Understanding Dihydroxyacetone Phosphate in Metabolism
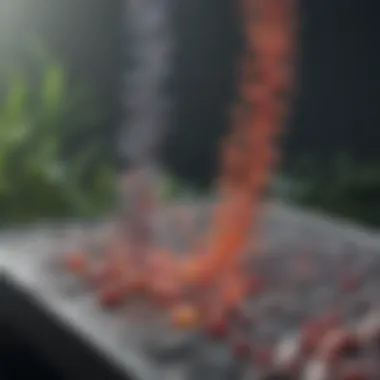
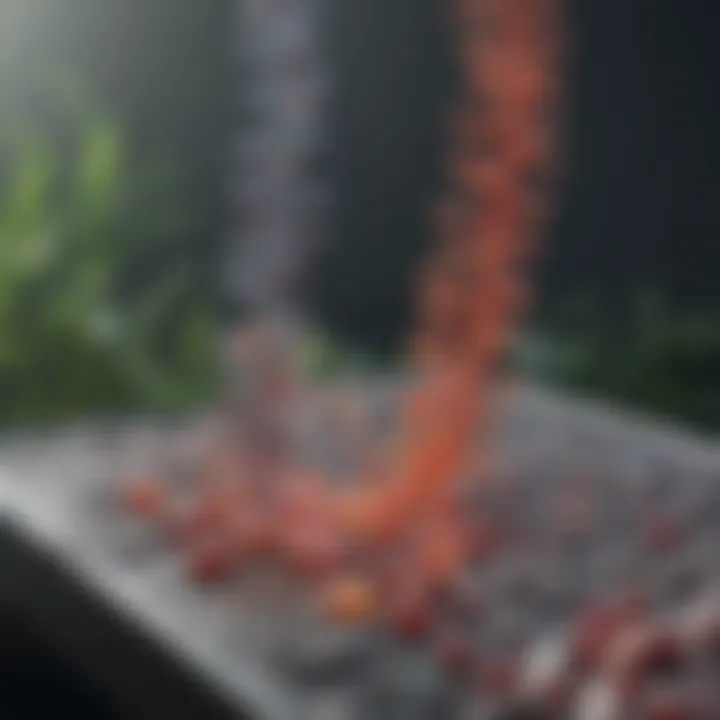
Intro
In the intricate world of cellular biochemistry, certain molecules serve as pivotal intermediates, guiding various metabolic pathways. Among them, dihydroxyacetone phosphate, often abbreviated as DHAP, stands out due to its dual role in both glycolysis and gluconeogenesis. This compound plays crucial parts in energy production and regulation, making it essential to understanding metabolic processes.
Why focus on DHAP? This seemingly simple three-carbon molecule weaves through numerous pathways in cells. It acts not only as a substrate but also as a regulatory factor, influencing the activity of several enzymes. Recognizing its role illuminates not just basic metabolic functions but also potential clinical implications in metabolic disorders such as diabetes or obesity. By dissecting the characteristics of DHAP, its reactions, and the consequences of its altered concentrations, we can deepen our comprehension of both normal physiology and pathological states.
In the sections that follow, we will examine the research pertaining to DHAP, unveiling its importance while providing insights into methodologies employed in current studies. Anticipating how DHAP fits within the larger puzzle of cellular metabolism helps in crafting a clearer picture of its significance.
Preface to Dihydroxyacetone Phosphate
Dihydroxyacetone phosphate, more commonly known as DHAP, is a pivotal component in the realm of cellular metabolism. This organic molecule is an intermediary in crucial metabolic pathways, primarily glycolysis and gluconeogenesis, and plays a significant role in energy production and the synthesis of carbohydrates. Understanding DHAP is not merely a matter of academic interest; it holds substantial implications for both physiology and clinical research.
From a foundational standpoint, the study of DHAP offers insights into how energy is harvested and utilized within cells. It effectively bridges pathways that transform glucose into energy, making it a topic of interest for those involved in biochemistry, nutrition, and health sciences. Additionally, the concentrations and activity of DHAP can provide indicators of metabolic health, which leads us to consider its clinical relevance.
Also worth noting is how the historical understanding of DHAP has evolved over time. Learning about its discovery and characterization can lend perspective to its current applications in biotechnology and metabolic engineering. In essence, the introduction to DHAP sets the stage for a deeper dive into its stunning biochemical role and the regulatory mechanisms underlying its functionality.
Definition and Chemical Structure
Dihydroxyacetone phosphate (C3H7O6P) is a three-carbon sugar phosphate. Structurally, it features a phosphate group attached to a glycerol backbone with two hydroxyl groups. This configuration enables DHAP to be part of various biochemical reactions, especially in its transformation to glyceraldehyde 3-phosphate when involved in glycolysis. Understanding its chemical structure is critical, as it determines the properties and reactivity of the molecule within different metabolic contexts.
The molecular formula showcases its complexity while also highlighting its essentiality. By examining the arrangement of its atoms, researchers can uncover how DHAP functions within cellular pathways. The phosphate group, for instance, establishes DHAP's role in energy transfer, acting as a source of chemical energy in biochemical transformations.
Historical Perspective
The history of dihydroxyacetone phosphate stretches back to early biochemical research in the mid-20th century. Initial isolations and characterizations often occurred alongside the foundational discoveries of glycolysis. Early biochemists like Embden and Meyerhof, who were instrumental in decoding the glycolytic pathway, hinted at the importance of intermediate compounds like DHAP.
As research progressed, scientists began to appreciate DHAPâs saturation in human metabolism, recognizing it not just as a single entity but as part of several interlinked pathways. Modern techniques, including mass spectrometry and nuclear magnetic resonance (NMR), have further unveiled the complex nature of DHAP, allowing for more nuanced studies of its metabolic roles and regulatory mechanisms.
This historical context underscores the evolution of knowledge surrounding DHAP and frames its relevance today. With a better understanding of its functions, researchers can address contemporary issues in metabolic disorders, thus paving the path for potential therapeutic innovations.
Biochemical Role of Dihydroxyacetone Phosphate
Dihydroxyacetone phosphate (DHAP) plays a pivotal role in various biochemical reactions within the body. Understanding this compound's biochemical significance is critical, as it serves as a key intermediary in both the energy-generating and biosynthetic pathways. By examining its contributions, we appreciate how DHAP plays a balancing act between catabolic and anabolic processes, impacting overall metabolism.
Metabolic Pathways Involving DHAP
Glycolysis
Glycolysis is the classic path of glucose breakdown, generating energy in the form of ATP. DHAP is formed from fructose 1,6-bisphosphate during the glycolytic phase, which adds to its essential role in energy production. The formation of DHAP represents a central link in carbohydrate metabolism. Its conversion to glyceraldehyde 3-phosphate allows for the continued flow of glycolysis. This pathway is universally recognized for its efficiency in rapidly generating energy, thus making it a popular point of study in cellular bioenergetics.
⢠Key Characteristic: Glycolysis is anaerobic, allowing energy production without the need for oxygen.
⢠Benefits: Quick ATP generation, especially under low oxygen conditions.
⢠Unique Feature: This pathway produces not just energy but also intermediates important for other metabolic pathwaysâessentially a metabolic multitasker.
However, glycolysis can lead to excessive lactate formation in anaerobic conditions, causing metabolic acidosis.
Gluconeogenesis
Gluconeogenesis, in stark contrast, is the synthesis of glucose from non-carbohydrate substrates. Here, DHAP acts as a fundamental precursor, demonstrating its versatility in metabolism. This process becomes particularly significant during fasting or intense exercise when glucose levels plummet. By converting DHAPâalongside other compoundsâinto glucose, the body maintains homeostasis.
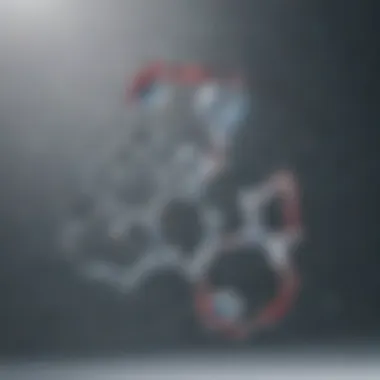
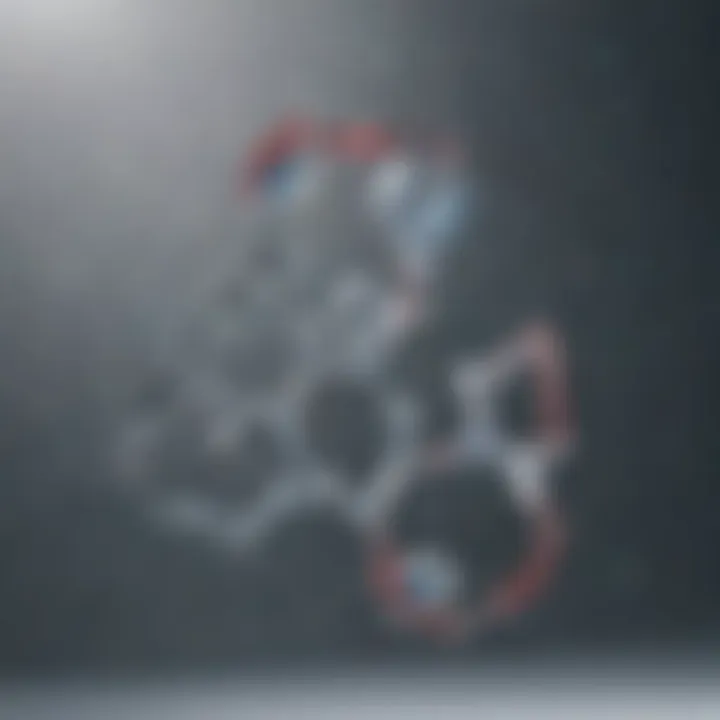
⢠Key Characteristic: Gluconeogenesis predominates in the liver and kidneys.
⢠Benefits: Essential during periods of starvation or hypoglycemia.
⢠Unique Feature: It allows the body to combat low blood sugar by creating glucose de novo.
While crucial, gluconeogenesis is energetically costly since it requires ATP and GTP, which can challenge the balance in low-energy states.
Pentose Phosphate Pathway
The Pentose Phosphate Pathway (PPP) is another significant route where DHAP comes into play, particularly in cellular anabolic processes. This pathway is not directly linked to ATP production. Instead, it generates NADPH and ribose-5-phosphate, essential for fatty acid synthesis and nucleotide production. DHAP can be interconverted to glyceraldehyde-3-phosphate, which feeds into the PPP, thus linking energy production with biosynthesis.
⢠Key Characteristic: Provides reducing power in the form of NADPH.
⢠Benefits: Critical for biosynthesis and cellular protection against oxidative stress.
⢠Unique Feature: This pathway is crucial for cellular growth and division due to ribose-5-phosphate availability for nucleotide synthesis.
Yet, itâs noteworthy that disregarding the energy production aspects can create imbalances if not regulated, leading to excessive metabolite production and potential disruptions in cellular functions.
Intermediary Metabolism
Intermediary metabolism provides a comprehensive view of how DHAP interacts with various biochemical pathways beyond energy production. It supports the intertwining of metabolic routes, facilitating the necessary adjustments in response to cellular needs.
DHAP's involvement in various pathways showcases its flexibility and importance to overall metabolic health. By effectively bridging the gap between energy production and biosynthesis, it illustrates how interconnected reactions are for maintaining homeostasis within the organism. Understanding those connections is essential for exploiting therapeutic targets in metabolic disorders.
Regulatory Mechanisms of Dihydroxyacetone Phosphate
Understanding the regulatory mechanisms of dihydroxyacetone phosphate (DHAP) is integral to grasping its complex role in cellular metabolism. This section delves into the various layers of control that ensure the precise concentration of DHAP within the cell, highlighting the benefits these regulatory mechanisms offer in maintaining metabolic homeostasis.
Enzymatic Regulation
Enzymatic regulation of DHAP primarily involves key enzymes that catalyze its formation and conversion into other metabolites. One such enzyme, aldolase, facilitates the reversible reaction between fructose 1,6-bisphosphate and DHAP in the glycolytic pathway. Another important player is triose phosphate isomerase, which converts dihydroxyacetone phosphate into glyceraldehyde 3-phosphate. The thought process here is similar to a finely tuned orchestra, where each enzyme must play its part at just the right time to keep the metabolic process smooth and efficient.
The activity of these enzymes is affected by various factors such as substrate availability, product concentration, and even environmental conditions like pH and temperature. For instance, significant elevations in fructose 1,6-bisphosphate can lead to enhanced activity of aldolase, indirectly boosting DHAP levels. This autoregulatory mechanism ensures that DHAP is available when needed but is also limited during periods of excess.
Moreover, enzyme inhibitors or activators can influence the activity of key enzymes, sometimes in surprising ways. For example, certain metabolites can bind to an enzyme and alter its shape and function, thus changing the rate of DHAP production without directly altering its concentration.
Allosteric Influences
Allosteric regulation refers to how molecules can bind to enzymes at sites other than the active site, thereby inducing a conformational change that alters enzymatic activity. In the case of DHAP, several allosteric regulators have been identified.
For instance, ATP, which signals a high energy state in the cell, can enhance the activity of enzymes like phosphofructokinase, indirectly influencing the flow of metabolites through glycolysis and ultimately affecting DHAP levels. Conversely, accumulation of AMP indicates a depleted energy state, which may shift the metabolic pathway's focus away from synthesis, thereby reducing DHAP levels when energy is scarce.
Hormonal Regulation
Hormonal mechanisms also play a pivotal role in regulating DHAP levels within the cell. Insulin and glucagon are two hormones that exert contrasting effects on cellular metabolism. When insulin is released, it promotes pathways that favor glycogenesis and glycolysis, enhancing glucose uptake, which can lead to increased DHAP through the glycolytic pathway. This regulation aligns with the bodyâs need to store energy after meals.
On the flip side, glucagon is secreted during fasting or low-energy states, triggering gluconeogenesis and lipolysis - processes that might lead to lower DHAP levels as nutrients are mobilized to generate glucose from non-carbohydrate sources. This dance of hormones ensures that DHAP remains in balance, much like a well-rehearsed ballet, adapting to the metabolic demands of the body.
"Understanding the regulatory mechanisms of DHAP sheds light on its multifaceted role in maintaining metabolic health."
Structural Properties of Dihydroxyacetone Phosphate
Understanding the structural properties of dihydroxyacetone phosphate (DHAP) is fundamental in grasping its roles within metabolic pathways. The molecular characteristics of DHAP not only dictate its function but also its interactions with enzymes and other cellular components. In this section, we will delve into the molecular configuration of DHAP, followed by an examination of isomerization and its subsequent impacts on metabolic processes.
Molecular Configuration
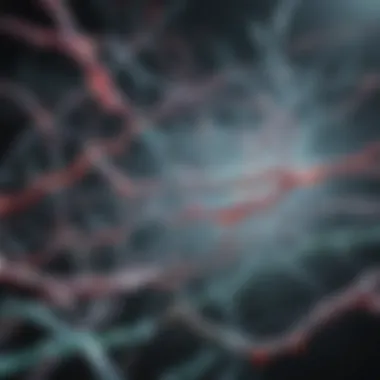
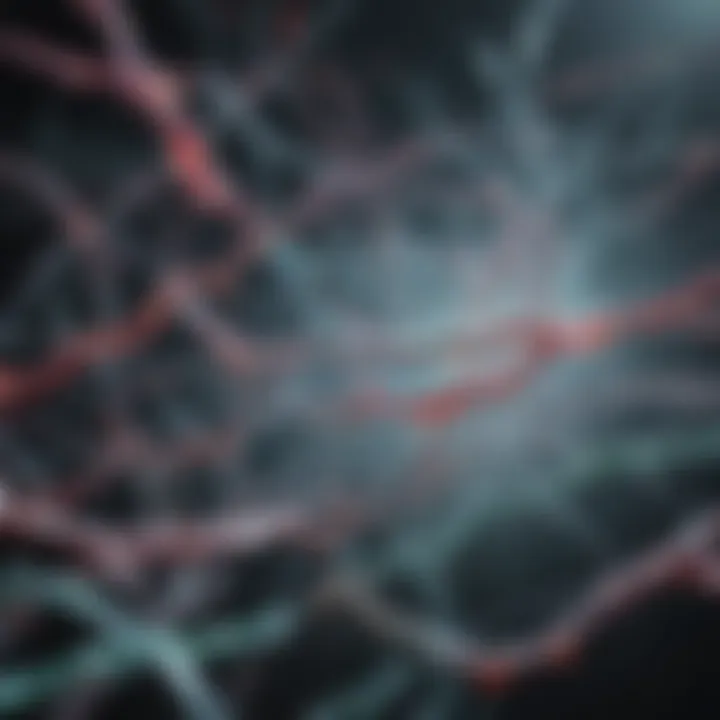
Dihydroxyacetone phosphate is a triose phosphate, which means it possesses three carbon atoms. Its chemical formula is CâHâOâP. This composition includes two hydroxyl groups (-OH) at specific carbon atoms and a phosphate group (-POâ) attached to the third carbon. The arrangement of these functional groups is critical as it influences both the charge and polarity of the molecule, thereby affecting its solubility in aqueous environments.
From a stereochemical perspective, DHAP exists in an equilibrium between its open-chain form and a cyclic structure. Importantly, the phosphate group introduces a negative charge, making DHAP highly reactive. This behavior is crucial during various enzymatic reactions. In a biological context, the exact configuration of DHAP allows it to serve as a significant precursor in glycolysis and gluconeogenesis.
Some key points regarding the molecular structure include:
- Functional Groups: The presence of hydroxyl and phosphate groups facilitates interaction with enzymes such as aldolase.
- Polarity and Reactivity: The charged nature promotes solubility and reactivity, essential for participation in metabolic pathways.
- Stereochemistry: DHAP can convert to glyceraldehyde 3-phosphate, highlighting its role in the overall metabolic network.
Isomerization and Its Effects
Isomerization refers to the process where a molecule transforms into an isomer, a different structural form that has the same molecular formula. In the case of dihydroxyacetone phosphate, it can isomerize into glyceraldehyde 3-phosphate. This transformation is catalyzed by the enzyme triose phosphate isomerase and it plays a critical role in the flow of metabolic reactions, particularly those linked to glycolysis and gluconeogenesis.
The significance of this isomerization includes:
- Metabolic Flexibility: The ability to switch between DHAP and glyceraldehyde 3-phosphate provides the cell with flexibility in energy production.
- Regulatory Role: Isomerization can regulate the availability of substrates for downstream metabolic processes, impacting energy yields and biosynthetic pathways.
- Dynamic Equilibrium: The reversible nature of this transformation means that under specific cellular conditions, one form can be favored over the other, affecting overall metabolism.
"The ability to interconvert between different molecular forms is a cornerstone of metabolic adaptability, allowing cells to navigate changing energy demands."
In summary, the structural properties of dihydroxyacetone phosphate, including its molecular configuration and the implications of isomerization, underscore its vital role in cellular metabolism. These characteristics not only enable DHAP to participate actively in key metabolic pathways but also highlight its dynamic nature, ensuring that cellular functions remain efficient and responsive to physiological demands.
Dihydroxyacetone Phosphate in Cellular Processes
Dihydroxyacetone phosphate, often casually referred to as DHAP, plays a pivotal role in the cellular processes of living organisms. Its significance cannot be overstated as it serves not just as an intermediary metabolite but as a linchpin connecting various biochemical pathways.
The understanding of DHAP goes beyond the rewiring of glucose. Emphasizing its cellular functions sheds light on how energy production, lipid metabolism, and even amino acid synthesis are intertwined with this molecule. In light of metabolic health, exploring these functions is vital for students, researchers, educators, and professionals who are versed in biochemistry and biology.
Role in Energy Production
DHAP is fundamentally linked to the energy currency of the cell. It is an integral part of glycolysis, the pathway through which glucose is broken down to generate ATP. Without DHAP, glycolysis would hit a speed bump, creating a bottleneck in energy extraction.
Consider this:
- When glucose is converted to DHAP, it represents a critical junctureâa fork in the metabolic road.
- DHAP can be readily converted into glyceraldehyde 3-phosphate, which directly feeds into the glycolytic pathway.
- The energetic potential of glucose metabolism hinges on maintaining an equilibrium that allows for smooth transitions between these intermediates.
Thus, one can argue that the role of DHAP in energy production highlights its importance in cellular respiration and overall metabolism.
Impact on Lipid Metabolism
Besides energy, DHAP has significant implications in lipid metabolism. It acts as a substrate for the synthesis of glycerol, a vital component of triglycerides. In simpler terms, this means that without DHAP, the body's ability to store fat in adipose tissues would be compromised.
The process can be condensed into a few points:
- DHAP contributes to the formation of backbone structures in lipids.
- The availability of DHAP influences not only energy storage but also insulation and cellular signaling.
- An imbalance, where DHAP is either too low or too high, could lead to metabolic disorders, affecting how the body manages fats.
Involvement in Amino Acid Metabolism
Finally, one cannot overlook the role of DHAP in amino acid metabolism. It plays an indirect but crucial role in synthesizing certain amino acids, thereby injecting itself into the very fabric of protein metabolism.
In this context, the implications of DHAP are manifold:
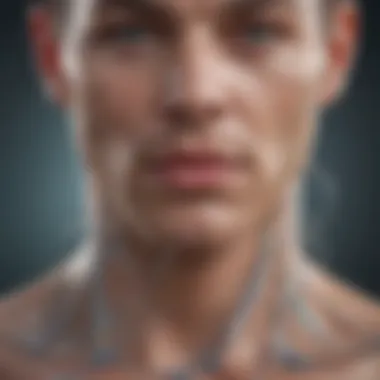
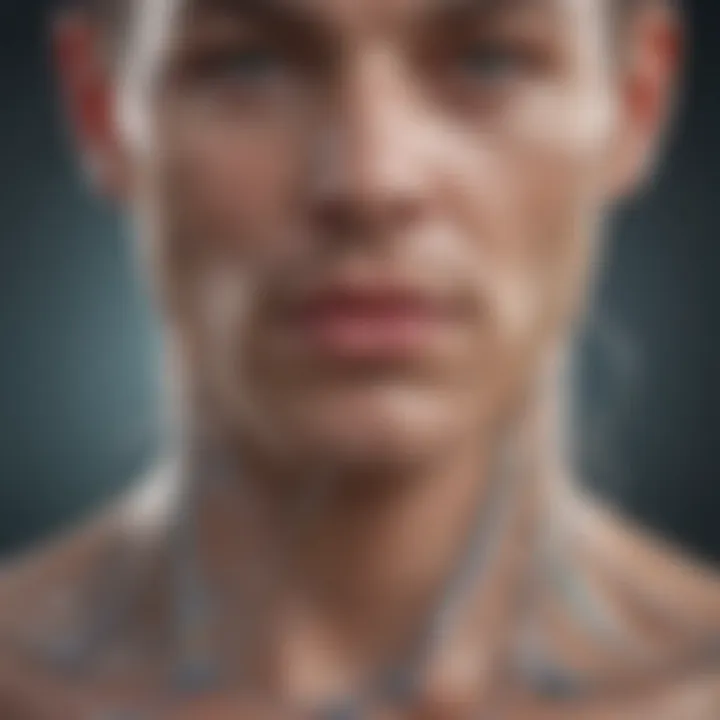
- It interfaces with various transamination and deamination reactions.
- It aids in providing carbon skeletons necessary for the synthesis of amino acids, forming a feedback loop in metabolic pathways.
Given the importance of proteins in countless physiological functions, the contribution of DHAP shows how interconnected these processes are. This interdependence places DHAP at the center of discussions pertaining to cellular health and metabolic balance.
"Dihydroxyacetone phosphate is more than a mere intermediate; it is a critical player in the grand narrative of cellular metabolism.â
In summary, the influence of Dihydroxyacetone phosphate on energy production, lipid metabolism, and amino acid synthesis underscores its comprehensive role in cellular processes. Without it, the metabolic orchestra would falter, demonstrating just how essential this compound is to life itself.
Clinical Relevance of Dihydroxyacetone Phosphate
Dihydroxyacetone phosphate (DHAP) extends beyond mere biochemical curiosity; its implications in clinical settings warrant significant attention. As an intermediary in critical metabolic pathways, understanding the role of DHAP can illuminate various metabolic disorders and catalyze the development of therapeutic strategies. This section will give an overview of its relevance, with particular emphasis on diseases like diabetes, obesity, and metabolic syndromeâconditions that often manifest in modern society. The discussion here is rooted in how alterations in DHAP concentrations can affect health and highlight potential benefits of targeting this molecule from a therapeutic standpoint.
Implications in Metabolic Disorders
Diabetes
Diabetes has garnered immense focus in recent years, and its relationship with DHAP is intriguing. To put it plainly, high glucose levels can lead to increased DHAP levels, especially given that it occurs at a critical junction in glycolysis and gluconeogenesis. This relationship becomes paramount in understanding how glucose homeostasis might be disrupted in diabetes. Some research suggests that excessive DHAP can be linked with diabetic complications due to the rise in end products of glycolysis.
A unique feature of diabetes is its multifaceted nature, encompassing both Type 1 and Type 2 forms, making it essential to consider how DHAP pathways differ across variants. Therein lies the real potentialâmodulating DHAP could provide a path for innovative therapeutic strategies aimed at restoring metabolic balance in diabetic patients.
Obesity
When examining obesity, one cannot overlook its complex interactions with cellular metabolism, including the metabolism of DHAP. Elevated DHAP levels sometimes signal increased lipid biosynthesis, a credit to its role in connecting glycolysis and lipid metabolism. This characteristic of obesity as a condition that can fuel metabolic imbalances makes it a compelling area for investigation.
The noteworthy aspect of obesity is its prevalence among various demographics and its links to other comorbidities. Pinpointing where DHAP fits into the obesity puzzle could shed light on weight management and offer paths to therapeutic interventions that target metabolic pathways to mitigate obesity-related health risks.
Metabolic Syndrome
Metabolic syndrome is a cluster of conditions that can include raised blood pressure, elevated blood sugar, excess body fat around the waist, and abnormal cholesterol levels. This condition is significant because it serves as a predisposition for cardiovascular diseases. It stands out as an excellent candidate for further exploration of DHAP's role, especially since changes in DHAP metabolism can reflect dysregulated energy homeostasis. In metabolic syndrome, an important characteristic is the insidious nature of the condition, often not diagnosed until significant health impacts arise. Understanding how DHAP may serve as a biomarker or therapeutic target could yield new insights into managing metabolic syndrome's progression or severity.
Potential Therapeutic Applications
Considering the interplay between DHAP and metabolic disorders, it logically follows that there lies therapeutic potential. Opportunities could be found in pharmacological approaches that modulate DHAP levels or its related metabolic pathways. Recent studies have indicated, for instance, that compounds aimed at regulating the action of enzymes associated with DHAP metabolism may pave the way for innovative treatments. Therapies focused on lowering DHAP levels could potentially ameliorate some of the adverse effects associated with diabetes or help in managing obesity. Given that the studies in this area are still unfolding, the horizon looks promising, and researchers are keen to explore how to harness this knowledge into tangible benefits for clinical practice.
The End
Dihydroxyacetone phosphate (DHAP) occupies a cornerstone position in the realm of cellular metabolism, making it imperative to underscore not only its structure and functions but also its broader implications. The interplay of various metabolic pathways that hinge on DHAP illustrates a complex orchestration essential for maintaining homeostasis within living organisms. Addressing the significance of this molecule illuminates its potential in understanding metabolic disorders, paving the way for novel therapeutic approaches.
The discussion in this article reveals that DHAP's role extends beyond mere participation in glycolysis and gluconeogenesis. In fact, its involvement in energy production, lipid metabolism, and amino acid synthesis showcases its adaptability and critical nature in biochemical processes. Here are some key takeaways from our exploration:
- Pathways and Interactions: DHAP interlinks several metabolic pathways, signifying how disruptions in its regulation can have cascading effects.
- Clinical Implications: Linking DHAP to conditions such as diabetes and obesity creates a narrative on how it could serve as a biomarker or a target for pharmacological interventions.
- Structural Attributes: Understanding DHAP's molecular configuration aids in discerning its behavior in various physiological contexts.
The importance of this conclusion lies not just in summarizing the findings, but also in inviting further inquiry into the multifaceted roles of DHAP, considering both established and emergent research. As we anticipate advancements in biochemistry, grasping the nuances of metabolite regulation like that of DHAP proves essential for future innovations in medical science.
Summary of Findings
Throughout this examination, several pivotal points emerged:
- Integral Role in Metabolism: DHAP is a key player in both glycolysis and gluconeogenesis, bridging the gap between catabolism and anabolism.
- Enzymatic Control: Various enzymes regulate DHAP's concentration and transformation, emphasizing the delicate balance that maintains metabolic health.
- Clinical Significance: The association of DHAP with metabolic disorders illustrates the relevance of this compound in health science, indicating possible directions for research into therapeutic interventions.
In addition, the mechanisms through which DHAP participates in lipid and amino acid metabolism underscore its versatility as a metabolite.
Future Directions in Research
Beneath the surface of current knowledge lies extensive potential for advancing our understanding of DHAP. Future research avenues could explore:
- Drug Development: Investigating how targeting DHAPâs pathways might foster novel therapeutic strategies for metabolic syndromes.
- Biomarkers for Disease: Evaluating DHAP levels in clinical settings to identify its efficacy as a biomarker for diagnosing metabolic disorders.
- Genomic and Proteomic Studies: Analyzing how genetic variations affect DHAP metabolism and its implications for personalized medicine.
There's a world of complexity wrapped in the structure and function of DHAP, and the journey does not end here. Unlocking the secrets of this molecule holds potential not only for biochemistry but also for improving human health across the globe.