Elastin Protein: Its Role in Health and Disease
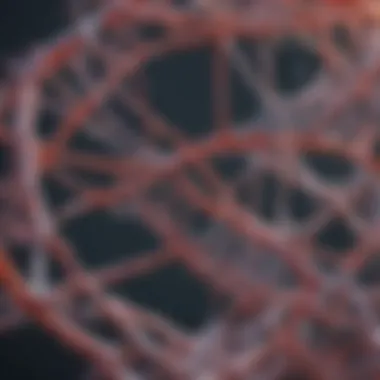
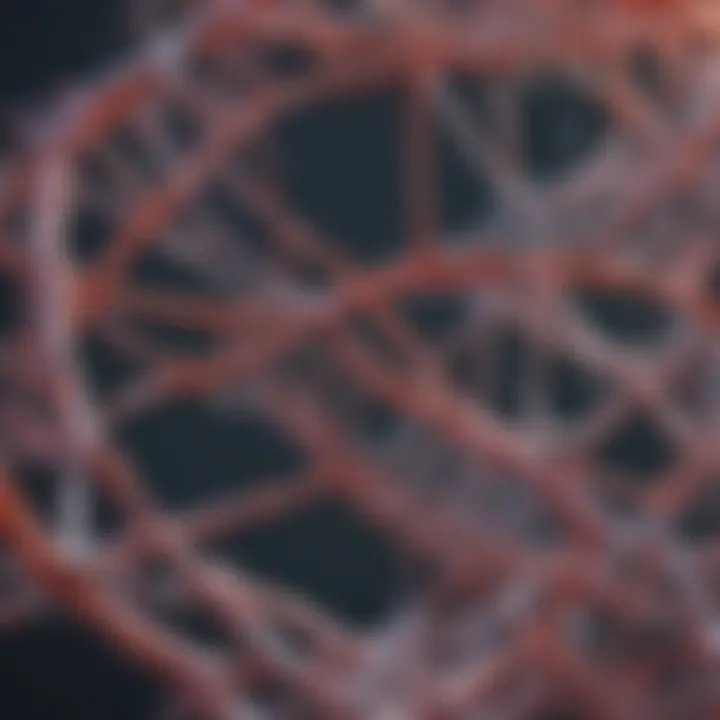
Intro
Elastin protein, a versatile component of our connective tissues, boasts the unique ability to stretch and recoil, akin to a rubber band. This remarkable property is crucial for maintaining the structural integrity of various tissues in the body. From skin and blood vessels to lungs and ligaments, elastin plays a fundamental role in providing elasticity and resilience. As we age, the production of elastin declines, leading to various health implications that warrant deeper examination.
Understanding elastin goes beyond just appreciation of its properties. The synthesis, organization, and degradation of this protein are deeply intertwined with numerous physiological processes. In this article, we will take a closer look at these mechanisms, how they are affected by aging, and the potentially significant medical conditions tied to elastin insufficiency.
Through this exploration, we aim to highlight not only the biological significance but also the cutting-edge research focused on replenishing elastin levels as a therapeutic strategy. The narrative will cover innovative approaches being studied, which could offer new avenues for treatment in dermatology and other fields.
By comprehensively dissecting these various aspects, the article aspires to deliver informative insights to students, researchers, educators, and professionals alike, bridging various scientific disciplines around this vital protein.
Research Overview
Summary of Key Findings
Elastin is predominantly found in connective tissues, where it works hand-in-hand with collagen to provide structure and elasticity. Recent findings suggest that the age-related decline in elastin and its synthesis correlates closely with several conditions, including skin aging, cardiovascular diseases, and pulmonary disorders. Here are some key takeaways:
- Structure and Function: Elastin is a fibrous protein composed primarily of amino acids like glycine, valine, and proline. Its unique cross-linking structure enables significant elasticity.
- Synthesis and Degradation: The fibroblasts are responsible for elastin production, while enzymes such as elastase play a role in its degradation.
- Impact of Aging: As people age, not only does elastin production slow down, but the existing fibers become fragmented, further reducing elasticity.
Importance of the Research
The examination of elastin protein is not only significant in the realm of basic biology but also presents critical implications for medical research. Understanding how elastin functions can aid in devising treatments for conditions associated with its loss, like osteoporosis, chronic obstructive pulmonary disease (COPD), and various skin disorders. Addressing elastin levels could also improve overall health and enhance the quality of life for aging populations.
One notable focus area is the development of therapies aimed at replenishing elastin through genetic engineering and synthetic biology. These innovative treatments hold promise and can transform the landscape of regenerative medicine.
Methodology
Study Design
The research encompasses a variety of interdisciplinary approaches, combining molecular biology, clinical studies, and translational research to provide a comprehensive picture of elastin's role in health and disease. For instance, in vitro studies of fibroblast cultures help elucidate the mechanisms of elastin synthesis and regulation.
Data Collection Techniques
Data collection is achieved through a combination of methods:
- Genetic Analysis: Investigating genetic expression levels of elastin in various tissues.
- Histological Examination: Utilizing microscopy to observe elastin architecture in tissue samples.
- Clinical Trials: Conducting trials on potential elastin therapies, assessing their effectiveness in increasing elastin levels or restoring tissue function.
By integrating these diverse methodologies, researchers can piece together a more holistic understanding of elastin's critical functions and its significance in medical conditions, paving the way for more targeted interventions.
Understanding Elastin Protein
Elastin protein is a fundamental player in the realm of structural biology, often overshadowed by its more famous counterpart, collagen. Yet, it’s elastin that provides tissues with the remarkable ability to stretch and recoil—think of it as the rubber band that helps hold our bodies together. Understanding elastin is crucial not just for recognizing its role in maintaining tissue integrity but also for grasping its implications in various medical conditions and aging processes.
Elastin is primarily found in areas that require flexibility, such as the skin, lungs, and blood vessels. This protein works tirelessly behind the scenes to ensure that our bodies can endure repeated stretching without losing shape or function. As we explore elastin further, it’s essential to consider its composition, biological significance, and the potential consequences when elastin levels wane or malfunction.
Definition and Overview
Elastin is a highly elastic protein that forms the main connective tissue matrix in vertebrates. Composed primarily of a unique combination of amino acids, elastin fibers help organs and tissues maintain their natural shape and assist in their functionality. Unlike other connective fibers, elastin has an inherent ability to retract after being stretched. This property plays a vital role in various physiological processes. For instance, in blood vessels, elastin allows the arteries to expand during blood flow and return to their original state when the pressure drops.
Understanding the specific characteristics of elastin comes with appreciating its resilience and functional makeup. It consists of elastin monomers, primarily aggregating to form cross-linked structures. These linkages increase the elasticity significantly compared to collagen fibers, which are more about providing tensile strength. Hence, elastin serves as a core element in soft tissues, allowing them to withstand dynamic mechanical stress.
Historical Context
The story of elastin dates back to the early 20th century when researchers started to distinguish this protein from collagen. In 1948, the scientist Earl W. Buehler contributed significantly to our understanding of elastin's structural properties through his pioneering studies. Prior to this, proteins were often studied in isolation, without much context about their functions in the wider biological tapestry.
Over the decades, elastin gained attention not only for its stretchiness but also for its potential connections to various diseases. By the late 20th century, scientists began to unravel how modifications in elastin structure or content could be tied to conditions such as cardiovascular diseases and skin disorders. The exploration of elastin has been a journey through science, showcasing not just the protein itself but its intricate connection to health, pathology, and aging over time.
Understanding elastin’s history sheds light on its significance in both research and clinical settings, as it highlights how far we've come in comprehending this crucial protein. The role of elastin extends beyond a structural support beam; it’s a focal point for advancing our knowledge regarding tissue engineering and regenerative medicine, driving further investigations into how we can modify its properties for therapeutic benefits.
The Structure of Elastin
The structure of elastin is a fundamental aspect that defines its unique functional properties in the body. Understanding this structure helps illuminate how elastin maintains tissue elasticity, a critical factor in providing resilience and flexibility across various systems. This segment delves into the intricate details that constitute elastin, providing insight on why it matters in health and disease.
Amino Acid Composition
Elastin's amino acid composition serves as the building block for its highly elastic character. Composed predominantly of glycine and proline, elastin delivers flexibility that is unrivaled by other proteins. The sequence of these amino acids contributes to the protein's ability to stretch and recoil, mimicking the behavior of a rubber band.
One notable aspect is the presence of desmosine and isodesmosine, unique cross-linking amino acids that form connections in elastin's structure. These links are essential in maintaining the integrity of elastic fibers. The balanced ratio of hydrophilic and hydrophobic amino acids also plays a role, giving elastin its ability to interact with the surrounding environment while retaining its structural properties.
"Elastin isn't just a passive structural element; it actively participates in the dynamic behaviors of tissues."
Molecular Architecture
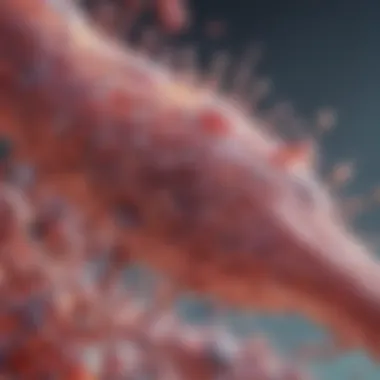
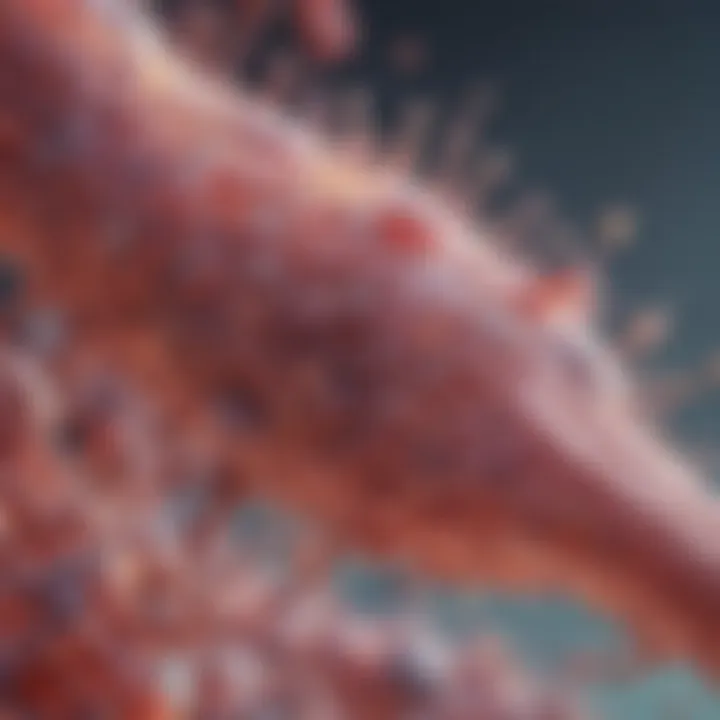
The molecular architecture of elastin is a complex interplay of chains that are intricately woven. The primary structure consists of long, coiled polypeptide chains which provide the protein its potential for elasticity. These chains form a scaffold with embedded cross-links that are essential for providing durability under stress.
Moreover, the secondary structure, which often takes the form of alpha helices and random coils, allows elastin to adapt its shape dynamically with any elongation or compression forces it experiences. This adaptability is particularly important in tissues that undergo frequent stretching, such as the skin or arterial walls. The tertiary structure leaves room for the protein to fold over itself, further enhancing its ability to return to its original conformation after deformation.
Cross-linking and Elasticity
Cross-linking is a vital feature that underpins the functionality of elastin. It not only enables the fibers to withstand mechanical stress but also determines how they respond to forces and environmental changes. These cross-links are crucial for maintaining the stability and elasticity of elastin fibers within the extracellular matrix.
The mechanical properties of elastin, such as its Young's modulus, are directly correlated with these cross-linking configurations. For instance, the more extensive cross-linking structure can enhance tensile strength while still allowing for elasticity. This balance is what allows elastin to endure both stretch and compression, making it indispensable for functions like skin resilience and vascular flexibility.
In summary, the structure of elastin—shaped by its unique amino acid composition, molecular architecture, and intricate cross-linking—plays a critical role in its function, underscoring its importance in the maintenance of tissue health and elasticity.
Biological Functions of Elastin
Understanding the biological functions of elastin is an integral component of grasping its overall significance in the human body. This protein not only facilitates the elasticity of numerous tissues but also supports vital physiological processes. Its ubiquity in various systems highlights its multifaceted roles and the serious consequences that can arise from elastin deficiency. Here, we delve into three critical areas where elastin plays a decisive role, starting with the functionality of tissues, the implications in vascular health, and the contribution to overall organ functionality.
Role in Tissue Elasticity
Elastin's primary function is to provide elasticity to connective tissues, allowing them to stretch and recoil. This characteristic is especially prominent in organs such as the lungs, skin, and blood vessels. For example, in the skin, elastin works in conjunction with collagen to maintain firmness and resilience. When elastin levels are adequate, skin can easily adapt to movements and changes in shape. The relationship between elastin and collagen is intricate; while collagen lends strength, elastin ensures flexibility. Without sufficient elastin, a person may notice wrinkling, sagging, or even loss of mobility in tissues.
- Key Functions of Elastin in Tissues:
- Allows for the flexibility necessary for movement.
- Supports ability to return to normal shape after stretching.
- Involved in sustaining vital functions of organs and systems.
Implications in Vascular Health
The significance of elastin in vascular health cannot be overstated. Blood vessels, particularly arteries, rely on elastin's properties to accommodate fluctuating blood pressures and support unimpeded blood flow. The elasticity of these arteries allows them to expand and contract in response to the heart's pumping action. A notable decline in elastin can lead to stiffening of the arteries, contributing to conditions such as hypertension and atherosclerosis. When discussing vascular diseases, it's notable that any impairment in elastin production can worsen the health of arteries, emphasizing elastin's role in preventing cardiovascular ailments.
"Without elastin, our blood vessels would be less able to adjust to the body's demands, leading to increased risks of cardiovascular issues."
Contribution to Organ Functionality
In addition to its roles in tissue elasticity and vascular health, elastin significantly contributes to the functionality of various organs. For example, in the lungs, elastin fibers allow for the essential expansion and contraction required for breathing. This elasticity is crucial because every inhalation and exhalation demands a flexible structure to facilitate airflow. Similar mechanisms are observed in the bladder, where elastin supports its ability to stretch as it fills with urine.
- Impact of Elastin on Organ Functionality:
- Enables lungs to function effectively during respiration.
- Facilitates proper expansion in the bladder.
- Supports the structural integrity of various organs under physical stress.
In summary, elastin is not merely a structural protein; it serves numerous biological functions that are vital for maintaining health. Its roles in tissue elasticity, vascular health, and organ functionality highlight why elastin is essential for a well-operating body.
Synthesis and Regulation of Elastin
The topic of synthesis and regulation of elastin is fundamentally about how our body creates and maintains this crucial protein. Understanding the mechanisms behind elastin biosynthesis is not just a biochemistry exercise; it plays a vital role in comprehending how our tissues function, age, and respond to various stresses. When we consider elastin, we’re not just looking at a protein, but a linchpin for tissue elasticity that impacts skin resilience, vascular flexibility, and much more.
Production Mechanisms
The production of elastin is primarily orchestrated by specialized cells known as fibroblasts, which are the silent workhorses in the connective tissues. They are responsible for synthesizing elastin along with other components like collagen. This synthesis process is intricate, primarily involving several steps:
- Transcription and Translation: Initially, the gene responsible for elastin is transcribed into mRNA within the cell nucleus. This mRNA then travels to the cytoplasm where ribosomes translate it into a precursor protein called tropoelastin.
- Secretion: Tropoelastin is secreted into the extracellular matrix (ECM) by the fibroblasts. The ECM serves as a supportive network, guiding the structural organization of tissues.
- Cross-linking: After secretion, tropoelastin units begin to cross-link under the influence of enzymes like lysyl oxidase, forming a dense elastic network. This process is critical, as it determines the elasticity and tensile strength of tissues.
By understanding these steps, researchers can better appreciate how alterations in elastin production might affect health. For instance, if certain fibroblast functions are compromised, it could lead to insufficient elastin levels, adversely affecting tissue resilience.
Regulatory Factors
In the realm of elastin synthesis, not every variable is under the direct control of the fibroblasts. Various regulatory factors closely influence how significantly elastin is produced:
- Growth Factors: Molecules such as transforming growth factor-beta (TGF-β) play a significant role. They stimulate fibroblast activity, promoting elastin synthesis.
- Mechanical Stress: The application of mechanical forces to tissues can also modulate elastin production. When tissues are subjected to stretching or other forces, fibroblasts respond by increasing elastin synthesis, thus adapting to the demands.
- Inflammatory Cytokines: On the flip side, certain cytokines associated with inflammation can inhibit elastin production, showcasing the dynamic balance of growth and decay in tissue function.
"Elastin regulation is a fine-tuned orchestra – where growth signals, mechanical stimuli, and inflammatory responses play vital parts."
These regulatory aspects underline the importance of elastin not just in normal physiology but also in pathological conditions. A thorough grasp of these factors is essential for developing targeted therapies aimed at restoring elastin levels and improving tissue function. Through the lens of elastin synthesis and regulation, we see a snapshot of a more extensive, intricate biological narrative that shapes both health and disease.
Degradation of Elastin
Elastin, the protein famed for its role in imparting elasticity to tissues, doesn’t just exist in a static state. Its degradation is a crucial process that affects tissue health and functionality. Understanding the degradation of elastin gives insights into not only the biological aging process but also various pathological conditions. This section focuses on two primary aspects: enzymatic breakdown and the multiple factors that influence the degradation rate of elastin.
Enzymatic Breakdown
The breakdown of elastin is primarily carried out by specific enzymes known as elastases. These enzymes can be endogenously produced or introduced into the body from external sources. They play a critical role in remodeling tissues and maintaining homeostasis. However, when elastin is broken down too rapidly or beyond natural limits, it can lead to structural anomalies in various tissues.
Some of the key features of enzymatic breakdown include:
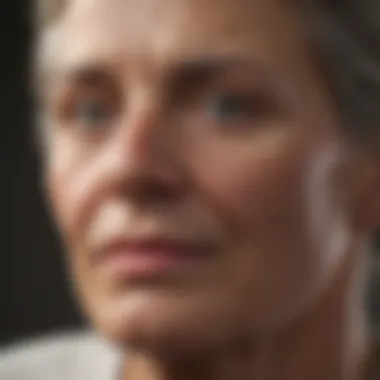
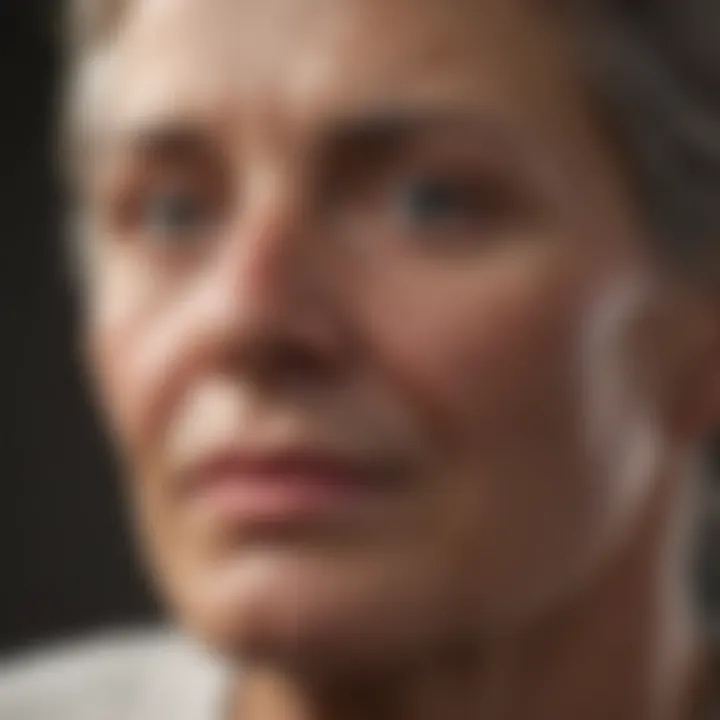
- Proteolytic Activity: Elastases hydrolyze peptide bonds in elastin, leading to two major outcomes: recycling of amino acids and modulation of tissue elasticity.
- Regulation: This enzymatic activity is typically regulated by the body’s natural inhibitors, such as alpha-1 antitrypsin. An imbalance in these inhibitors can lead to excessive elastin degradation.
"There’s a fine line between necessary degradation for tissue health and destructive breakdown causing disorders."
In various diseases like emphysema or chronic obstructive pulmonary disease (COPD), an abnormal increase in elastase levels can wreak havoc on lung function. Thus, understanding this breakdown process is pivotal in addressing conditions related to elastin deficiency.
Factors Influencing Degradation Rate
The rate at which elastin degrades is not uniform; it is influenced by a multitude of factors. Understanding these factors can provide valuable insights into preventive and therapeutic measures.
- Aging: As we grow older, elastin production slows down, and the existing elastin begins to degrade faster. The result? Skin loses its firmness, and joints may become stiffer.
- Environmental Factors: Exposure to ultraviolet (UV) radiation from the sun is a significant contributor to elastin degradation. UV light can damage the structural fibers of elastin in the skin, contributing to premature aging and loss of elasticity.
- Hormonal Changes: Hormonal fluctuations, particularly during menopause, can also affect elastin levels. The decrease in estrogen levels is often linked to a decline in elastin synthesis, exacerbating age-related changes.
- Lifestyle Choices: Diet and exercise are crucial. High levels of sugar and an unhealthy lifestyle can lead to glycation, a process that can impair elastin and increase its breakdown. Meanwhile, regular exercise promotes better blood circulation and nutrient delivery to tissues, contributing to elastin preservation.
Additional factors can include genetic predispositions and chronic illnesses, which can alter both the quantity and quality of elastin in the body.
In summary, the degradation of elastin is a complex interplay of enzymatic actions and external influences. Recognizing the dynamics behind this process not only enhances our understanding of tissue health but also opens avenues for potential therapeutic approaches.
Elastin and Aging
Elastin, a critical protein for maintaining the elasticity and resilience of various tissues, undergoes significant changes as we age. Understanding the impact of aging on elastin is essential not only to comprehend the normal physiological changes but also to dissect its implications in various health conditions. As people move past their youthful years, the body’s ability to produce elastin diminishes, leading to various structural challenges in our tissues.
Changes in Elastin Levels Over Time
Elastin levels naturally fluctuate throughout a person’s lifetime. In youth, elastin is abundant, allowing for smooth and flexible skin as well as efficient functioning of arteries and organs. However, studies indicate that elastin synthesis peaks in childhood and gradually declines after the age of 20. By the time we hit middle age, the quantity and quality of elastin can significantly drop, resulting in stiffer, less elastic tissues. The reduction in elastin production is often compounded by factors such as environmental stressors, diet, and lifestyle choices.
Several interconnected elements contribute to this decline:
- Genetic Factors: Variability in genes responsible for elastin synthesis can influence the body's ability to maintain adequate elastin levels.
- Hormonal Changes: The natural fluctuations in hormones linked to aging can adversely affect elastin production.
- Environmental Influences: Sun damage, pollution, and lifestyle habits such as smoking can degrade elastin and accelerate its breakdown.
The ramifications of decreased elastin can manifest in noticeably sagging skin, deterioration of vascular elasticity, and less elastic lungs, all of which may contribute to a range of age-related conditions.
Effects on Skin and Tissues
The reduction of elastin can have pronounced effects on the skin and other connective tissues. As the quantity of elastin fibers decreases, the skin may become less resilient, leading to more pronounced wrinkles, decreased suppleness, and even conditions like sagging. The clinical observations are stark:
- Visible Signs of Aging: Lines and wrinkles arise due to the inability of the skin to maintain its shape and bounce back after movement. The dermis can start looking weathered, impacting overall aesthetic appeal.
- Impact on Vascular Health: Without adequate elastin, blood vessels can become rigid and less able to handle fluctuations in pressure, increasing the risk of conditions such as hypertension.
- Lung Functionality: Reduced elastin in lung tissue can compromise the functionality of these organs, leading to decreased respiratory health as elasticity wanes.
"Elastin deficiency can act as a double-edged sword, not only restricting the physical elasticity of tissues but also harboring potential pathways to disease development."
Overall, the interplay between aging and elastin is complex. Maintaining elastin levels is crucial for preventing a host of age-related complications and ensuring a better quality of life as we advance in age.
Pathological Implications of Elastin Deficiency
Elastin's elastic properties are not just crucial for the flexibility of our skin; they are significant in maintaining the integrity of various organs and systems in the body. A lack of elastin or its dysfunction can lead to a plethora of health issues, underscoring the need for a deeper look into the pathological implications of elastin deficiency. The importance of understanding this deficiency cannot be overstated as it paves the way for medical advancements and therapeutic strategies in dealing with related diseases.
Relevance to Cardiovascular Diseases
Elastin plays a vital role in the cardiovascular system. The structure of the aorta and other major arteries is largely dependent on elastin fibers, which allow for the required flexibility during blood flow. Without sufficient elastin, the arteries can become stiff, leading to conditions such as hypertension. The stiffness can contribute to arterial hypertension, which is a major risk factor for heart attacks and strokes.
Research indicates that degradation of elastin occurs with age or in certain diseases, further compromising vascular health. This degradation disrupts the delicate balance needed for proper vascular function.
"A healthy elastin network not only supports elasticity but also helps to regulate blood flow, reducing the risk of cardiovascular events."
Association with Lung Disorders
In the lungs, elastin provides the necessary springiness for the alveoli, enabling adequate gas exchange. A deficiency here is particularly troubling, as it can lead to respiratory conditions such as emphysema. In this condition, the loss of elastic fibers means that the lungs struggle to expel air. As a result, this can cause the lungs to become hyperinflated, severely limiting breathing capacity. Furthermore, conditions like chronic obstructive pulmonary disease (COPD) also show a strong correlation with elastin loss, impacting the overall quality of life for those affected.
Impacts on Skin Conditions
Elastin's role in youthful, resilient skin is widely recognized, yet its deficiency can lead to numerous skin ailments. As elastin levels decline, skin loses its firmness and elasticity, leading to conditions like sagging and wrinkles. Beyond cosmetic concerns, this decline can also exacerbate certain skin disorders, including skin tears and chronic wounds. The implications can be profound, affecting not only appearance but also skin health and integrity. Moreover, conditions like Ehlers-Danlos syndrome spotlight the severe effects of elastin deficiencies, resulting in fragile skin and increased bruising.
In summary, elastin deficiency carries significant pathological implications, affecting various organ systems and leading to a range of diseases. By further understanding these connections, the potential for innovative therapies and interventions to counteract elastin loss continues to grow.
Therapeutic Approaches Targeting Elastin
As we delve into the world of elastin, the discussion must turn towards therapeutic strategies that aim to harness or restore the functionality of this crucial protein. Given elastin's pivotal role in maintaining tissue elasticity and structural integrity, its therapeutic potential becomes a hot topic. Researchers and clinicians are increasingly focused on how to either mimic elastin's properties or replenish its presence in aging or damaged tissues.
Innovations in Elastin Mimetics
The quest for elastin mimetics—a class of synthetic compounds designed to replicate the functions of elastin—has gained significant traction in recent years. These mimetics present numerous benefits. Firstly, they can serve as substitutes in tissue engineering, offering a scaffold that promotes cellular attachment and growth in a manner similar to natural elastin.
Moreover, elastin mimetics hold promise for treating various conditions characterized by elastin deficiency, such as chronic venous insufficiency or skin aging. These synthetic compounds may potentially enhance the elasticity of the compromised tissues, leading to improved functional outcomes.
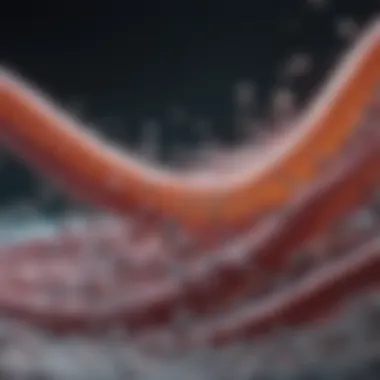
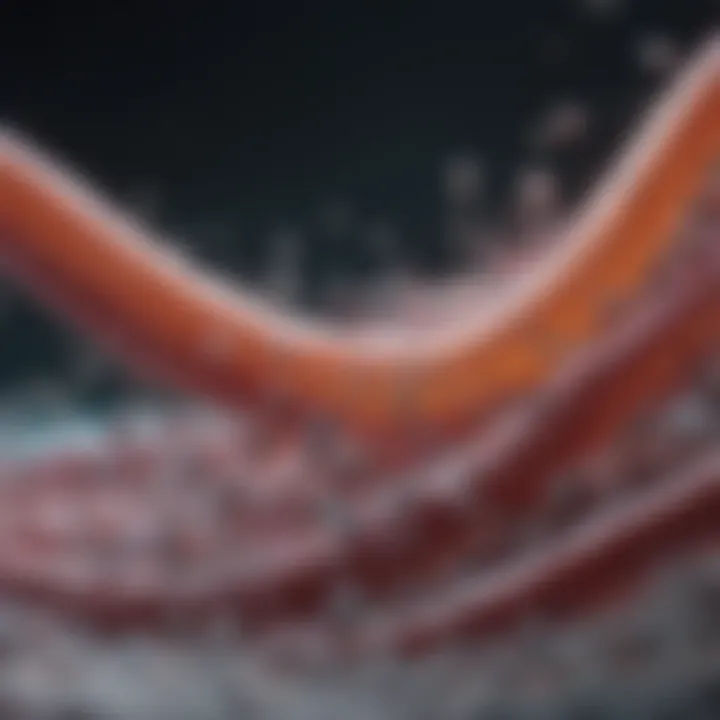
Several innovative approaches aim to develop these mimetics. Some utilize bioengineering techniques to create peptides that closely resemble elastin’s structure and functionality. Others explore naturally occurring elastin-like polypeptides that have been modified for enhanced durability and performance.
In addition, the ongoing research investigates how these elastin mimetics can be delivered effectively to the target tissues. Localized delivery through hydrogels or patch systems might enhance the efficacy and reduce potential systemic effects.
"Innovations in elastin mimetics are not just about replacement; they’re about creating a new paradigm in regenerative medicine that bridges the gap between synthetic and biological solutions."
Replenishment Strategies
Replenishment strategies focus on techniques aimed at increasing the levels of natural elastin in the body. Understanding the mechanisms behind elastin synthesis is essential for developing effective replenishment strategies. A promising avenue is to utilize growth factors known to enhance elastin production. For instance, transforming growth factor-beta (TGF-β) has shown potential in stimulating elastin synthesis in fibroblasts.
Moreover, recent advances in gene therapy offer another potential pathway for elastin replenishment. These approaches involve modifying the expression of genes responsible for elastin production, potentially restoring normal levels of elastin in affected tissues.
In clinical environments, topical therapies containing growth factors or other signaling molecules are being explored for their ability to stimulate or enhance elastin production in the skin. Such therapies hold the potential not just to rejuvenate aging skin but also to provide therapeutic benefits in conditions where elastin is significantly diminished.
To summarize, both elastin mimetics and replenishment strategies are frontiers in elastin science, each offering unique approaches to address the challenges posed by elastin deficiency. As research progresses, it becomes clear that targeting elastin—be it through innovative mimetics or strategic replenishment—presents a vital opportunity for the treatment of a range of disorders.
Experimental and Clinical Research
Research into elastin protein has evolved substantially and holds significant promise in both understanding its biological functions and developing therapeutic strategies. This section aims to shed light on the current state of experimental investigations and clinical trials focused on elastin, emphasizing their relevance in unraveling the complexities of elastin-related conditions. By analyzing these studies, we can discern how they address existing knowledge gaps and pave the way for innovative treatments.
Current Studies on Elastin's Role
Recent investigations have broadened our understanding of elastin's multifaceted roles beyond mere structural support. Notably, studies have focused on how elastin contributes to tissue resilience and responds to pathological changes.
- Tissue Response Mechanisms: Researchers have examined how elastin interacts with various cell types, including fibroblasts and endothelial cells, in response to mechanical stress and inflammation. These studies indicate that elastin isn't just a passive player; it actively participates in signaling pathways that regulate tissue repair and remodelig.
- Elastin in Disease Contexts: A growing body of research highlights elastin's altered expression in diseases such as aortic aneurysms and pulmonary issues. For instance, studies using animal models and human tissues reveal that decreased elastin integrity can exacerbate the symptoms and progression of such conditions.
- Molecular Techniques: Cutting-edge technologies like CRISPR and RNA sequencing are being employed to dissect the molecular underpinnings of elastin-related diseases. These tools enable researchers to manipulate elastin genes in controlled environments, providing insights into how genetic variations affect elastin's functionality and durability.
This research lays the groundwork for potential applications in regenerative medicine, offering tantalizing prospects for tissue engineering and other therapeutic environments.
Clinical Trials Assessing Therapies
Clinical trials examining elastin-based therapies reflect a burgeoning field. These trials target various aspects of elastin functionality and aim to develop interventions that could alleviate or reverse diseases linked to elastin deficiency.
- Novel Therapeutic Strategies: Some trials are investigating elastin mimetics, synthetic compounds that mimic the properties of natural elastin. Beneficial outcomes in vascular health have been reported, showing potential in preventing or treating conditions like hypertension and atherosclerosis.
- Replenishment Techniques: Other studies focus on strategies to enhance elastin deposition in tissues. This includes evaluating the effectiveness of growth factors such as transforming growth factor-beta (TGF-β) in stimulating fibroblast activity, thus boosting elastin synthesis in aged or damaged tissues.
- Assessing Safety and Efficacy: The clinical trials rigorously evaluate not just the efficacy but also the safety of new therapies related to elastin. Monitoring adverse effects and long-term outcomes is vital as researchers attempt to validate the promise of novel elastin-targeted strategies.
One trial, specifically aimed at assessing the safety and effectiveness of a specific elastin mimetic in adults with chronic obstructive pulmonary disease, reported improvements in respiratory function and quality of life metrics, highlighting the potential of elastin-targeting therapies.
Overall, the ongoing experimental and clinical research is crucial in unlocking the potential of elastin proteins. The insights drawn from these studies are anticipated to influence future therapeutic interventions, ultimately leading to enhanced patient outcomes in elastin-associated conditions.
Future Directions in Elastin Research
Elastin research is nothing short of a golden opportunity for a deeper understanding of flexible tissues in the human body. New advancements are shedding light on this elastomeric protein, which plays a crucial role in the elasticity of various organs such as the lungs, skin, and blood vessels. The future directions of elastin research look promising, not just for academic insight but for tangible benefits in medicine and biotechnology.
Emerging Technologies
Technological revolutions have a knack for transforming fields of study, and elastin research is no different. As we look forward, several emerging technologies hold potential to advance our understanding of elastin. For instance:
- CRISPR Gene Editing: This genetic editing tool allows researchers to modify genes precisely, paving the way to investigate how the alteration of elastin gene expression impacts both health and disease.
- 3D Bioprinting: The capacity to create bioengineered tissues can simulate the mechanical properties of elastin and provides new methods for repairing or replacing damaged tissues.
- Nanotechnology: Utilizing nanoparticles can enable targeted delivery of therapeutic agents that can regenerate or stabilize elastin, improving treatment efficacy.
These techniques can help create a more detailed picture of elastin's role in various biological processes, addressing long-standing questions about its elasticity and resilience.
Potential Impacts on Regenerative Medicine
The implications of elastin research stretch right into the realm of regenerative medicine. Understanding how to stimulate elastin production or repair its structure could reshape treatment approaches for many conditions.
- Skin Rejuvenation: With the knowledge gained, strategies could be devised to restore elastin levels in aging skin, leading to less invasive cosmetic procedures or better outcomes for existing treatments.
- Vascular Repair: Regenerating elastin in blood vessels could significantly enhance recovery from cardiovascular diseases. This could contribute to reducing complications and improving overall patient health.
- Pulmonary Function: In lung tissues, restoring elastin could be revolutionary for people suffering from chronic obstructive pulmonary disease (COPD) or other similar conditions by enhancing their lung elasticity.
These advancements not only promise better health outcomes but also signify a new era where combating the natural decline of elastin can lead to healthier, longer lives.
"Emerging technologies are not just tools; they're the key to unlocking new potential in elastin research and regenerative medicine."
Epilogue
The conclusion section of this article serves as an essential lens through which the multifaceted nature of elastin protein is synthesized. It is not merely a wrap-up of the findings; rather, it acts like the final brush strokes on a detailed painting that reflects the essential truth about elastin's role in human health. Understanding elastin's properties and functions has profound implications. It emphasizes the interconnectedness of structural integrity and tissue elasticity in the body, drawing a clear line to the broader health implications.
Summary of Key Findings
A comprehensive study of elastin reveals several critical points:
- Essence of Elastin: Elastin is crucial for enabling tissues to stretch and recoil, significantly impacting areas such as skin, lungs, and blood vessels.
- Synthesis and Regulation: The intricate processes by which elastin is synthesized and later regulated underscore the complexity of physiological mechanisms at play. Understanding these processes provides insight into potential therapeutic approaches.
- Aging and Degradation: A notable decline in elastin, particularly with aging, addresses the underlying reasons for reduced elasticity in tissues, presenting a direct line to conditions like arteriosclerosis and skin aging.
- Pathological Considerations: Deficiencies in elastin highlight the importance of this protein in cardiovascular and respiratory health, linking it to various disorders and paving the way for targeted interventions.
- Future Prospects: Novel therapeutic approaches focusing on elastin replenishment, such as the development of elastin mimetics, could revolutionize treatment options for diseases related to elastin loss.
These findings encapsulate the essence of elastin's relevance, aligning with the aim of the article to articulate the nuances of this pivotal protein.
Implications for Future Studies
Delving into elastin research opens numerous avenues for future exploration:
- Focus on Therapeutics: As the demand for effective treatments increases, researching elastin-enhancing therapies presents a ripe field. Understanding how to mimic or stimulate elastin production could lead to breakthroughs in regenerative medicine.
- Interdisciplinary Approaches: Future studies would benefit from integrating insights from molecular biology, material science, and clinical health to create multi-faceted strategies in tackling diseases associated with elastin deficiencies.
- Longitudinal Studies: Research designed to track elastin levels over time in diverse populations can shed light on how individual lifestyle choices influence elastin integrity and health outcomes.
- Technological Advances: The merging of biotechnology and elastin research holds the potential for innovative solutions, such as using biotechnology to create novel biomaterials that mimic elastin properties for surgical repair or aesthetic applications.
In closing, the exploration of elastin not only enhances scientific understanding but also lays the groundwork for future studies that could significantly alter health outcomes across various medical fields. The implications are vast, making elastin protein an enduring subject for scholarly discourse.