Electric Field Sensing: Principles and Applications
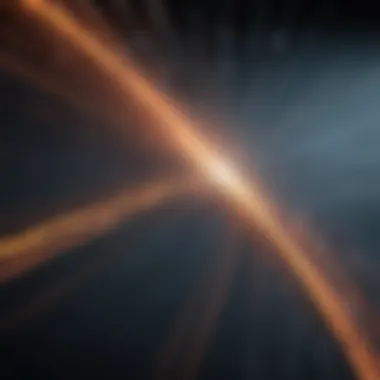
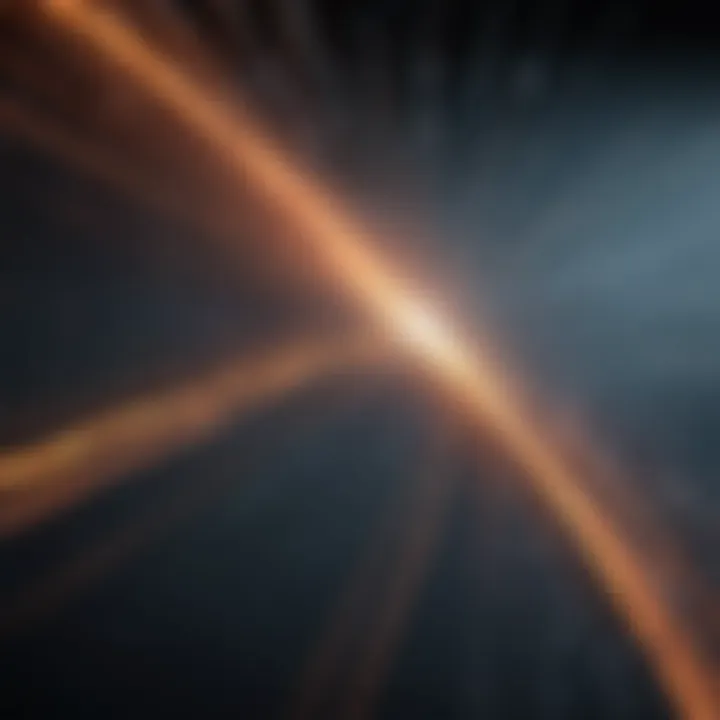
Intro
Electric field sensing has emerged as a vital technology, bridging numerous scientific and industrial disciplines. By detecting and measuring electric fields, researchers and professionals can gain insights into a wide range of phenomena. Understanding how electric field sensors work, along with their diverse applications, is key to grasping the modern technological landscape.
This article focuses on electric field sensing technologies, elaborating on the principles that govern their operation and their significant applications. Areas such as physics, engineering, and environmental science benefit greatly from these technologies. The growing demand for precision in measurements and increased sensitivity in detection has motivated advancements in electric field sensing methods.
Research Overview
Summary of Key Findings
Research indicates that electric field sensing technologies are diverse and applicable in multiple fields. The fundamental concepts involve the interactions of electric fields with materials, which can be measured using various techniques. The most common methods include capacitive sensing and electromagnetic field detection. Each technique has its strengths, providing unique insights based on the context of use.
Some key findings include the efficiency of specific materials in detecting electric field changes, and the importance of environmental factors in sensor performance. The calibration of these sensors to ensure accuracy has also been found essential.
Importance of the Research
The importance of electric field sensing research lies in its pivotal role in advancements across numerous sectors. In industrial applications, for example, electric field sensors ensure safety by detecting fault conditions in electrical installations. In environmental monitoring, they contribute to assessing the impact of electric fields on ecosystems.
Furthermore, the academic sector relies on precise electric field measurements to advance theoretical models and experimental setups. Understanding these principles empowers professionals and researchers to innovate and implement solutions that address complex challenges.
Methodology
Study Design
The methodology underlying electric field sensing research often involves a systematic approach. It typically begins with the selection of appropriate sensor technologies based on the desired measurements.
To ensure reliability, factors such as sensor type, material composition, and environmental variables are considered. The design phase includes simulations and prototypes, allowing researchers to refine their methodologies before wide-scale implementation.
Data Collection Techniques
Data collection is a critical component in electric field sensing studies. Techniques vary for different applications. Some common methods include:
- Capacitive Measurement: Involves collecting data through changes in capacitance due to varying electric fields.
- Electromagnetic Waves: Utilizes the propagation of electromagnetic waves to detect field strength and variations.
- Voltage Measurement: This method detects induced voltage in a sensor, which can be related back to the electric field.
Additionally, sensor calibration techniques are employed to enhance accuracy and reliability, ensuring that the data collected reflects the actual electric field conditions reliably.
"A diligent approach to calibration and data collection is imperative for obtaining accurate readings in electric field sensing."
Through careful application of these methodologies and techniques, future advancements in electric field sensing can be anticipated.
Prolusion to Electric Field Sensing
Electric field sensing represents a significant technological advancement in understanding and interacting with our environment. This discipline focuses on measuring and interpreting electric fields, which are intrinsic to many physical phenomena. Electric fields play crucial roles in various applications ranging from fundamental research in physics to practical applications in engineering and environmental monitoring. By understanding the principles and methodologies associated with electric field sensing, we can harness these fields for effective outcomes across numerous domains.
Definition of Electric Field Sensing
Electric field sensing refers to the methods and technologies used to detect and measure electric fields. An electric field is a physical field surrounding electric charges, which exerts a force on other charges within the field. The sensing techniques vary, incorporating both passive and active sensor elements, and can involve static or dynamic measurements. A proper understanding of electric field sensing is essential for both theoretical investigations and practical implementations, enabling more precise control and analysis of electric phenomena in the surrounding environment.
Historical Context
The journey of electric field sensing dates back to the early explorations of electromagnetism in the 19th century. Initially, experimentation focused on basic observation, with Andre-Marie Ampere and Michael Faraday setting foundational principles. Their work laid the groundwork for what would evolve into modern electric field sensing technologies. For many years, the field languished, constrained by technological limitations. The advent of solid-state electronics in the latter half of the 20th century spurred rapid advancements. Innovations such as capacitive sensors and Hall effect sensors enabled more accurate and sensitive measurements of electric fields. This historical evolution highlights the constant interplay between theoretical advances and technological capability in the field of electric sensing.
Current Relevance
In contemporary research and industry, electric field sensing is increasingly relevant. The rise of smart technologies and the demand for precise measurement systems reinforces the need for effective electric field sensors. Industrial applications, such as those found in automation and energy sectors, rely heavily on accurate electric sensing. Furthermore, environmental monitoring employs electric field technologies to assess pollution levels and electromagnetic interference. These applications underscore the critical nature of electric field sensing. As society advances, understanding and effectively implementing electric field sensing technologies will be key to fostering innovation and addressing new challenges in science and engineering.
Theoretical Foundations
Understanding the theoretical foundations of electric field sensing is essential for grasping its applications and relevance in various scientific and engineering disciplines. This section covers the basic principles, mathematical representation, and the factors influencing electric field measurements. By delving into these elements, readers can appreciate how electric field sensors operate and their significance in advancing both technology and knowledge across multiple fields.
Basic Principles of Electric Fields
At its core, an electric field is a region around charged particles. It manifests as a force exerted on other charged entities within its vicinity. This force can be quantified based on the electric charges present and their specific positioning. The relationship between charge, distance, and the resulting field strength is encapsulated in Coulomb's law. Understanding this fundamental principle is crucial in electric field sensing as it dictates how sensors detect variations in electric fields.
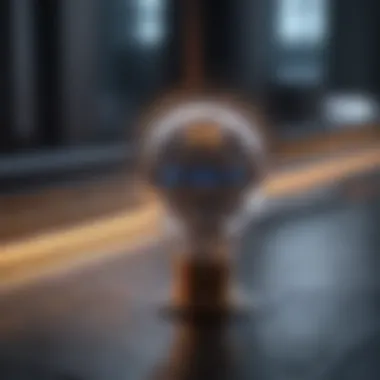
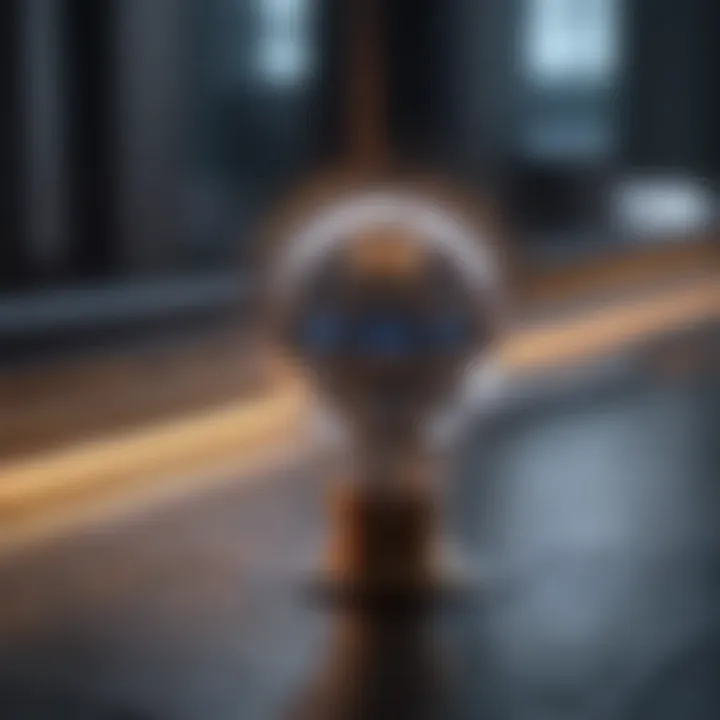
Electric field sensors rely on detecting changes in field strength. These changes indicate the presence of nearby charges or variations due to environmental factors. The sensors convert these fields into measurable electrical signals, allowing for practical applications in various domains.
Mathematical Representation
The mathematical representation of electric fields is foundational for understanding how measurements are taken. The electric field (E) created by a point charge (q) can be expressed as:
where:
- is the electric field strength,
- is Coulomb's constant, approximately equal to 8.99 x 10^9 N m²/C²,
- is the charge, and
- is the distance from the charge.
This equation signifies that the field strength diminishes with increased distance from the charge. In practical sensing applications, multiple charges and their geometric arrangements complicate field calculations. Therefore, sensors often utilize various models and computational methods to accurately interpret the data collected in dynamic environments.
Factors Influencing Electric Field Measurements
Several factors can influence the accuracy and reliability of electric field measurements. Understanding these factors is vital for optimizing the performance of electric field sensors.
- Geometric Configuration: The spatial arrangement of sensors relative to the charged bodies affects the measurements. The closer a sensor is to a charge, the stronger the detected field.
- Environmental Conditions: Changes in temperature, humidity, and atmospheric pressure influence the electric field and the sensor's response. Calibration under specific environmental conditions is often necessary for accurate readings.
- Material Properties: Different materials have varying dielectric properties that can affect field distribution and sensor accuracy. The choice of sensor materials plays a crucial role in determining the overall performance.
In summary, the theoretical foundations of electric field sensing encompass essential principles and mathematical frameworks. They also outline the factors influencing measurements. A sound grasp of these elements serves as a critical step towards effective application in advanced settings, including industrial and research environments.
Types of Electric Field Sensors
Understanding the different types of electric field sensors is essential. These sensors allow for a detailed analysis of electric fields in diverse environments. Key elements like sensitivity, response time, and application suitability vary greatly between types. This understanding helps researchers and engineers select appropriate technology for specific tasks. Comprehending these sensor types is crucial for maximizing efficiency and accuracy in electric field measurements.
Passive Electric Field Sensors
Passive electric field sensors operate without drawing power from an external source. They rely on the field’s existence to function. One major type is the capacitor-based sensor. These sensors have plates that accumulate charge through the electric field. The amount of charge accumulated correlates with the field strength. Therefore, they can provide a direct measurement of electric fields in their vicinity.
Advantages of passive sensors include:
- Low power consumption.
- High reliability as they contain no moving parts.
- Simplicity in design, making them easier to manufacture.
However, there are limitations. The sensitivity of passive electric field sensors can be lower than active alternatives. Their output can be influenced more by environmental conditions. Thus, while they are often suitable for stable environments, changes in temperature or humidity can affect their accuracy.
Active Electric Field Sensors
Active electric field sensors, in contrast, require an external power source. These sensors can provide enhanced sensitivity and faster response times. They often utilize methods such as capacitive, resistive, or inductive techniques to measure electric fields. For instance, the use of a charged probe can allow the sensor to sample the electric field more dynamically.
Benefits of active sensors include:
- Greater sensitivity and accuracy than passive models.
- Ability to operate in more varied and demanding conditions.
- Enhanced signal processing capabilities, allowing for better data acquisition.
Yet, it's important to consider some drawbacks. The reliance on an external power source can increase complexity. They can also require more intricate calibration processes, which may not be straightforward for all users. Additionally, active sensors can introduce noise into measurements because of their own electronic systems, potentially skewing results.
Comparison of Sensor Types
When comparing passive and active electric field sensors, several factors come into play.
- Sensitivity: Active sensors generally outperform passive models. This makes them ideal for applications requiring detailed analysis.
- Power: Passive sensors consume minimal energy, making them suitable for long-term deployments.
- Environmental Suitability: Active sensors can handle variable conditions better than passive sensors, which may struggle in fluctuating climates.
"Each sensor type has its strengths and weaknesses. Understanding these will lead to better decision-making in field measurements."
Measurement Techniques
In the realm of electric field sensing, measurement techniques form the backbone of accurate detection and analysis. These techniques are pivotal as they determine how well an electric field can be monitored, measured, and interpreted. Understanding the various methods enables researchers and engineers to select the appropriate technique for specific applications. Furthermore, the choice of measurement techniques influences the sensitivity and reliability of the sensing equipment, making it a critical topic in the broader discussion of electric field sensing.
Direct Measurement Techniques
Direct measurement techniques involve physically interacting with the electric field to obtain data. These methods often utilize sensors that can detect the electric field strength and direction directly. Common devices used in direct measurement include field probes and capacitive sensors. These tools can provide real-time data, which is essential for applications requiring instantaneous feedback.
One of the primary advantages of direct measurement techniques is their straightforward setup. Typically, they necessitate fewer complex calculations, allowing for immediate interpretation of results. According to several studies, these techniques are favored in environments where rapid assessments are critical, such as in industrial monitoring and research labs.
However, direct measurements also carry limitations. They can be affected by environmental interferences, which may distort the readings. Thus, users must consider external factors like proximity to other electrical devices or changes in temperature, which could affect precision.
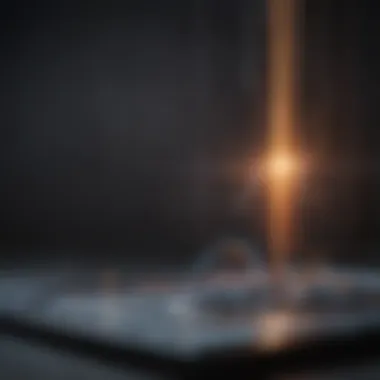
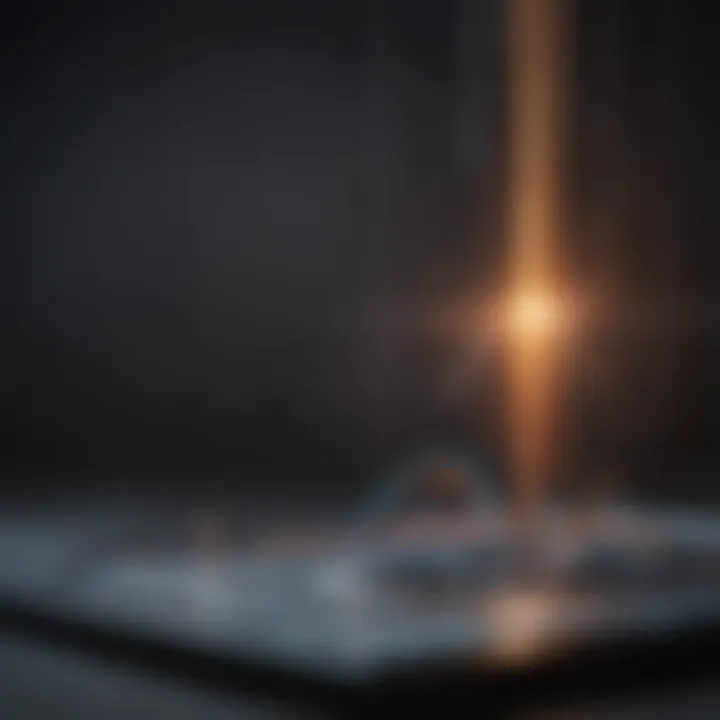
Inductive Measurement Techniques
Inductive measurement techniques capitalize on the principles of electromagnetic induction. This method often employs coils or inductive sensors that can detect changes in the magnetic field that arise from electric field variations. By measuring the induced electromotive force in the sensor, it is possible to infer the strength and nature of the electric field present.
These techniques have particular relevance in scenarios where physical intrusion into the environment is impractical. For instance, they are commonly used in hazardous areas where direct measurement might pose safety risks.
Moreover, inductive techniques can be advantageous in capturing changes over broad areas, thanks to their capacity to scan larger environments. Nonetheless, they require substantial calibration to ensure accuracy and can be influenced by nearby conductive materials affecting the electromagnetic fields. Thus, careful consideration and planning are essential when utilizing inductive methods.
Differential Sensing Methods
Differential sensing methods aim to mitigate noise and interference by comparing measurements taken from two or more positions. These methods typically utilize pairs of sensors arranged strategically to isolate the electric field signal from unwanted background noise. By contrasting measurements from different locations, these techniques enhance sensitivity and improve measurement accuracy.
The application of differential sensing proves invaluable in environments characterized by significant electromagnetic interference. For example, urban areas with numerous electrical devices can benefit from this approach, as it allows the sensor system to filter out extraneous signals. The advantage here is clear; users can gain clearer insight into the electric fields of interest.
However, differential measurement requires meticulous alignment of sensors and can introduce complexity in signal processing. Users must ensure that any changes in electric field strength are genuinely due to the phenomena of interest and not artifacts from sensor placement.
Key Point: Each measurement technique has unique strengths and weaknesses, and the choice often depends on the specific context and requirements of the application.
Calibration and Validation
Calibration and validation are critical aspects of electric field sensing. They ensure that the measurements obtained from sensors are accurate and reliable. In a realm where precision is paramount, effective calibration techniques can greatly enhance measurement quality. Calibration refers to the process of adjusting the sensor to meet specific standards of accuracy, while validation examines how well the sensor performs in an actual field setting.
Importance of Calibration
The importance of calibration cannot be overstated. It serves as a foundation for credible data collection. Without calibration, the readings from electric field sensors may be incorrect, leading to misguided conclusions and potentially dangerous decisions. Accurate calibration helps in identifying systematic errors and correcting them accordingly. By regularly calibrating sensors, users can maintain a high level of confidence in their measurements, which is vital for both research and industrial applications. This process also facilitates compliance with relevant industry standards, ensuring sensors operate within accepted margins of error. In summary, calibration is essential for ensuring data integrity and for enhancing the performance of electric field sensors.
Calibration Techniques
There are several calibration techniques commonly used in electric field sensing. These techniques include:
- Reference Standards: Utilizing known sensors with established accuracy helps ensure new sensors are reliable. The readings from these reference devices serve as benchmarks.
- Cross-Calibration: This involves using multiple sensors to measure the same electric fields. Comparing results can reveal inconsistencies and highlight necessary adjustments.
- Software Calibration: Advanced calibration techniques leverage software algorithms to adjust readings based on known errors and environmental variables.
- Environmental Adjustments: Factors such as temperature and humidity can affect measurements. Calibration techniques often account for these variables to improve accuracy.
Implementing these calibration strategies ensures that electric field sensors provide consistent and reliable data, essential for both experimental research and practical applications.
Field Validation Methods
Field validation methods serve to confirm the reliability and accuracy of sensor performance in real-world scenarios. These methods may involve:
- Field Testing: Conducting controlled experiments in varying environmental conditions helps assess sensor performance. This allows for observation of how sensors react to different electric field strengths and configurations.
- Comparison with Alternative Measurements: Validating sensor readings by comparing them with data obtained from unrelated methods or instruments can yield insights into their reliability.
- Long-Term Monitoring: Observing sensor performance over extended periods can help identify degradation or drift in measurements. Long-term data helps establish patterns that can signal when recalibration is necessary.
Challenges in Electric Field Sensing
Electric field sensing, while a vital tool in many scientific and industrial fields, encounters several significant challenges. Understanding these obstacles is essential for improving measurement accuracy and sensor performance. This section will delve into specific elements that complicate electric field sensing, examining technical limitations, environmental factors, and interference from various sources.
Technical Limitations
The technical limitations often encountered in electric field sensing revolve around the inherent capabilities of different sensors. Types of sensors—such as resistive, capacitive, or inductive—may exhibit varying degrees of sensitivity and accuracy. In some designs, resolution may be compromised due to physical constraints or sensor architecture, causing issues in precise field measurements.
A noteworthy technical limitation is the range of measurement, which can vary significantly depending on the sensor type and its configuration. For example, capacitive sensors might only effectively measure fields over short distances.
Calibration processes also play a crucial role in addressing technical limitations. Sensors that are not properly calibrated can give inconsistent results, leading to erroneous conclusions. It is critical that standard procedures are followed to ensure that sensitive equipment operates within its optimal parameters.
Environmental Factors
Environmental factors can significantly impact measurements. Variations in temperature, humidity, and atmospheric pressure can alter the behavior of electric fields. For instance, increased humidity can affect the conductivity of surrounding materials, which might introduce errors in readings from electric field sensors.
Natural phenomena, such as lightning or electromagnetic interference from nearby equipment, can also distort field measurements. Additionally, human-made structures can create localized disturbances, complicating the interpretation of data.
To mitigate these impacts, it is essential to conduct measurements under controlled conditions when possible. Field studies can also be designed to account for environmental variations by taking multiple readings in different conditions.
Interference and Noise
Interference and noise are perhaps the most notorious challenges in electric field sensing. Sensors may pick up unwanted signals from various sources, diluting the accuracy of the intended measurement. Electronic devices, radio frequency signals, and even nearby electrical infrastructure can introduce interference, leading to complex data interpretation.
Noise can appear in various forms, including thermal noise, shot noise, or flicker noise, depending on the sensor technology employed. This noise can obscure the subtle changes in electric fields that sensors are designed to detect. Therefore, it is vital to employ shielding techniques and advanced filtering algorithms to reduce noise levels.
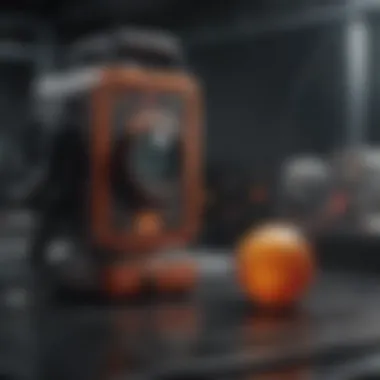
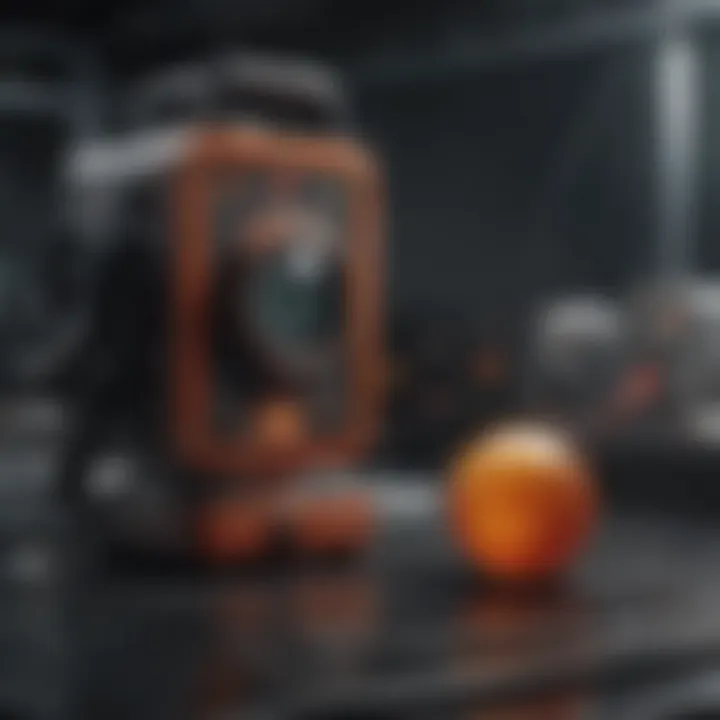
- Implementing noise reduction techniques can significantly enhance the reliability of measurements:
- Use of shielding materials to protect sensors from external electromagnetic fields.
- Application of digital signal processing methods to isolate and analyze relevant data more accurately.
Applications of Electric Field Sensing
Electric field sensing technology plays a pivotal role across a multitude of disciplines, which includes physics, engineering, and environmental science. Understanding its applications not only demonstrates the technology's versatility but also highlights its necessity in modern society. The benefits of electric field sensing devices encompass improved safety, enhanced data collection, and the facilitation of ground-breaking research. Specific considerations must also taken into account, including sensor accuracy, environmental adaptability, and practical deployment.
Industrial Applications
In industrial settings, electric field sensing is utilized to monitor and control processes with a high degree of precision. These sensors are integral in sectors like manufacturing, energy production, and telecommunications. For instance, electrical utilities leverage electric field sensors to manage their grid systems effectively. By measuring electric fields, they can monitor power levels, avert outages, and ensure efficient load distribution.
In manufacturing, electric field sensors can detect equipment malfunctions before they escalate. This predictive maintenance reduces downtime and operational costs. Additionally, in environments where safety is paramount, such as chemical plants, electric field sensing can identify potential hazards, supporting workforce safety and compliance with regulations.
"Electric field sensors not only streamline processes but also contribute to enhanced safety standards across various industries."
Research Applications
The scientific community heavily relies on electric field sensing for diverse research purposes. These sensors facilitate experiments aiming to understand physical phenomena, material properties, and biological processes. In experimental physics, researchers often use electric field measurements to study particle interactions and electromagnetic properties.
In the realm of material science, electric field sensing assists in characterizing materials at small scales. This capability allows for advancements in developing stronger, lighter, and more efficient materials. Additionally, in biological research, electric field sensors measure bioelectric processes, which provide insights into cellular functions and interactions.
Environmental Monitoring
Environmental applications of electric field sensing are gaining importance due to increasing concerns over ecological changes and human impact. Sensors can detect electric fields associated with various processess in plants and animals. This information leads to better understanding ecosystem dynamics.
In addition, electric field sensing technologies are implemented in monitoring pollution levels, tracking wildlife, and studying climate changes. The data gathered can help inform policy decisions and conservation efforts. Furthermore, these sensors can provide real-time data, which is crucial for timely responses to environmental issues.
Future Trends in Electric Field Sensing
Electric field sensing has evolved significantly over the years, and understanding the future trends in this domain is essential for grasping its potential impact across multiple sectors. As technology advances, the importance of electric field sensors will extend into new realms of application, ensuring their relevance in modern science and industry. Key elements such as emerging technologies, integration with the Internet of Things (IoT), and advancements in sensor sensitivity will shape the trajectory of electric field sensing.
Emerging Technologies
There are various new technologies that are reshaping the landscape of electric field sensing. One notable area is the use of micro-electromechanical systems (MEMS). These miniature devices make it possible to fabricate highly sensitive sensors on a small scale. Their integration into everyday applications is noteworthy. Active research in nanotechnology is also promising, as it can lead to innovations in sensor detection capabilities via the use of nanoscale materials. The incorporation of advanced materials, like graphene, is likely to enhance performance in both sensitivity and response time.
"Emerging technologies are providing innovative solutions that expand the boundaries of what electric field sensing can achieve."
Notable technologies include:
- Quantum Dots: Offering high precision and sensitivity in detection.
- Smart Materials: Adapting to environmental changes automatically.
- AI Integration: Leveraging artificial intelligence to process data and enhance prediction capabilities.
Integration with IoT
The fusion of electric field sensing with the Internet of Things holds considerable promise. IoT allows for the connectivity of devices, creating networks that can monitor conditions in real time. Sensors communicating with one another can enhance monitoring capabilities in diverse fields such as environmental science, agriculture, and even industrial automation.
For example, in precision farming, electric field sensors can relay data related to soil moisture levels and crop health, which can then be processed by cloud-based systems to optimize resource usage. This level of integration not only promotes efficiency but also opens new avenues for research and application.
Potential Advancements in Sensitivity
Sensitivity is a critical factor in the efficacy of electric field sensors. Future advancements are likely to focus on improving the sensitivity of these devices while reducing noise interference. One potential avenue for improvement is the development of algorithms that can filter out background noise more effectively, making it easier to discern small variations in electric fields.
Furthermore, developments in materials science, such as the use of highly sensitive conductive polymers, can yield sensors that detect minute changes in electric fields. Improving sensitivity will have profound implications for applications in fields such as medical diagnostics and environmental monitoring, where precise data can lead to better decision-making and enhanced outcomes.
By focusing on these future trends, stakeholders in electric field sensing can stay ahead of the curve, ensuring they leverage the latest technologies and methodologies to maximize efficiency and accuracy in their applications.
End
The conclusion of this article holds paramount importance as it synthesizes the myriad concepts discussed throughout. Electric field sensing technologies embody a significant intersection of theoretical knowledge and practical application. Understanding this field enhances not only academic inquiry but also informs advancements in technology across multiple sectors. Evaluating the principles and applications presented underscores the necessity for continual innovation in sensing methodologies.
Summary of Key Points
In summary, electric field sensing encapsulates various key elements that are essential for furthering our understanding of electric fields and their applications. Notably:
- Definition and Fundamentals: Electric field sensing is built on clear definitions and foundational principles that govern electric fields.
- Types of Sensors: The distinction between passive and active electric field sensors delineates their roles in varying contexts, underlining their respective advantages.
- Measurement Techniques: Direct, inductive, and differential measurement techniques delineate the methodological approaches to electric field sensing.
- Calibration and Challenges: Proper calibration techniques are critical for accuracy, as are comprehending the inherent challenges faced during measurement including environmental interference.
- Diverse Applications: The varied applications across industrial, research, and environmental domains highlight the versatility and importance of electric field sensing technologies.
Future Outlook
Looking ahead, the future of electric field sensing appears highly promising. With the emergence of new technologies and their integration into the Internet of Things (IoT), the sensitivity and functionality of electric field sensors will likely improve. Potential advancements may include:
- Innovation in Sensor Design: New materials and technologies could lead to the development of sensors that are more sensitive and less prone to interference.
- Increased Integration: As IoT continues to expand, electric field sensors may be integrated into smart systems for more robust data collection and analysis.
- Interdisciplinary Applications: Future trends may reveal novel applications in fields such as healthcare, energy management, and environmental science, driving new research avenues.