Fluorescence Micrographs: Techniques and Applications
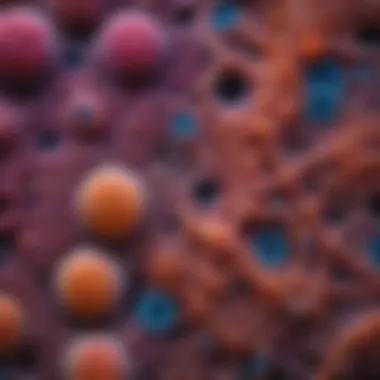
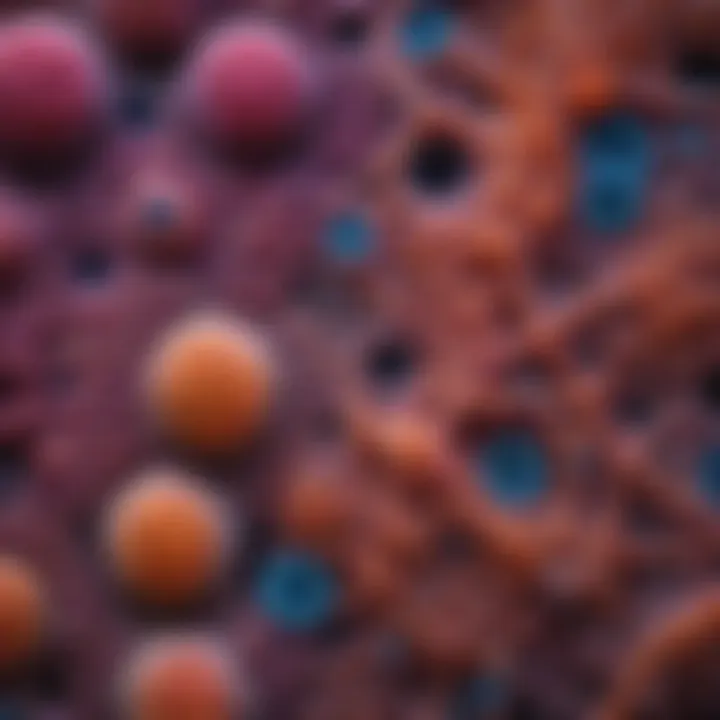
Intro
Fluorescence microscopy represents a revolutionary advancement in imaging technology. By utilizing specific wavelengths of light to excite fluorophores, researchers can capture detailed images of cells and tissues. These images, known as fluorescence micrographs, provide valuable insights into biological processes. The versatility and precision of fluorescence microscopy make it an essential tool in various scientific fields, from cellular biology to molecular genetics.
The significance of this technique goes beyond mere image capture. Fluorescence micrographs allow scientists to visualize cellular components, track dynamic processes in live cells, and conduct complex experiments that were not possible with traditional microscopy methods. As we explore this article, we will uncover the fundamental techniques, diverse applications, and methods for interpreting the nuances of fluorescence micrographs.
Prologue to Fluorescence Micrographs
Fluorescence micrographs are crucial tools in biological and clinical research. These images reveal detailed insights into cellular structures and functions. Understanding fluorescence micrographs can enhance researchers' capabilities to interpret complex biological phenomena. The ability to visualize specific components in cells provides a distinct advantage in various scientific disciplines.
Definition and Concept
Fluorescence micrographs are images obtained via fluorescence microscopy. This technique employs fluorophores, substances that emit light when excited by a specific wavelength. The emitted light is then captured to produce detailed images of samples. Currently, fluorescence microscopy represents a powerful approach for examining biological materials, allowing scientists to visualize structures that would otherwise remain hidden under regular light microscopy.
In essence, fluorescence micrographs excel in capturing the dynamic nature of biological processes. Researchers can observe interactions between proteins, study cellular dynamics, and analyze localization of materials within cells. This capability to visualize in real-time speaks to both the beauty and complexity of living systems, aiding both research and diagnostics across various fields.
Historical Background
The journey of fluorescence microscopy began in the early 20th century. The emission of light by materials when illuminated opened new avenues in microscopy. The foundational work by scientists such as Augustin Jean Fresnel and later, the advancements by Ernst Abbe in microscopy optics paved the way. Developments in fluorescent dyes in the mid-20th century further complemented microscopy techniques. Today, fluorescence micrographs are not only common in laboratories but have become essential in understanding numerous biological processes.
The growth of fluorescence microscopy has also expanded with the introduction of novel techniques and high-resolution imaging methods. Instruments have evolved from bulky and expensive to more accessible and sophisticated devices, making fluorescence micrographs a standard in contemporary research practices.
"Fluorescence microscopy has the potential to reveal features of biological processes that remain unexplored through conventional imaging methods."
By tracing the history and progress of fluorescence micrographs, one can appreciate not only their scientific implications but also the ongoing innovation within this field.
Fundamental Principles of Fluorescence
Understanding the fundamental principles of fluorescence is essential for demystifying how fluorescence micrographs are generated and interpreted. This section will discuss how fluorescence works, the types of fluorophores involved, and the significance of excitation and emission spectra. Mastery of these elements provides a solid foundation for anyone involved in fluorescence microscopy.
Fluorescence Mechanism
Fluorescence is a process by which certain substances absorb light at one wavelength and then emit light at a longer wavelength. This phenomenon occurs when an electron in a molecule absorbs energy, promoting it to a higher energy state. After a brief time, typically in nanoseconds, the electron returns to its ground state, releasing energy in the form of light.
In biological applications, fluorophores, or fluorescent dyes, are often utilized to label specific structures or proteins within cells. The interaction between fluorophores and biological specimens is complex and varies depending on the chemical properties of the fluorophore used. For instance, some fluorophores may be more stable or brighter than others, affecting the quality of the resulting micrographs.
"Fluorescence is not merely a lighting phenomenon; it is a transformative tool critical for research advancements."
Types of Fluorophores
There are several types of fluorophores, each with distinct properties that make them suitable for specific applications. Some common types include:
- Fluorescein: A bright dye with high sensitivity, often used in microscopy; it is ideal for visualizing cellular structures.
- Rhodamine: Known for its stability and brightness, Rhodamine provides excellent contrast for various cellular components.
- Quantum Dots: Nanoparticles that exhibit size-tunable luminescence, allowing for a broad range of colors and applications, especially in multiplexing.
- GFP (Green Fluorescent Protein): A naturally occurring protein that fluoresces green when exposed to light, widely used in live-cell imaging.
Choosing the right fluorophore is crucial, as it can significantly impact the clarity and applicability of the micrographs produced. Factors to consider include photostability, brightness, and the specific biological targets of interest.
Excitation and Emission Spectra
The characteristics of excitation and emission spectra are vital for successful microscopy. Each fluorophore has a specific range of wavelengths that it can absorb (excitation spectrum) and, conversely, a range of wavelengths at which it emits light (emission spectrum). Understanding these spectral properties enables researchers to select the appropriate filters and settings for their microscopy equipment.
Typically, the excitation spectrum peaks when the specific wavelength light is applied. Subsequently, the emitted light will be detected at the emission spectrum peak. It is important to ensure that there are no overlaps between different fluorophores being used in multi-labeling experiments, as this can lead to potential complications in interpreting the resulting images.
Mastering the fundamental principles of fluorescence is essential for advancing knowledge and techniques in fluorescence microscopy. By knowing how fluorescence works, the various fluorophores available, and the importance of spectral properties, researchers can conduct more accurate studies and interpret their data with confidence.
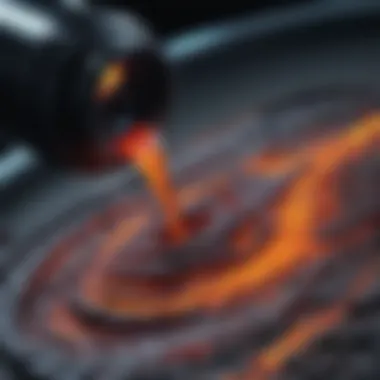
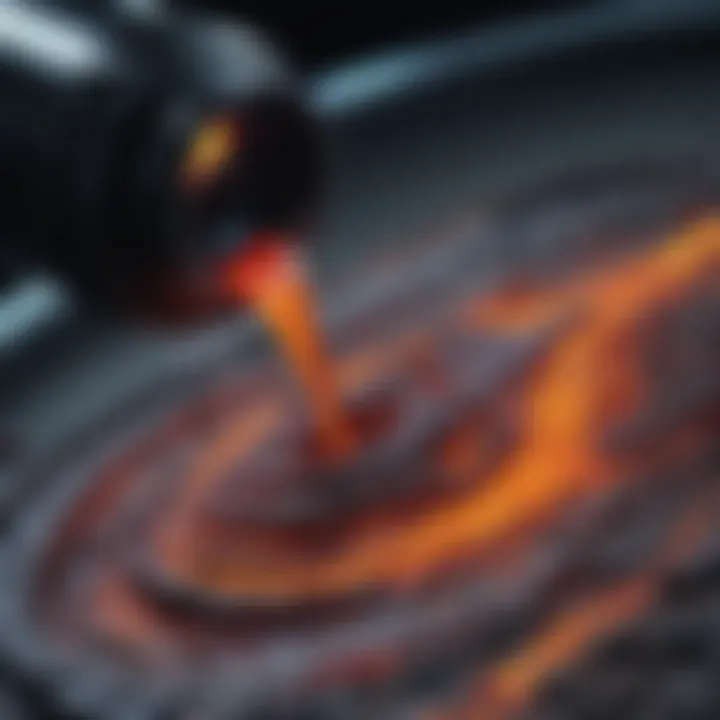
Fluorescence Microscopy Techniques
Fluorescence microscopy techniques play a crucial role in biological research and imaging. They provide scientists the ability to visualize and analyze cellular components in a way that is often difficult or impossible with traditional microscopy methods. Various techniques have been developed, each with unique strengths, applications, and considerations. Understanding these methods is essential for effectively harnessing their potential in research and clinical settings.
Widefield Microscopy
Widefield microscopy is one of the most basic forms of fluorescence microscopy. It allows for the simultaneous imaging of a wide area, thereby providing a comprehensive overview of a sample. This method illuminates the sample with a uniform light source, enabling the detection of emitted fluorescence from multiple fluorophores across a field of view.
Advantages of widefield microscopy include its simplicity and ability to capture dynamic processes in real time. However, it suffers from limitations related to photobleaching and background signal. The entire specimen is illuminated, often resulting in a higher background noise that can obscure finer details. Additionally, due to the loss of depth resolution, distinguishing between structures located closely within the Z-axis can be challenging.
Confocal Microscopy
Confocal microscopy addresses some of the limitations found in widefield microscopy by using a spatial pinhole in conjunction with a focused laser light source. This design allows for the capture of images at specific depths within the sample, which significantly reduces background noise. The capability of imaging across various Z-planes provides a three-dimensional perspective of cellular structures.
Confocal microscopy is particularly beneficial for studies requiring high resolution and detailed imaging. It also enables quantitative analysis of fluorescence signals, making it a favored choice in both research and diagnostic applications. Despite its advantages, confocal microscopy can be time-consuming and requires careful sample preparation. Furthermore, the reliance on laser sources can increase the likelihood of photobleaching in sensitive samples.
Total Internal Reflection Fluorescence (TIRF)
Total Internal Reflection Fluorescence (TIRF) is a specialized technique that excels at imaging events occurring near a sample's interface, such as cell membranes. TIRF employs an evanescent wave created by total internal reflection, allowing for excitation of fluorophores in very close proximity to the coverslip. This significantly enhances the signal-to-noise ratio, making it ideal for investigating molecular interactions at the cellular periphery or for live-cell imaging.
While TIRF offers high sensitivity and reduced background interference, its application is limited to examining events very close to the surface. Consequently, it is less suitable for studying complex three-dimensional structures within a sample. Researchers considering TIRF must also ensure proper sample thickness and refractive index matching to optimize imaging conditions.
Super-Resolution Microscopy
Super-resolution microscopy represents a significant advancement in fluorescence imaging, enabling users to visualize structures at resolutions beyond the diffraction limit of light. Techniques such as STED (Stimulated Emission Depletion) and PALM (Photo-activated Localization Microscopy) have transformed our understanding of molecular organization within cells.
By employing various means of manipulating fluorophore behavior, super-resolution techniques allow for the localization of individual molecules. This capability opens avenues for analyzing processes at the nanoscale, offering insights into cellular dynamics at an unprecedented level. However, these methods can be more complex and require specialized equipment and expertise. Their use may also necessitate considered fluorophore properties to achieve optimal results.
"Investing in the right microscopy technology is essential for acquiring valuable data that can advance scientific knowledge."
Well-planned application of these techniques can lead to significant innovations in understanding biological processes. As the field of fluorescence microscopy continues to evolve, remaining cognizant of these techniques will help researchers make informed choices in their imaging strategies.
Sample Preparation for Fluorescence Microscopy
Sample preparation is a critical aspect of fluorescence microscopy. The conditions under which samples are prepared directly influence the quality of the resulting fluorescence micrographs. Ensuring the samples are correctly fixed, stained, and mounted can enhance the visualization of specific cellular components, leading to more accurate interpretations of the data.
Fixation Techniques
Fixation is essential for preserving cellular structures and biomolecules in a state as close to their natural form as possible. It prevents degradation and maintains the integrity of the samples during observation. Common fixation methods include:
- Formaldehyde: This is widely used due to its effectiveness in cross-linking proteins, preserving the cell architecture.
- Methanol: Often employed for cytological preparations, it penetrates cells faster than formaldehyde and is good for preserving nucleic acids.
- Glutaraldehyde: This is another cross-linking fixative often used for electron microscopy, albeit it can introduce artifacts if not used properly.
Each fixation method comes with its benefits and limitations. For instance, formaldehyde is suitable for protein preservation, but excessive exposure can lead to autofluorescence issues. Therefore, choosing a fixation technique requires careful consideration of the intended application and the specific characteristics of the sample.
Staining Protocols
Staining enhances the contrast in fluorescence micrographs, making it easier to distinguish various structures within the sample. Various protocols exist, tailored to the specific requirements of the research. Key aspects include:
- Fluorophores Selection: Different fluorophores provide unique excitation and emission spectra. Choice depends on the target structures and the fluorescence microscopy technique used.
- Dyes and Probes: Options vary from general stains like DAPI for nuclear visualization to specific markers that target particular proteins or cellular processes.
- Concentration and Incubation Time: Optimal concentration and appropriate incubation times are crucial. Too little stain results in weak signals, while too much can lead to high background fluorescence.
Properly optimized staining protocols increase the specificity of the imaging process and improve the overall quality of fluorescence micrographs. Researchers must remain aware of the potential for photobleaching and background interference when selecting stains and protocols.
Mounting and Imaging Conditions
The mounting medium is vital in preserving the sample for imaging and reducing focus-related artifacts. Choosing the right mounting media can help maintain excitation and emission properties of the fluorophores. Options such as glycerol, mounting media with antifade reagents, and more specialized media like Vectashield are popular choices.
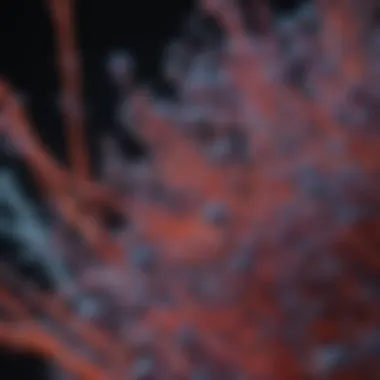
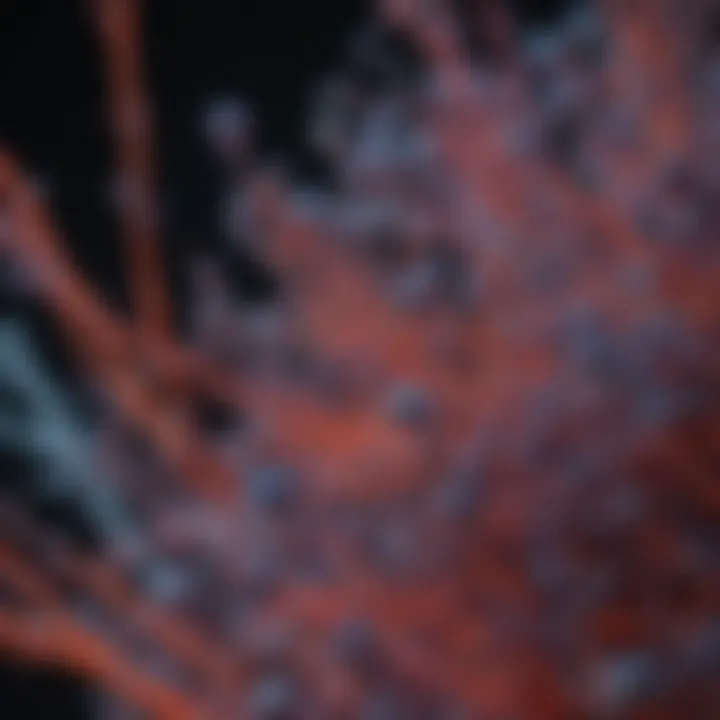
- Antifade Solutions: These formulations help to minimize the photobleaching during imaging, allowing for longer observation times.
- Refractive Index Matching: The index of the mounting medium should match that of the coverslip and the objective lens to reduce refraction and distortion.
Before imaging, it is critical to adjust the microscope's settings according to the sample's characteristics. This includes setting appropriate exposure times and using the correct filters for excitation and emission.
By understanding the significance of sample preparation, researchers can achieve clearer and more informative fluorescence micrographs, paving the way for deeper insights into biological processes.
Applications of Fluorescence Micrographs
Fluorescence micrographs offer significant insights across various scientific fields. This section focuses on the specific applications and their impact on advancing knowledge and research. The ability to visualize cellular structures and processes is crucial in many disciplines, enhancing our understanding of biological systems.
Cell Biology
In cell biology, fluorescence micrographs are invaluable. They allow researchers to explore cellular structures, dynamics, and interactions in high detail. The use of different fluorophores enables scientists to label specific proteins or organelles. This optical contrast helps in visualizing live cell processes in real-time. For instance, studying cell migration, division, and signaling pathways has advanced through these techniques.
Several techniques are used to visualize cells effectively. Widefield microscopy helps in obtaining images of shorter exposure times. Confocal microscopy enhances resolution and depth perception. These methods are pivotal in identifying cellular anomalies, offering early insights into diseases such as cancer.
Neuroscience
Fluorescence imaging significantly contributes to neuroscience. It provides a deeper understanding of neuronal structures and connections. With fluorophores that bind specifically to neural markers, researchers can visualize synaptic plasticity and neuronal communication. Methods like two-photon microscopy are particularly beneficial for studying live brain tissues.
The ability to label specific neuronal populations opens avenues for understanding complex brain functions. This includes exploring memory formation and sensory processing. The insights gained from fluorescence micrographs can lead to innovative therapeutic strategies for neurological disorders.
Clinical Pathology
In clinical pathology, fluorescence micrographs play a role in diagnostics and research. Pathologists utilize these images to identify diseases at the cellular level. Techniques like fluorescence in situ hybridization (FISH) help in detecting genetic abnormalities. This is crucial for cancer diagnosis, allowing for targeted treatment approaches.
Furthermore, fluorescence microscopy aids in understanding disease progression. The visualization of tumor microenvironments offers insights into tumor behavior and response to therapies. The precision of fluorescence micrographs in revealing cellular details underscores their importance in medical diagnostics.
Pharmaceutical Research
The field of pharmaceutical research also derives substantial benefits from fluorescence micrographs. These images are essential for drug discovery and development. Understanding how drugs interact with specific cellular components can guide the design of new therapies. Fluorescence micrographs help in evaluating drug efficacy, localization, and mechanisms of action.
In the realm of pharmacokinetics, visualizing drug distribution within cells is crucial. This enables researchers to optimize dosing regimens and minimize side effects. The intersection of fluorescence imaging with drug research is reshaping how new treatments are developed and assessed, making this application critical in the fight against diseases.
"Fluorescence microscopy has transformed our approach to biological research, enabling unprecedented visualization capabilities."
The applications of fluorescence micrographs are numerous and vital. Each field benefits uniquely, highlighting the interdisciplinary nature of this imaging technique. As technology advances, further applications are likely to emerge, enhancing scientific knowledge and understanding.
Interpreting Fluorescence Micrographs
Interpreting fluorescence micrographs is a critical step in analyzing and understanding the data derived from fluorescence microscopy. This section sheds light on the techniques employed to interpret these micrographs accurately and the implications of their interpretation in scientific research. Considering the nuances in fluorescence imagery, accurate interpretation can lead to increased reliability in scientific findings and bolster further explorations into cellular mechanisms.
Analyzing Image Quality
The quality of fluorescence micrographs directly affects the reliability of the conclusions drawn from them. Assessing image quality involves examining several factors:
- Signal-to-Noise Ratio (SNR): A high SNR enhances the visibility of the fluorescence signal compared to background noise, making it easier to identify specific structures or molecules within the sample.
- Contrast: Proper adjustment of contrast levels is essential. Low contrast may obscure details while overly high contrast may create artifacts.
- Resolution: The ability to distinguish between two separate points in an image is known as resolution. Techniques like super-resolution microscopy have revolutionized the ability to analyze small structures accurately.
- Dynamic Range: This refers to the range of light intensities that the imaging system can detect, affecting the recognition of different fluorescence populations.
In academic and clinical settings, utilizing tools for assessing these parameters can ensure image quality remains high. A comprehensive image quality analysis supports accurate interpretations, leading to robust biological inferences.
Quantitative Analysis of Fluorescence Intensity
Quantitative analysis is indispensable for drawing meaningful conclusions from fluorescence micrographs. This analysis involves measuring the intensity of fluorescence emitted by specific areas of a sample. It relies on several steps, including:
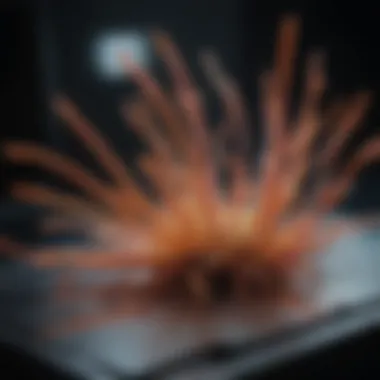
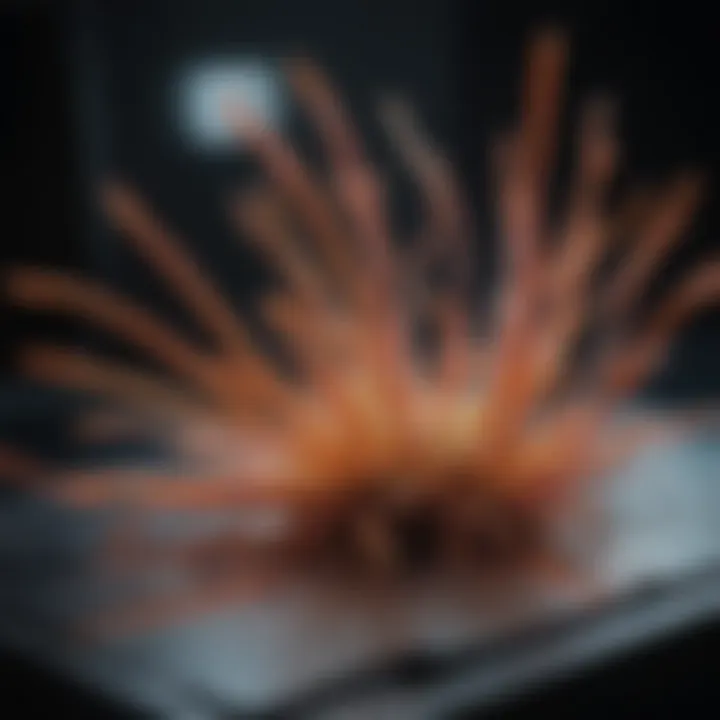
- Calibration: Establishing a standard intensity for the fluorophores is fundamental. Calibration ensures that the fluorescence intensity measured correlates appropriately to the number of molecules present.
- Intensity Measurements: Software tools can be used to measure intensity levels across various regions within the micrograph. This data can then be statistically analyzed to determine relationships within the biological system under study.
- Comparing Fluorophore Concentrations: By evaluating relative intensity across different samples or conditions, researchers can infer concentrations of targeted molecules or assess dynamic processes, such as cellular responses to drugs.
The integration of quantitative analysis into fluorescence studies contributes significantly to our understanding of complex biological interactions, enhancing the confidence in described outcomes.
Handling Artifacts and Background Noise
Interpreting fluorescence micrographs also necessitates awareness of potential artifacts and background noise. Common artifacts include:
- Photobleaching: The phenomenon where fluorophores lose their ability to fluoresce upon prolonged exposure to excitation light can lead to misleading interpretations.
- Cross-talk: This occurs when signals from one fluorophore overlap with those of another, particularly when multiple dyes are used simultaneously in multi-channel imaging.
- Out-of-Focus Blur: This can arise in widefield microscopy and may distort the perceived structures.
To minimize these issues, it is essential to implement:
- Appropriate Controls: Utilize negative controls or unstained samples to identify background fluorescence that may obscure results.
- Image Processing Techniques: Software-assisted processing can enhance the clarity of the images and reduce noise, allowing better visualization of the signals of interest.
- Choosing the Correct Dyes: Selecting fluorophores with minimal spectral overlap can help prevent cross-talk and improve overall image quality.
In summary, a comprehensive understanding of these aspects ensures that interpretations made from fluorescence micrographs are accurate and reliable, providing a solid foundation for future research.
Future Directions in Fluorescence Microscopy
Future directions in fluorescence microscopy hold promising implications for research across various fields. Advancements in technology are opening new avenues for visualization, analysis, and interpretation of biological samples. These developments not only enhance image quality but also expand the potential applications of fluorescence microscopy.
Emerging Technologies
Emerging technologies in fluorescence microscopy are set to revolutionize how scientists observe and analyze biological systems. Some notable advancements include:
- Multicolor Imaging: New labeling techniques allow for the simultaneous observation of multiple cells or structures. This capability provides richer data about interactions and functions within the same sample.
- Advanced Detectors: Modern detectors improve sensitivity and resolution. Techniques such as avalanche photodiode sensors contribute to lower light levels during imaging, reducing phototoxicity.
- Light Sheet Microscopy: This method illuminates the specimen from the side, creating a thin sheet of light. It permits imaging of live organisms in real time while minimizing damage to the samples.
- Machine Learning and AI: The integration of artificial intelligence is enhancing image analysis. Algorithms can now identify and quantify features automatically, streamlining data analysis processes.
These technologies not only provide clearer images but also facilitate longer experimentations with live samples. The continuous refinement of these tools ensures that researchers can meet the increasing demands for detail and accuracy in fluorescence microscopy.
Integration with Other Modalities
Integrating fluorescence microscopy with other imaging modalities provides a multi-faceted approach to studying complex biological systems. The combination of different techniques allows researchers to gather comprehensive data from samples. Some notable integrations include:
- Fluorescence and Electron Microscopy: This combination can overcome the limitations of conventional fluorescence microscopy regarding resolution. While fluorescence imaging provides functional information, electron microscopy offers detailed structural insights.
- Fluorescence and MRI: Merging techniques from magnetic resonance imaging allows for deeper insights when assessing live tissues. This is particularly useful in clinical settings where detailed anatomical and functional information is crucial.
- Fluorescent Imaging and Mass Spectrometry: This association brings together imaging techniques and biochemical compositional analysis. It is beneficial for understanding the localization of metabolites or proteins within a biological matrix.
As these technologies consolidate, they enable researchers to form a more holistic view of cellular processes. The capacity to gather information from different perspectives strengthens the overall analysis and fosters greater discoveries, particularly in fields like neuroscience and cellular biology.
The future of fluorescence microscopy promises to transform our approach to biological research by harnessing synergistic combinations of technologies.
Closure and Summary
The conclusion serves as a pivotal element in comprehending the intricate framework of fluorescence micrographs. It encapsulates the findings explored throughout the article, reinforcing the significance of fluorescence microscopy in biological research. Such imaging techniques not only offer insights into cellular structures and functions, but they also provide a visual narrative that illustrates complex interactions at the molecular level.
A core theme in the discussion of fluorescence micrographs is the evolving technology. Advancements in imaging methodologies continuously enhance our ability to visualize biological processes in real-time. This is key for researchers, educators, and students aiming to grasp the dynamics of life at the cellular scale. As the techniques evolve, so does the potential for breakthroughs in various research fields.
Furthermore, the concluding section emphasizes the responsibilities of the scientific community in applying these technologies thoughtfully and ethically. Issues such as reproducibility, the accuracy of interpretations, and transparency in methodologies warrant attention. The implications of improper use of fluorescence techniques could hinder progress in understanding vital biological phenomena.
"Understanding fluorescence micrographs empowers researchers to explore the unseen world of cells and their behaviors, thereby propelling science forward."
In summary, the conclusion brings together the essential discussions from previous sections, focusing on the pivotal role of fluorescence micrographs in illustrating biological realities. The comprehensive examination serves as a foundation for further exploration, underscoring the necessity for continued innovation and ethical conduct in research practices.
Key Takeaways
- Fluorescence microscopy is vital for visualizing cellular and molecular processes.
- Recent technological advancements enhance imaging capabilities.
- Ethical considerations are crucial for responsible application and interpretation of results.
- The ongoing development of fluorescence techniques holds promise for future research and discovery.
Implications for Future Research
The implications for future research stemming from the use of fluorescence micrographs are profound. As researchers continue to innovate in imaging technologies, several key trends emerge:
- Improved Resolution: New techniques promise to achieve unprecedented resolution, allowing scientists to visualize cellular structures with greater detail.
- Real-Time Imaging: With advancements like super-resolution microscopy, live-cell imaging becomes more reliable, enabling the study of dynamic processes in real time.
- Cross-Disciplinary Applications: Fluorescence techniques will likely find increasing applications beyond traditional fields such as cell biology, impacting areas like materials science and nanotechnology.
- Artificial Intelligence Integration: Incorporating AI for image analysis can expedite understanding by automating quantification processes in fluorescence micrographs.
In light of these trends, researchers must remain vigilant about the reproducibility of results and maintain transparency to support advancements in knowledge and applications. Continued exploration and development within the field promises to deepen our understanding of life at the cellular level.