Comparing TEM and SEM: Insights for Researchers
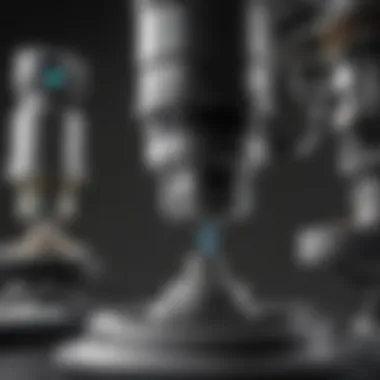
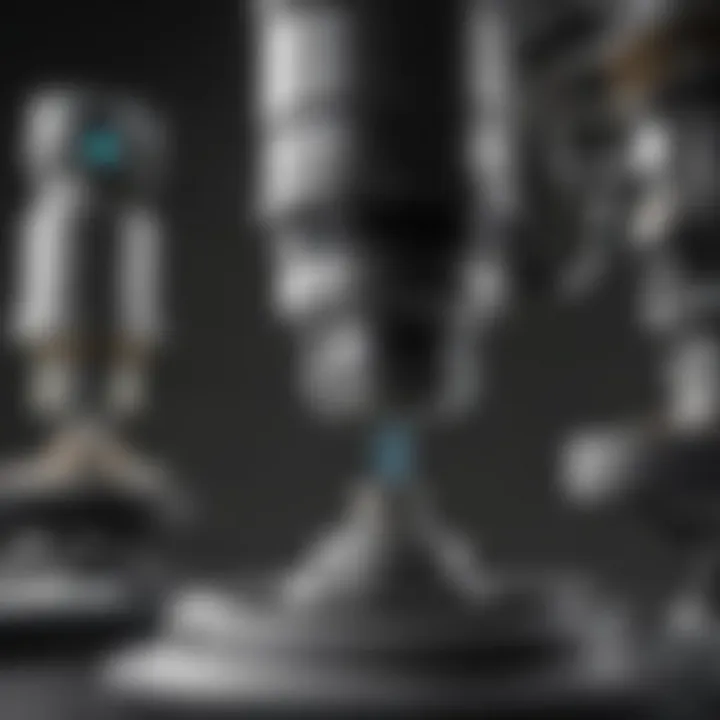
Intro
In the realm of advanced materials analysis, Transmission Electron Microscopy (TEM) and Scanning Electron Microscopy (SEM) serve as critical methodologies. Each technique offers unique insights into the structural, morphological, and compositional properties of materials at the micro and nanoscale. Understanding the nuances that distinguish TEM from SEM is fundamental for researchers, educators, students, and professionals aiming to harness these technologies for various applications.
Both microscopy techniques employ electron beams for imaging and analysis, yet they differ in their operational principles, sample requirements, and the type of information they provide. This article aims to clarify these differences and assist in the selection of the appropriate technique based on specific research objectives. It encompasses a thorough examination of each method, addressing key aspects such as image resolution, limitations, and suitable applications. The detailed exploration will illuminate both the capabilities and constraints intrinsic to each microscopy platform, ultimately guiding users in their investigative endeavors.
Overview of Microscopy Techniques
In the field of scientific research, microscopy techniques play a crucial role in providing insights at the microscopic level. These techniques allow researchers to observe structures and materials that are beyond the resolution of the naked eye. Understanding these microscopy techniques not only enhances our knowledge about materials but also aids in the development of new technologies and applications across various disciplines such as biology, materials science, and nanotechnology.
The Role of Microscopy in Scientific Research
Microscopy serves as a fundamental tool in many areas of scientific research. It enables scientists to explore the complexity of biological organisms, to analyze materials at the atomic level, and to investigate the properties of substances. The microscopic observation can reveal information about surface structures, composition, and physical properties. Such understanding is essential for advancements in fields such as material development and medical diagnostics.
Moreover, microscopy allows for the visualization of dynamic processes in real-time, facilitating a deeper understanding of phenomena like cell interactions and chemical reactions. Consequently, the ability to observe and analyze samples at high resolutions can lead to significant breakthroughs in science and engineering.
Classification of Microscopy Techniques
Microscopy techniques can be broadly classified based on various criteria, including the type of radiation used, the imaging technique, and the nature of the specimen. Some key classifications are:
- Optical Microscopy: Utilizes visible light to illuminate samples. Common types include bright field and fluorescence microscopy.
- Electron Microscopy: Uses electron beams to achieve much greater resolution than optical methods. There are two primary types: Transmission Electron Microscopy (TEM) and Scanning Electron Microscopy (SEM).
- Scanning Probe Microscopy: Involves scanning the surface of the sample with a probe to gather information on surface topography and properties.
Understanding these classifications is important for selecting the right technique for specific research objectives. Each method has unique advantages and limitations that influence its applicability to different types of samples and observations.
Foreword to Transmission Electron Microscopy (TEM)
Transmission Electron Microscopy (TEM) holds a significant position in the field of materials science and biological research. Its ability to provide high-resolution images at a nanometer scale allows for an in-depth understanding of sample structures. This article section elaborates on TEM's fundamental principles, its practical use, and its broader implications in scientific research. By grasping these aspects, one can fully appreciate TEM's value in both academic and applied contexts.
Fundamental Principles of TEM
At the core of TEM is the principle of transmitting electrons through an ultra-thin sample. An electron beam is generated using an electron source, typically a tungsten filament or a field emission gun. Once emitted, the electrons are focused into a beam that illuminates the sample. The electrons interact with the material, resulting in a combination of transmitted and scattered electrons which ultimately form an image on a phosphorescent screen or a digital detector. This technique can achieve atomic resolution, allowing scientists to visualize not just the arrangement of atoms but also defects and interfaces at a nanoscale.
Image Formation in TEM
Image formation in TEM involves several complex interactions. As the electron beam penetrates the sample, it may undergo various processes - transmission, diffraction, and scattering. The transmitted electrons contribute to the image, while diffracted electrons provide information about the material's crystalline structure. This results in contrast variations that indicate different densities or atomic positions. Through careful adjustments of the electron optics, specific features of the sample can be emphasized or suppressed. Therefore, the resulting images contain a wealth of information about the structural attributes and composition of the specimen.
Sample Requirements for TEM
Sample preparation for TEM is critical; it requires samples to be exceptionally thin, typically around 100 nanometers or less. This thinness is essential because electrons need to pass through the sample for effective imaging. Additionally, samples must be conductive or coated with a conductive layer if they are insulating, to avoid charging effects during imaging. The preparation process often involves grinding, polishing, and ion milling to achieve the desired thickness while maintaining the integrity of the sample for accurate analysis.
Common Applications of TEM
TEM is widely used across various disciplines. In materials science, it helps to elucidate the microstructure of alloys, ceramics, and nanomaterials. In biology, TEM is instrumental in studying cellular organelles and the morphology of viruses. Furthermore, researchers utilize TEM to analyze defects in semiconductors and the properties of polymers. The potential applications are vast, making TEM an indispensable tool for modern scientific investigation.
Advantages and Limitations of TEM
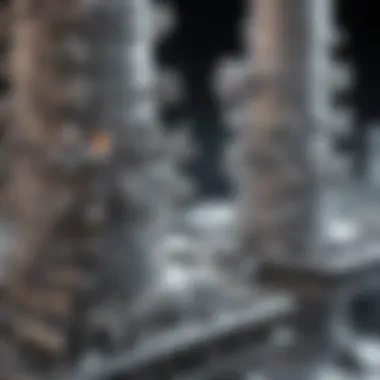
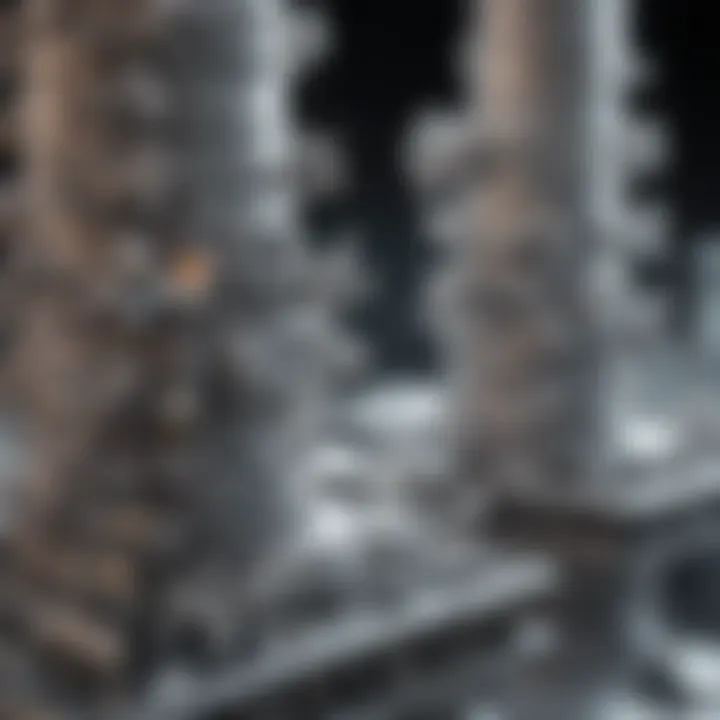
Advantages of TEM:
- High spatial resolution provides detailed images of nanostructures.
- Ability to perform electron diffraction and spectroscopy for compositional analysis.
Limitations of TEM:
- Sample preparation can be labor-intensive and time-consuming.
- The need for thin samples may not be suitable for all materials.
- It requires a vacuum environment, limiting the type of samples that can be analyzed.
TEM is a remarkable microscopy technique that offers profound insights into materials at an atomic level. Its strengths and limitations dictate its use in specific research scenarios.
Prolusion to Scanning Electron Microscopy (SEM)
Scanning Electron Microscopy (SEM) serves as an invaluable tool in the fields of materials science, biology, and nanotechnology. Its ability to render detailed images of surfaces through electron interactions marks it as a critical instrument in the analytical lab. Understanding SEM is essential for researchers and professionals who seek to uncover insights that traditional optical microscopy cannot offer. The technology's significance lies not only in its high-resolution imaging capabilities but also in its versatility across different domains.
When exploring materials, the way an object interacts with electrons under a vacuum significantly influences the types of data obtained. SEM provides surface morphology and composition information, essential for material analysis. As such, its alignment with various scientific disciplines further emphasizes the relevance of mastering this methodology for various applications.
Fundamental Principles of SEM
The core principle of SEM relies on scanning a focused beam of electrons across a sample surface. When this electron beam interacts with the atoms of the sample, it generates signals that can reveal various information about the surface topography and composition. The emitted secondary electrons are collected to form an image. This process delineates features with high precision down to the nanometer scale.
Critical aspects of this technique include:
- Electron Source: SEM typically uses a tungsten filament or a field emission gun to generate electrons.
- Vacuum Environment: A high vacuum is essential in SEM to prevent electron scattering by air molecules.
- Detectors: Various detectors capture secondary and backscattered electrons, facilitating a wide range of imaging modalities.
Overall, SEM relies on these principles to assess the details of samples with significant accuracy.
Image Formation in SEM
Image formation in SEM materializes through a sequence of interactions following the primary electron beamโs impact on the sample. As the beam scans the surface, secondary and backscattered electrons are generated. The emissions are collected in a detector, converted into a signal, and processed to create the final image displayed on a screen. The intensity of the signal correlates with the number of electrons collected, thus translating surface features into grayscale variations, allowing for high-resolution imaging.
Two key factors influence the images:
- Depth of Field: SEM provides a significant depth of field, resulting in sharp images of samples with three-dimensional features.
- Resolution: The resolution of SEM images can reach below 1 nanometer, showcasing fine structural details.
Ultimately, the imaging process in SEM allows for precise characterization of materials at each step of analysis.
Sample Preparation for SEM
The fidelity of SEM images hinges on thorough sample preparation. Unlike TEM, the sample for SEM does not require thin sections. A few considerations for preparing samples include:
- Conductivity: Non-conductive samples need to be coated with a conductive layer, like gold or carbon, to prevent charging under the electron beam.
- Stability: The sample must withstand the vacuum of the SEM chamber and the electron beam.
- Size: Samples should be appropriately sized to fit inside the chamber and ideally less than a few centimeters in dimension.
The success of SEM imaging relies heavily on the adherence to these preparation techniques.
Applications of SEM in Research
SEM plays a pivotal role in various fields, including:
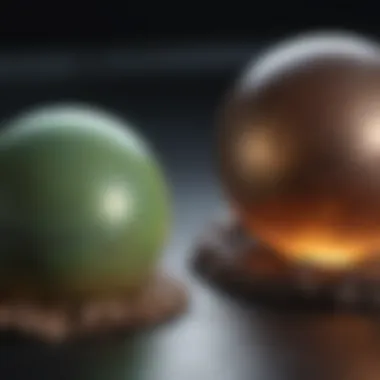
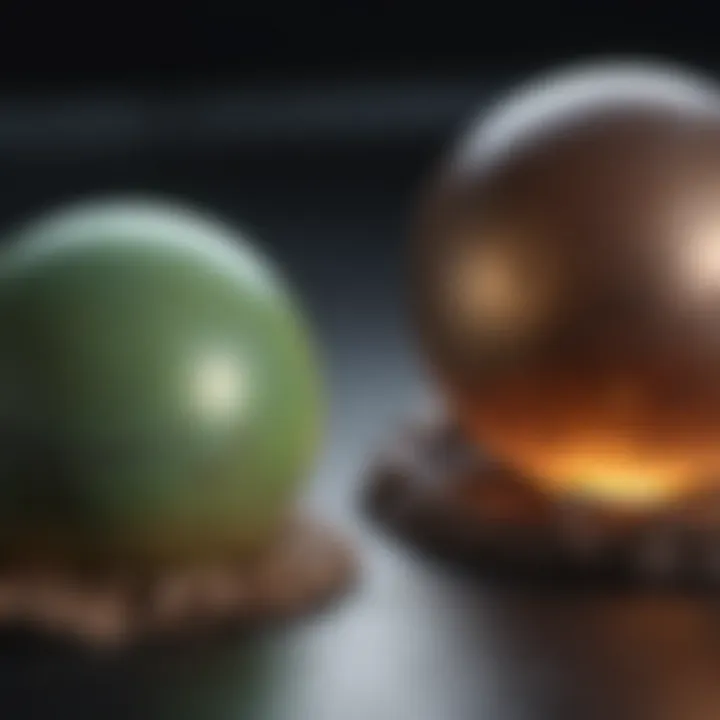
- Material Science: Analyzing the microstructure and surfaces of metals, ceramics, and polymers.
- Biology: Investigating cellular structures, tissues, and microorganisms at high resolution.
- Nanotechnology: Characterizing nanostructures and assembling new materials at the atomic level.
The versatility of SEM allows researchers to gather critical information across disciplines, supporting innovations in material design and research methodologies.
Advantages and Limitations of SEM
Advantages of SEM:
- High resolution and depth of field enhance image quality.
- Analysis in various environments (e.g., environmental SEM).
- Ability to perform elemental analysis via EDS (Energy Dispersive X-ray Spectroscopy).
Limitations of SEM:
- Requires vacuum conditions, which may not be suitable for all samples.
- Sample preparation can be time-consuming and challenging.
- Limited to surface analysis; internal structures are not directly visualized.
Understanding these advantages and limitations helps researchers effectively utilize SEM in their research endeavors.
Comparative Analysis of TEM and SEM
The comparative analysis of Transmission Electron Microscopy (TEM) and Scanning Electron Microscopy (SEM) serves as the foundation for understanding how these two techniques differ in both functionality and application. This section positions the reader to appreciate the critical insights gained from contrasting these methods. By delving into elements such as resolution, image quality, and cost, researchers can make informed choices tailored to their project needs. A comprehensive understanding fosters enhanced decision-making in selecting the ideal microscopy technique for specific scientific inquiries.
Resolution and Image Quality
Resolution plays a pivotal role in determining the image quality of microscopy techniques. In the case of TEM, it offers exceptional resolution, reaching down to the atomic scale. This high level of detail is achieved due to the wavelength of the electrons used, which is significantly shorter than that of visible light. Consequently, TEM facilitates intricate imaging of materials at a nano-scale level, making it invaluable in fields like material science and nanotechnology.
On the other hand, SEM provides lower resolution than TEM, yet it excels in surface topography imaging. The images produced by SEM are three-dimensional, giving a holistic view of the sample's structure. While SEM can achieve resolutions in the order of nanometers, the depth of field in SEM images is one of its standout features, allowing researchers to capture the surface features of samples effectively. Therefore, the choice between these two techniques will largely depend on whether the research priorities lie with magnifying surface details or probing the internal structure of materials.
Three-Dimensional Imaging Capabilities
The capability of creating three-dimensional images is one of the distinguishing features of SEM. Through its scanning technique, SEM recreates the sample surface in fine detail, providing much-needed perspective for researchers studying complex structures. This 3D representation is vital in a variety of applications, including biology, materials science, and semiconductor research, where understanding surface morphology is essential.
In contrast, while TEM is fundamentally a two-dimensional imaging technique, it also allows for some 3D segmentation through advanced analytical techniques. However, it does not inherently provide the same level of visualization of surface features as SEM. Researchers interested in three-dimensional characterization of materials often find SEM to be a more suitable option, particularly when dealing with layered structures or composite materials.
Cost and Accessibility of Techniques
Cost is a critical factor when selecting either TEM or SEM. Generally, TEM instruments are more expensive to procure and maintain compared to SEM systems. This is primarily due to the complexity and requirements of TEM, including the necessity for advanced sample preparation and the operating environment, which often requires higher vacuum levels. Moreover, the operational costs associated with TEM tend to be higher because of the specialized knowledge needed to operate the machinery and analyze the data.
In contrast, SEM systems are more prevalent in laboratories due to their relative affordability and easier operation. The access to SEM instruments is typically broader, making them a popular choice for educational settings as well as industrial applications. Researchers might choose SEM for routine investigations, while reserving TEM for projects that demand high-resolution insights into sample structures.
"Understanding the comparative characteristics of TEM and SEM is essential for researchers. The selection of the appropriate technique is dependent on the specific needs of each project."
In summary, the comparative analysis of TEM and SEM sheds light on their distinct functionalities, resolution capabilities, cost implications, and their respective roles in scientific research. A clear understanding of these factors ensures researchers can strategically choose the method that best aligns with their validation efforts.
Selecting Between TEM and SEM
Making the right choice between Transmission Electron Microscopy (TEM) and Scanning Electron Microscopy (SEM) is crucial for achieving desired outcomes in research and analysis. Each technique offers unique advantages and comes with its own set of constraints. Understanding these differences helps researchers tailor their approach based on specific scientific questions and material properties.
When selecting between TEM and SEM, several elements influence the decision-making process:
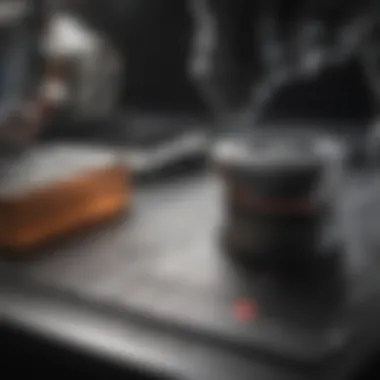
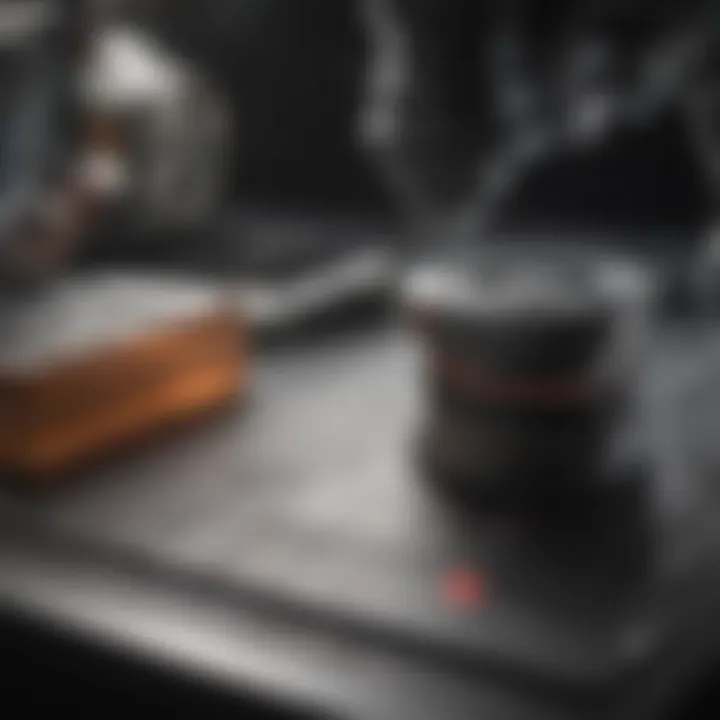
Factors Influencing Technique Selection
- Resolution Requirements: TEM typically provides higher resolution than SEM. If the investigation needs atomic-level detail, TEM is usually preferable. In contrast, SEM may suffice for lower resolution needs, such as surface morphology.
- Sample Thickness: TEM requires very thin samples, often around 100 nanometers or less. If a sample cannot be thinned adequately, SEM may be the better choice since it can analyze bulk specimens.
- Surface vs. Internal Structure: Depending on the focus of the study, the choice may differ. SEM excels at imaging surface topography and morphology. Contrarily, if internal structures or atomic arrangements are the primary concern, TEM is more suitable.
- Material Type: Some materials might be more compatible with one technique over another. For example, biological specimens often require preservation strategies in TEM that may not be necessary in SEM.
- Cost and Access: TEM instruments are generally more expensive to operate and maintain than SEM systems. Researchers must consider their budget and the accessibility of these tools in their institutions.
Case Studies: When to Choose TEM or SEM
To illustrate the decision-making process, consider the following case studies:
- Case Study 1: Nanomaterials Research
A researcher aiming to analyze the crystal structure of a new nanomaterial finds that they need atomic-level images for characterization. TEM provides the necessary resolution and depth of information, making it the clear choice. - Case Study 2: Surface Coatings Analysis
Another team examines the surface properties of a coated material. They require detailed images of the surface topography and compositional analysis. SEM is fitting here due to its strengths in imaging and the sampleโs robust nature, allowing for easy analysis without extensive preparation. - Case Study 3: Biological Sample Study
In a study involving cellular structures, researchers may prefer TEM as it facilitates imaging of internal organelles. However, if the focus shifts to understanding cell surface interactions, SEM becomes more applicable due to its non-invasive imaging capabilities.
Choosing between TEM and SEM remains a critical step in microscopy. Each case requires careful consideration of the specific research objectives, material characteristics, and practicalities surrounding the available resources.
Future Trends in Electron Microscopy
The field of electron microscopy is evolving rapidly due to technological advancements and emerging applications. Understanding these future trends is crucial for researchers and professionals who aim to apply these techniques effectively in various scientific domains. This section examines the primary advancements and potential applications that will shape the landscape of electron microscopy in coming years.
Advancements in Microscopy Technology
Recent developments in imaging technology have significantly enhanced the capabilities of electron microscopy. Innovations such as high-resolution detectors and chromatic aberration correction have improved image clarity and resolution, enabling researchers to view samples at an unprecedented level of detail. Moreover, the integration of machine learning and artificial intelligence in image processing can streamline data analysis, making it more efficient and accurate. This not only saves time but also reduces the chances of human error during interpretation of complex data sets.
Futhermore, electron tomography, a technique allowing three-dimensional visualization, is also being refined. This technique can reveal the fine structural details of materials in three dimensions, enhancing our understanding of structural hierarchies at the nanoscale. As these technologies continue to develop, electron microscopy will likely be essential in materials science and nanotechnology research, offering insights into previously unobservable structures.
Potential Applications of Emerging Techniques
The advancements in electron microscopy technology lead to several promising applications across various scientific fields. In materials science, for instance, enhanced electron microscopy can greatly assist in developing new alloys and composite materials with superior properties. By observing the behavior of materials on the atomic level, scientists can tailor properties to meet specific performance criteria, thus facilitating innovation in industries such as aerospace and electronics.
In biological research, emerging techniques such as cryo-electron microscopy can revolutionize structural biology by allowing for the observation of biomolecules in their near-native state. This can yield insights into protein structures that are crucial for understanding biological processes and can aid in drug discovery.
"As we harness the capabilities of new technologies in electron microscopy, we will unlock new frontiers in research opportunities that were previously thought impossible."
The potential for electron microscopy in environmental science is also significant. Innovations may allow for tracking pollution at the nanoscale, contributing to our understanding of environmental impacts and addressing global challenges.
In summary, the future trends in electron microscopy are poised to deepen our understanding of materials and biological systems. As advancements in technology pave the way for new applications, research will continue to benefit from the detailed insights provided by electron microscopy. This will ensure its critical role in science and engineering disciplines.
Ending
The conclusion of this article serves a crucial role in synthesizing the information explored throughout. By summarizing the significant points made regarding Transmission Electron Microscopy (TEM) and Scanning Electron Microscopy (SEM), it offers clarity on the distinctions between the two methods. This section aids in reinforcing the reader's comprehension of both techniques.
Summary of Key Points
To conclude the exploration of TEM and SEM, the following key points stand out:
- Principle Differences: TEM utilizes transmitted electrons for imaging, offering high resolution, while SEM employs reflected electrons, yielding a detailed 3D surface topology.
- Sample Preparation: TEM requires ultra-thin samples, often necessitating complex preprocessing, whereas SEM allows for bulk samples, with varying preparation techniques.
- Applications: TEM is often preferred for crystallography and nanomaterials, while SEM excels in surface studies and larger samples.
- Advantages and Limitations: Each method has unique strengths; TEM provides superior resolution, while SEM is more user-friendly and accessible.
By consolidating these aspects, researchers can better appreciate the situational prowess of each microscopy technique.
The Importance of Choosing the Right Technique
The selection between TEM and SEM is not a decision to be taken lightly. Understanding the specific needs of a research project is fundamental.
- Research Goals: Recognizing whether high-resolution imaging or detailed surface information is paramount can guide the selection process.
- Budget Constraints: Each technique involves different operational costs and maintenance; understanding financial implications is essential.
- Sample Nature: The characteristics of the samples being analyzed dictate preparation methodologies and imaging outcomes.
In sum, this conclusion highlights the importance of informed choices in electron microscopy. Understanding how TEM and SEM differ guides researchers toward selecting the suitable technique. This decision directly impacts the quality of the data obtained and the advancement of scientific inquiry.