utilizing circularly polarized light to analyze molecular structures. This technique allows scientists to deduce information about the secondary structure of proteins and nucleic acids. The key characteristic of CD is its sensitivity to molecular chiral properties, making it indispensable for studying asymmetric molecular environments. Its popularity stems from the relatively simple setup and the ability to collect data on multiple samples simultaneously.
A unique feature of circular dichroism is its capacity to provide real-time insights into dynamic processes, such as protein folding. The advantages include a non-destructive approach and the ability to perform analysis in various states. However, challenges may arise in interpretation, requiring expertise to derive meaningful insights from the data collected.
Staining Techniques
Staining techniques enhanced by circular polarization contribute significantly to cellular and tissue analysis. This application involves the use of circularly polarized light to differentiate between stained and unstained areas in biological samples. The key characteristic of staining techniques is their enhancement of contrast, allowing for clearer visualization of cellular components.
The unique feature of circularly polarized staining is its ability to provide differential contrast without the need for complex staining procedures. Such techniques can yield high-resolution images, facilitating better analysis in histology and cytology. Additionally, they can capture more detailed structures without excessive alteration of the sample. One downside is that specialized equipment may be necessary to utilize this technique effectively.
In Quantum Computing
Qubit Representation
In quantum computing, circular polarization serves as an effective method for qubit representation, essential for executing quantum algorithms. The fundamental aspect of this application lies in its reliance on the quantum properties of light. Circularly polarized photons can represent quantum states, leveraging their spin characteristics for information processing. This makes it a beneficial choice for quantum systems.
A unique feature of using qubits represented by circularly polarized light is the ability to perform complex quantum operations rapidly. Circular polarization reliably encodes information, facilitating error correction and enhancing computational efficiency. However, establishing robust systems for quantum computing with this technique can be technically demanding and resource-intensive.
Quantum Communication
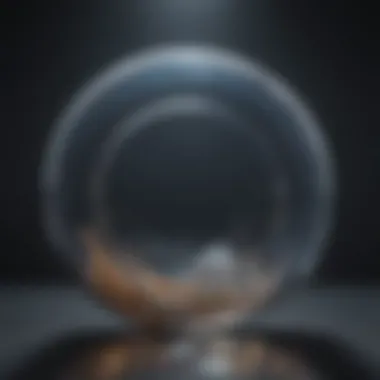
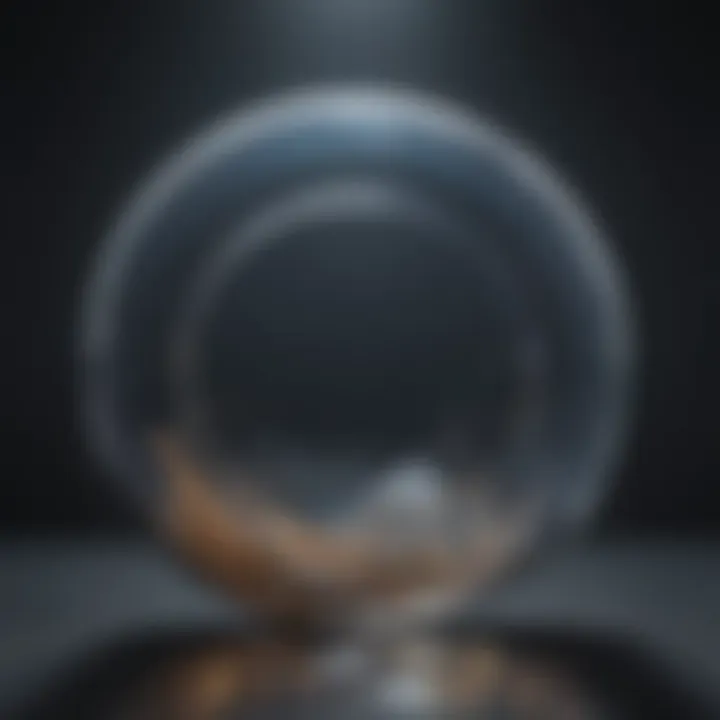
Circular polarization also plays a crucial role in quantum communication by allowing secure transmission of quantum information. The key characteristic of this application is its use of entangled photons, harnessing circular polarization to carry information securely between parties. This aspect makes circular polarization a highly sought-after technique in developing secure communication protocols.
The unique feature of quantum communication utilizing circular polarization is its resilience against eavesdropping. Circularly polarized states can encode information in ways that make interception detectable. This advantage positions quantum communication at the forefront of secure information transfer. However, challenges exist in maintaining the stability of quantum states over long distances, impacting the practical implementation of these systems.
Recent Advances in Circular Polarization Research
The recent advancements in circular polarization research represent a vital intersection between fundamental physics and practical applications. Understanding these advances enables better control over light and material interactions. Researchers have made notable progress in developing technologies that leverage the unique properties of circularly polarized light. This exploration opens new avenues in various fields, such as telecommunications, imaging, and quantum computing.
Emerging Technologies
Metamaterials
Metamaterials have emerged as a transformative component in the realm of circular polarization. These materials possess unique properties that can manipulate electromagnetic waves in ways standard materials cannot. The key characteristic of metamaterials is their engineered structure, which allows for control over the properties of light at the sub-wavelength scale. This feature is particularly beneficial for applications requiring precise manipulation of light.
The use of metamaterials can enhance the efficiency of devices that utilize circular polarization, such as antennas and sensors. One unique aspect of metamaterials is their potential to achieve negative refraction, allowing for inventive designs in optical systems. However, the complexity of their fabrication poses a challenge; creating high-quality metamaterials is not always feasible on a large scale.
Nanophotonics
Nanophotonics, another significant development in the study of circular polarization, involves the manipulation of light at the nanoscale. This field allows researchers to explore the properties of light in new ways, particularly in relation to circularly polarized light. A key characteristic of nanophotonics is its ability to confine light to volumes much smaller than the wavelength, which is essential for advanced optical applications.
The unique feature of nanophotonics is its capacity to enhance interactions between light and matter, paving the way for improved functionalities in sensors, emitters, and other optical devices. While the advantages are substantial, downsides include challenges in fabrication techniques and the need for precise conditions to achieve desired results.
Applications in Material Science
Chiral Materials
Chiral materials are increasingly significant in the context of circular polarization research. These substances have a unique ability to interact with circularly polarized light, resulting in selective absorption or reflection. This characteristic makes chiral materials valuable for various applications, including sensing and biomedical imaging.
The remarkable property of chiral materials is their selectivity, which can enhance the sensitivity of devices that rely on detecting circularly polarized light. They are seen as beneficial because they can lead to advancements in areas such as drug design and diagnostics. However, challenges remain, particularly around the efficient production and integration of these materials into practical applications.
Optical Devices
Optical devices are central to harnessing the benefits of circular polarization. Various devices, such as cameras and optical sensors, rely on this property for enhanced performance. The key characteristic of optical devices designed around circular polarization is their ability to improve image contrast and accuracy.
A unique feature of these devices is their capacity to filter and analyze light signals effectively. They find prominent use in telecommunications and imaging systems, where precision is crucial. The downside may be the complexity and cost involved in manufacturing sophisticated optical devices equipped with circular polarization capabilities.
Challenges and Limitations
Understanding the challenges and limitations associated with circular polarization of light is crucial for advancing research and development in multiple fields. Despite its numerous applications, there are several factors that researchers and practitioners must navigate to optimize the use of circularly polarized light effectively.
Technological Barriers
The path to harnessing circular polarization faces various technological barriers. One major hurdle lies in the production of high-quality circularly polarized light. Many current methods to generate this type of light, like utilizing polarizers and wave plates, often introduce imperfections. These defects lead to a diminished degree of circular polarization, limiting its effectiveness in sensitive applications.
Additionally, the integration of circular polarization techniques in existing optical systems can prove difficult. Many current technologies are designed for linear polarization, requiring significant adjustments or reengineering to accommodate circular polarization. Another issue involves the scalability of these technologies. Producing circularly polarized light sources without compromising on quality or efficiency remains an active area of investigation.
Moreover, there is a notable gap in standardized measurement techniques for assessing circular polarization. The absence of uniform protocols complicates comparative studies across different research institutions. This lack of standardization can prevent successful collaboration and hinder the replication of experiments, which is vital in the scientific community.
Theoretical Challenges
From a theoretical perspective, research into circular polarization involves various complex models and mathematical frameworks. The intricacies of light-matter interaction under circular polarization are not completely understood. This lack of understanding represents a significant challenge, as it limits the ability of scientists to predict how materials will behave when exposed to circularly polarized light.
Furthermore, many theories regarding circular polarization do not account for all variables affecting its behavior. These simplified models often fail to encompass the full range of phenomena depending on different materials or wavelength ranges. As a result, researchers may find themselves at a disadvantage when trying to apply theoretical insights to practical applications.
Epilogue
In this article, we have explored the vital subject of circular polarization of light. Understanding this phenomenon is essential for various scientific and technological applications. Circular polarization is not just an abstract concept; it has practical implications in multiple fields such as telecommunications, optical imaging, and quantum computing. Addressing both the generation and measurement of circularly polarized light enhances our ability to harness its properties for specific tasks, fostering innovation.
Summary of Findings
The exploration into circular polarization has revealed key insights:
- Definition and Characteristics: Circular polarization involves the rotation of the electric field vector in a helical pattern, offering distinct optical properties.
- Generation Techniques: Both natural and artificial methods exist to create circularly polarized light, with technologies like polarizers and wave plates being crucial.
- Measurement Techniques: Polarimetry and interferometry are effective ways to analyze circularly polarized light, aiding in various applications.
- Applications: The relevance of circular polarization spans diverse fields including optical systems, biological research, and quantum computing. Each application showcases its importance in advancing knowledge and technology.
Future Perspectives
The future of circular polarization research is promising. As technology continues to advance, new methodologies will likely emerge. Some future directions include:
- Emerging Technologies: Innovations in metamaterials and nanophotonics could lead to enhanced capabilities for manipulating light.
- Broadened Applications: Further investigation into chiral materials could reveal additional benefits in material science.
- Integration in Quantum Computing: Continued exploration of circular polarization could play a role in improving qubit representation and quantum communication frameworks.