Genome Sequencing Techniques: Innovations & Impacts
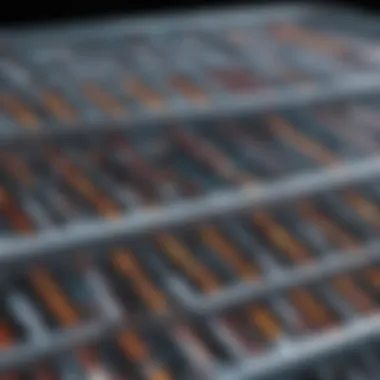
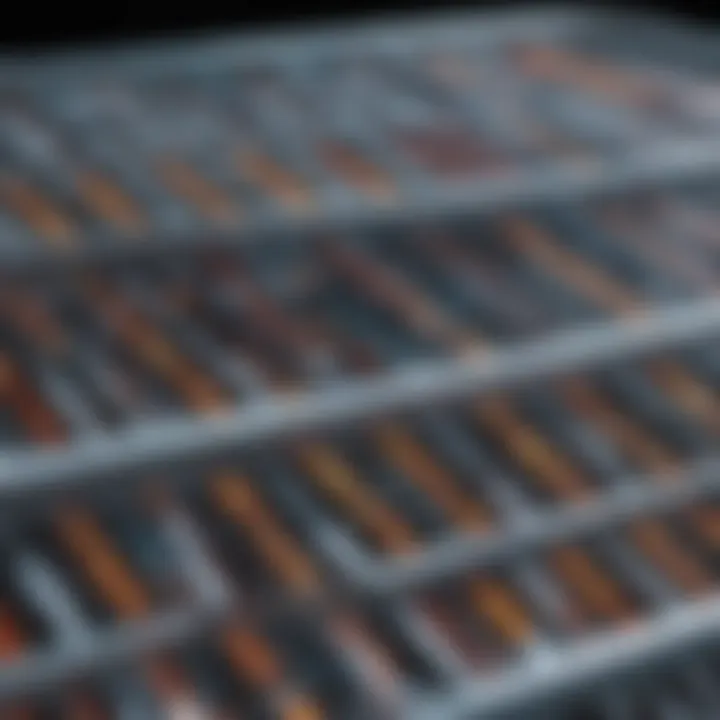
Intro
Genome sequencing is an essential tool in modern biology, facilitating a deeper understanding of genetic material. This article elucidates various techniques in genome sequencing, scrutinizing both classic and innovative methods. Each technique has unique operational principles, impacts, and implications across disciplines, including personalized medicine, evolutionary biology, and genetic research.
As we navigate this intricate field of study, we will dissect major methodologies, discuss their relevance, and assess advancements that enhance accuracy and reduce costs. This exploration aims to equip students, researchers, educators, and professionals with the insights needed to grasp the importance of genome sequencing in the scientific landscape.
Research Overview
Summary of Key Findings
The various techniques of genome sequencing offer distinct advantages and challenges. Our findings reveal that:
- Sanger Sequencing remains the gold standard, offering high accuracy but lower throughput.
- Next-Generation Sequencing (NGS) has revolutionized the field, providing faster results at a reduced cost.
- Third-generation sequencing technologies are emerging as powerful tools, allowing for direct sequencing of single DNA molecules.
Further analysis indicates that these methodologies are not equal; their utility varies based on research objectives and available resources.
Importance of the Research
Understanding the different genome sequencing techniques is vital for leveraging their potential in various applications. In personalized medicine, they enhance cancer treatments by tailoring therapies to genetic profiles, thus improving patient outcomes. Furthermore, in evolutionary biology, they offer insights into genetic variations, shedding light on species adaptation over time.
As research evolves, it is crucial to stay updated on technological advancements and their implications.
Methodology
Study Design
To examine the range of sequencing techniques, a comparative analysis approach was adopted. This method enables an in-depth understanding of the strengths and weaknesses inherent in each sequencing technique. The focus is placed on aspects such as accuracy, throughput, cost, and operational principles.
Data Collection Techniques
Data was collected from a multitude of peer-reviewed articles, industry reports, and databases. The selected publications span various timelines to present an inclusive review of historical and contemporary sequencing techniques. In instances where applicable, we relied on open-access resources for full transparency.
"As technologies advance, their implications in genetics and medicine become increasingly significant for our understanding of life itself."
In summary, genome sequencing opens numerous pathways for scientific inquiry and application. By assessing established and emerging techniques, we lay the groundwork for further discussion on their wide-ranging implications.
Foreword to Genome Sequencing
The field of genomics has evolved rapidly in recent decades, reshaping our understanding of biology and medicine. Genome sequencing is at the forefront of this revolution, enabling researchers to decode the genetic blueprint of organisms. This process not only highlights the intricacies of life but also paves the way for advancements in personalized medicine, genetic research, and evolutionary studies. As we explore this significant topic, we will detail the methods today’s scientists use, the implications of these techniques, and their potential to revolutionize health care and our understanding of life itself.
Definition and Importance
Genome sequencing is the comprehensive process of determining the complete nucleotide sequence of an organism’s DNA. This process includes both the identification of all the genes and the sequencing of the repetitive non-coding regions that comprise a significant portion of most genomes. The importance of genome sequencing lies in its ability to provide insights into the genetic information that underpins disease, development, and phylogeny.
With the advent of genome sequencing technologies, researchers gain access to a wealth of data that considerably enhances our understanding of genetic disorders, evolution, and species conservation. Moreover, it allows for personalized medicine, where treatments can be tailored based on an individual's genetic profile. As a result, the benefits derived from genome sequencing are profound and multifaceted, ranging from improved diagnostics to targeted therapies, making it a cornerstone of modern biological sciences.
Historical Context
The journey of genome sequencing has progressed through significant milestones over the last few decades. The first notable work comes from Frederick Sanger in the 1970s, who developed the Sanger sequencing method, allowing for the sequencing of smaller fragments of DNA. This method dominated the field for many years. Sanger's achievements included the sequencing of the first entire viral genome, which laid the groundwork for later developments.
The introduction of the Human Genome Project in 1990 marked a pivotal moment in the history of genome sequencing. This ambitious international initiative aimed at sequencing the entire human genome, and by 2003, it successfully mapped all the genes in human DNA. This extensive project not only advanced our scientific knowledge but also catalyzed the development of next-generation sequencing technologies.
As technologies have continued to evolve, techniques such as Next Generation Sequencing (NGS) have emerged. These enable the sequencing of entire genomes rapidly and at a reduced cost, facilitating a new era of genomic research. The historical context highlights how far the field has come and sets the stage for an in-depth examination of the various sequencing techniques that are now available.
Overview of Genome Sequencing Techniques
Genome sequencing is a critical process that enables scientists to map the genetic material of organisms. Understanding the different techniques is essential to unlocking the complexities of genetics. This section outlines the primary methods used in genome sequencing, highlighting their distinct advantages, limitations, and applications.
Next Generation Sequencing (NGS)
Next Generation Sequencing, commonly known as NGS, has revolutionized the field of genomics. It allows for the simultaneous sequencing of millions of DNA fragments, making it significantly faster than traditional methods. The benefits include higher throughput and decreased costs, leading to widespread adoption in various research fields. However, it comes with certain limitations that researchers need to consider.
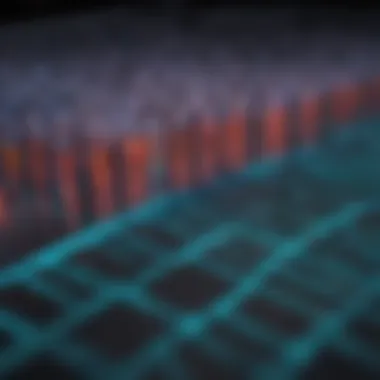
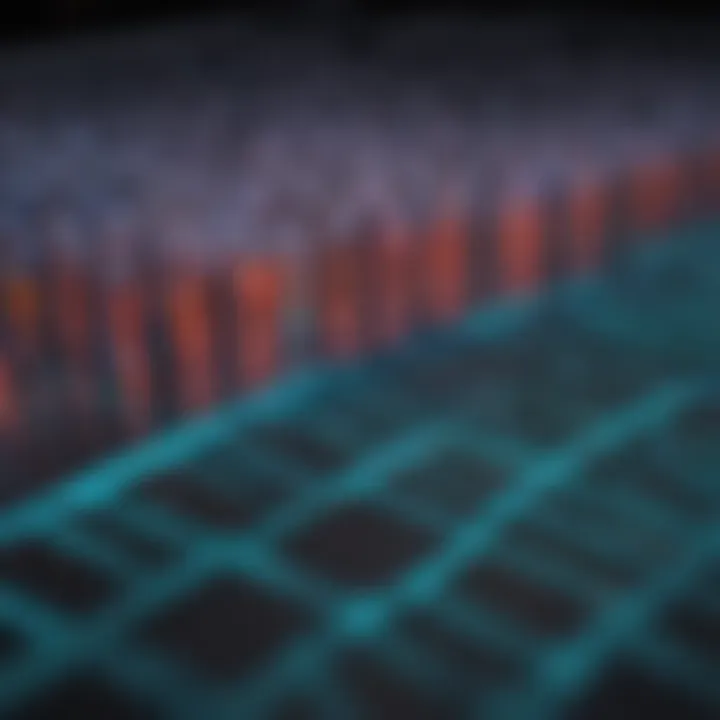
Advantages of NGS
The most notable advantage of NGS is its ability to sequence large amounts of DNA very quickly. This capability is particularly useful for projects that require comprehensive genomic information, such as population genomics. NGS offers a cost-effective solution compared to earlier methods like Sanger sequencing when analyzing extensive datasets. Additionally, its efficiency and accuracy make it a preferred choice among researchers looking to work with complex genomes.
Limitations of NGS
Despite its advantages, NGS is not without its challenges. One of the primary limitations is the difficulty in accurately identifying structural variations in genomes. NGS also involves significant computation power for data analysis, which can be resource-intensive. Furthermore, the quality of analysis heavily depends on the sequencing depth, leading to potential inconsistencies that can complicate results.
Sanger Sequencing
Sanger sequencing remains a foundational technique in genomics, known for its reliability. Developed in the 1970s, it provides precise data for smaller projects or target regions of interest. It is especially valued in validating sequences obtained through NGS, serving as a trusted methodology in many laboratories.
Principles of Sanger Sequencing
The principles behind Sanger sequencing involve using dideoxynucleotides for chain termination during DNA synthesis. This method allows for the generation of fragments that are then separated by size to determine the underlying sequence. Its high accuracy is often cited as a reason for its continued relevance in genomics.
Applications in Research
Sanger sequencing is predominantly used when researchers need precise results for specific sequences. It is especially useful in clinical diagnostics for genetic disorders, where accuracy is paramount. However, its high cost and low throughput compared to NGS means it is usually limited to specific applications, rather than large-scale sequencing projects.
Whole Genome Sequencing
Whole Genome Sequencing (WGS) is an advanced method that involves sequencing all the genetic material in an organism. This technique can provide comprehensive insights into genetic variants, mutations, and personal genomics.
Process of Whole Genome Sequencing
The process of WGS typically entails using NGS technology to capture and sequence the entire genome. The initial step involves isolating DNA, followed by library preparation, sequencing, and data analysis. WGS has an ability to uncover a significant amount of genetic information, thus aiding various studies from literature to applications in medicine.
Interpretation of Data
Interpreting data from WGS can be quite complex. The sheer volume of data generated requires advanced computational tools and statistical methods to accurately interpret genetic variations. The information gleaned from WGS can have important implications for understanding diseases, especially in personalized medicine.
Targeted Sequencing
Targeted sequencing focuses on specific areas of the genome, making it a more directed approach compared to WGS. This technique is designed for studies aimed at specific genetic regions, which can be critical in certain clinical and research scenarios.
Focus on Specific Regions
By honing in on particular genes or regions of interest, targeted sequencing can yield high-quality data with greater efficiency. This specialized approach can reduce costs and analysis time while providing in-depth insights into relevant genetic factors.
Clinical Applications
Targeted sequencing finds significant use in clinical diagnostics, particularly in identifying genetic conditions and guiding treatment strategies in personalized medicine. This focused methodology brings clarity to complex cases where whole genome sequencing may provide overwhelming data.
Single-Cell Sequencing
Single-cell sequencing allows researchers to analyze the genomes of individual cells, a powerful capability in understanding cellular heterogeneity.
Significance in Research
The significance of single-cell sequencing lies in its ability to reveal differences between individual cells in a sample. This level of detail is crucial for studies in development, cancer research, and more. Single-cell approaches can lead to discoveries that bulk sequencing methods might overlook.
Challenges Faced
However, single-cell sequencing is accompanied by considerable challenges. These include higher costs and the technical complexity of the analysis. Additionally, sample preparation is more intricate and must be done carefully to avoid contamination or loss of data.
Nanopore Sequencing
Nanopore sequencing introduces a novel method of sequencing by measuring changes in electrical current as nucleotides pass through a nanopore.
Mechanism of Action
The mechanism involves passing DNA strands through a nanopore and detecting individual bases based on their specific electrical properties. This allows for real-time sequencing and a simpler process compared to other technologies.
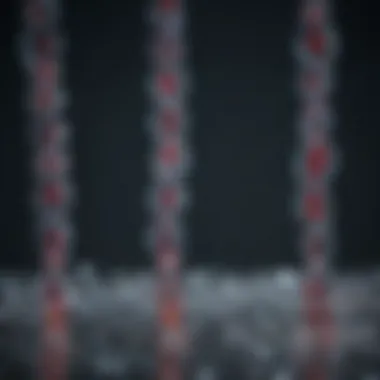
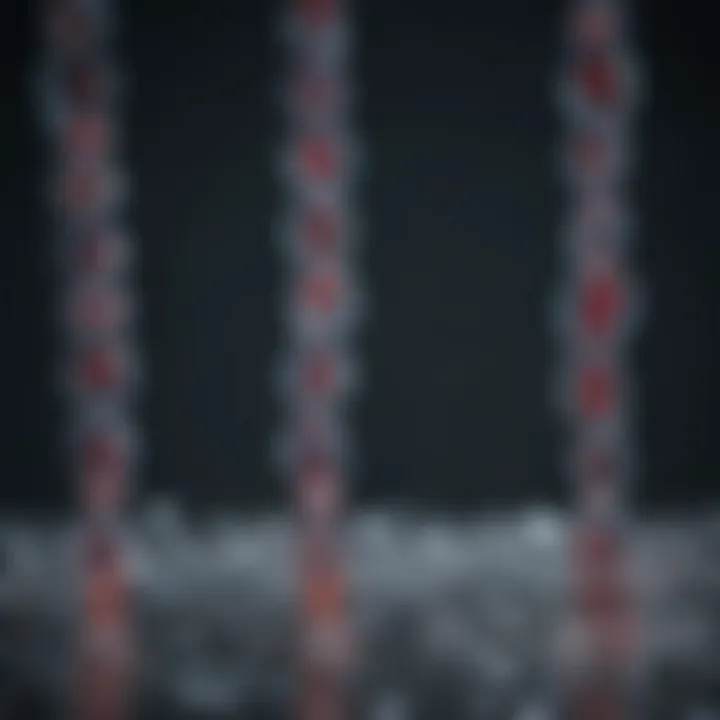
Future Prospects
In the future, nanopore sequencing holds great potential for clinical applications and field work due to its portability and rapid results. Ongoing improvements aim to enhance accuracy and reduce costs, making it an exciting area of research within genomics.
Advances in genome sequencing techniques are shaping the future of genomics, providing deeper insights into genetic data than ever before.
Comparative Analysis of Genome Sequencing Techniques
The comparative analysis of genome sequencing techniques sheds light on the unique advantages and limitations of each method. Understanding these differences is crucial for researchers and practitioners as they select the most appropriate technique for their specific goals. The effectiveness of genome sequencing is influenced by various factors such as cost, speed, accuracy, and the nature of the genetic material being studied. By evaluating these elements comprehensively, stakeholders can make informed decisions that align with their research objectives and resource availability.
Cost-Effectiveness
In the landscape of genome sequencing, cost-effectiveness plays a pivotal role. The financial investment required for sequencing can vary widely depending on the technique employed. Next Generation Sequencing (NGS), for instance, offers higher throughput at a lower per-sample cost compared to Sanger sequencing. This has made NGS the preferred choice for large-scale projects such as population genomics and health initiatives.
However, while initial setup costs for NGS may be substantial, its efficiency in producing a large volume of data can justify this expense over time. On the contrary, traditional methods like Sanger sequencing are generally less costly for small-scale projects but do not scale well for larger operations. Researchers must analyze their budget constraints and project scope to determine the most economical choice.
Additionally, clinical applications often demand a higher accuracy level and prompt results, which can influence the overall cost-effectiveness analysis. Utilizing NGS in direct clinical applications, such as cancer diagnostics, may require supplementary investments in bioinformatics to interpret complex datasets accurately.
Ultimately, an understanding of the financial implications enables researchers to optimize resources while achieving their sequencing goals.
Speed and Throughput
Speed and throughput are critical factors in the efficacy of genome sequencing. Techniques like NGS have revolutionized the pace at which sequencing can be performed, allowing researchers to process thousands of samples simultaneously. This capacity has significant implications for both large cohort studies and urgent clinical diagnostics.
In contrast, Sanger sequencing typically yields results more slowly due to its sequential procedural nature. For projects where rapid results are necessary, such as pathogen identification during an outbreak, the speed of sequencing becomes a deciding factor. The ability to deliver faster results can improve outcomes significantly in clinical settings, potentially affecting treatment options.
Moreover, throughput refers to the number of samples or sequences that can be processed in a given time frame. High-throughput capabilities of NGS enhance productivity, rendering it ideal for large research endeavors. It not only accelerates data generation but also allows for more comprehensive studies involving genomic diversity, evolutionary studies, and extensive population health data analysis.
To summarize, analyzing speed and throughput involves considering both sample size and the urgency of the results. This understanding informs method selection and helps in strategizing the sequencing workflow for optimal efficiency.
Applications of Genome Sequencing
The applications of genome sequencing are vast and transformative, influencing multiple fields such as medicine, research, and conservation. This section delves into specific applications, highlighting their significance and implications.
Personalized Medicine
Role in Treatment Plans
Personalized medicine tailors healthcare to individual characteristics. Genome sequencing plays a vital role in this process by providing detailed genetic information. This information helps doctors create specific treatment plans based on a person’s genetic makeup. An important feature is the ability to identify mutations that may impact drug metabolism.
With the precise data from genome sequencing, clinicians can choose the most effective medication and dosage for each patient. This increases treatment efficacy and minimizes adverse effects. Integrating genetic data into treatment enhances the overall quality of care. However, it can be costly and may not be readily accessible in all healthcare settings. Still, its growing prevalence highlights its potential in modern medical practices.
Ethical Considerations
The ethical implications of genome sequencing in personalized medicine cannot be overlooked. Concerns about privacy and consent arise as personal genetic information is collected and utilized. An essential characteristic is the need for safe handling of sensitive data. It is necessary to prevent misuse or unauthorized access to genetic profiles.
Moreover, the equitable distribution of personalized medicine remains a significant issue. Not everyone has equal access to the benefits of genome sequencing. There is a pressing need for guidelines and policies ensuring equitable practices. Thus, the ethical considerations surrounding personalized medicine are not just theoretical. They are highly relevant to practical implementation in real-world settings.
Disease Diagnosis and Research
Genome sequencing has revolutionized the diagnosis and research of various diseases, enabling scientists and clinicians to understand genetic factors underlying many health conditions.
Genetic Disorders
Genetic disorders often stem from anomalies in an individual's DNA. Genome sequencing aids in identifying these anomalies, allowing for a more accurate diagnosis. By uncovering the genetic basis of these disorders, researchers can further study the mechanisms at play. This knowledge is invaluable for developing targeted therapies or interventions.
The unique feature of detecting mutations gives this application significant advantages. Early diagnosis can lead to timely interventions and better outcomes for patients. However, the process is intricate, requiring advanced expertise and resources. Genetic counseling also becomes necessary to guide families and patients through understanding the implications of the findings, ensuring that the benefits outweigh the emotional burden that may arise from such knowledge.
Infectious Diseases
Infectious disease management has improved with the advent of genome sequencing. This method allows for precise identification of pathogens, which is crucial for accurate diagnosis and treatment. Understanding the genetic characteristics of infections informs decisions about treatment options.
Additionally, genome sequencing enables tracking of pathogen evolution. This information is useful in outbreak management, as it helps in determining transmission routes. The rapid identification of strains can lead to improved public health responses. However, there is a potential downside related to resource investment needed for such diagnostics. Despite this, the benefits in controlling and understanding infectious diseases are significant, marking genome sequencing as a pivotal tool in modern medicine.
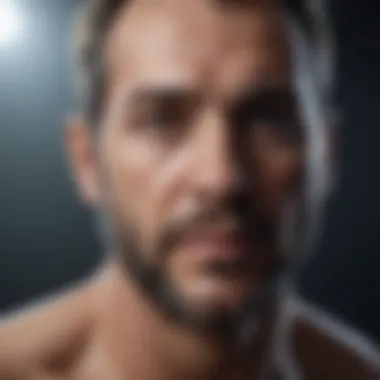
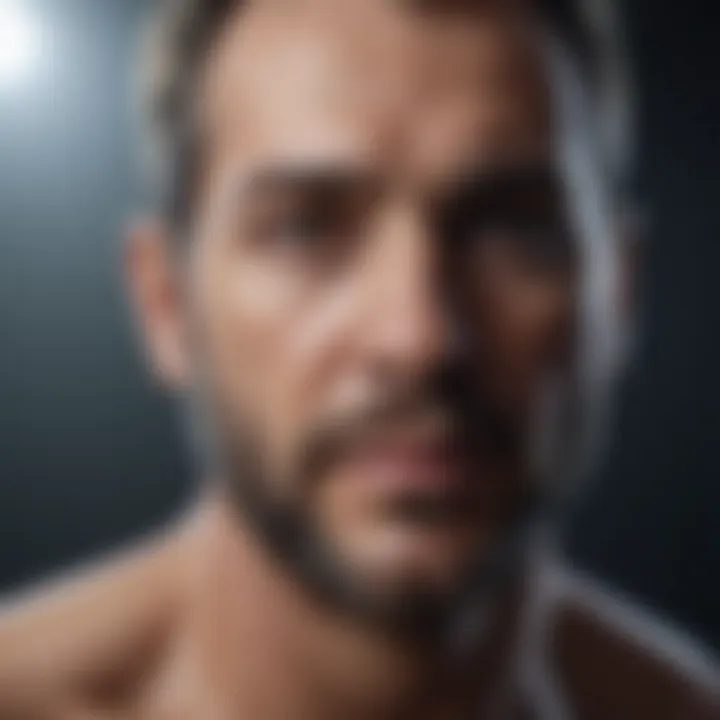
Evolutionary Biology and Conservation
In evolutionary biology, genome sequencing provides insights into the genetic makeup of various species. This information helps scientists understand evolutionary relationships and biodiversity. Moreover, in conservation efforts, sequencing can assist in preserving endangered species.
By analyzing genomic data, conservationists can identify genetic diversity within populations. This information is important for developing effective conservation strategies. It can also assist in monitoring species adaptation to climate change or other environmental pressures. However, the application is still developing, and further research is necessary to maximize its benefits.
Ethical and Social Implications
The realm of genome sequencing extends beyond scientific inquiry into significant ethical and social considerations. As technologies evolve, they prompt discussions about privacy, access to information, and societal implications. Recognizing these implications is paramount for researchers, healthcare providers, and policymakers alike. This section explores these aspects including data privacy and the broader societal impact.
Data Privacy Concerns
Data privacy is a critical concern in genome sequencing. Individuals whose genomes are sequenced generate sensitive information about their genetic makeup. This knowledge can reveal not just predispositions to certain diseases, but also information about ancestry and even potential future health risks. Because of this sensitivity, it is essential to establish robust frameworks to protect genomic data.
Legislation, such as the Genetic Information Nondiscrimination Act (GINA) in the United States, aims to protect individuals from discrimination based on genetic information. However, gaps remain in legislation when it comes to data sharing and privacy in many jurisdictions.
To address these concerns, institutions must adopt measures like:
- Informed consent practices that clearly inform participants about data usage.
- Anonymization techniques to reduce the risk of identification from genetic materials.
- Strong data governance policies that dictate how genetic information is stored, used, and shared.
Implementing these processes can help mitigate the risks associated with genetic data while promoting public trust in genomic research.
Impact on Society
The societal impact of genome sequencing cannot be overstated. By better understanding genetic predispositions, personalized medicine emerges as a revolutionary application of this technology. Treatments tailored to an individual's genetic profile can enhance patient outcomes significantly. Yet, this also raises questions about equity in healthcare access.
As genomic testing becomes more integral to the healthcare system, disparities may arise based on socioeconomic status. If only a segment of the population can access personalized treatments, societal divides could widen. Thus, addressing healthcare equity remains a pressing issue.
Moreover, as genomic information becomes more prevalent, discussions surrounding its use in non-medical contexts arise. Employers, insurers, and even governments may seek access to genetic information, which could lead to discrimination or stigmatization.
In summary, while genome sequencing technology offers benefits, it also necessitates careful consideration of ethical and social factors. Informed consent, data security, healthcare equity, and societal attitudes toward genetic information are all critical areas requiring ongoing dialogue and robust policies to guide future developments.
"As we advance in our understanding of the genome, the ethical landscape will become increasingly complex. It is imperative that we navigate these challenges with foresight and integrity." - Unknown
Future Directions in Genome Sequencing
The landscape of genome sequencing is rapidly evolving. Future directions in genome sequencing hold immense potential for both scientific advancement and practical applications. This section discusses innovations on the horizon and the potential integrations with artificial intelligence, addressing their implications on research, healthcare, and societal impact.
Innovations on the Horizon
Innovations in genome sequencing are allowing for more precise and efficient methods. The advent of improved sequencing technologies is helping scientists to decode genetic information faster and at a lower cost. Notable trends include:
- Miniaturization of Sequencing Platforms: Smaller devices are emerging, making sequencing more accessible in diverse settings, including remote locations.
- Increasing Throughput: Technologies like high-throughput sequencing continue to advance, enabling the analysis of numerous samples simultaneously. This scalability is critical for studies that require a vast amount of data.
- Long-Read Sequencing Techniques: Techniques such as PacBio or Oxford Nanopore are being developed, with a focus on reading longer sequences of DNA. This capability allows for a better comprehension of complex genome regions.
- Real-Time Sequencing: The next generation of sequencing may allow for immediate data analysis. This could revolutionize fields such as diagnostics, where timely information is crucial.
These advancements promise not just enhanced efficiency, but also the ability to uncover more about genetic variations and their implications in health and disease.
Potential for Integration with AI
The integration of artificial intelligence in genome sequencing could redefine the entire field. AI technologies can process vast numbers of genomic data quickly, significantly aiding interpretation. Several benefits include:
- Data Analysis: Machine learning algorithms can identify patterns in genomic data that may not be evident to researchers. This capability could assist in predicting disease risks based on genetic information.
- Automation: The automation of data processing and analysis through AI can reduce the time required for genome sequencing projects, increasing overall productivity.
- Personalization: AI can help develop tailored approaches based on individual genomic information, enhancing personalized medicine.
Experts in the field are optimistic about AI's role in parsing complex datasets, helping refine diagnostic frameworks, and enhancing our understanding of genetic relatedness over time.
"The future of genome sequencing lies in technology that not only sequences DNA faster but interprets it intelligently."
As these innovations and integrations unfold, the implications for personalized healthcare and biological research will be profound, shaping a new era in genomic science.
Culmination
Genome sequencing has transformed the landscape of biology and medicine. It allows us to decode the genetic information contained within DNA, providing insights that were previously unattainable. The advancements in sequencing technologies have made it more accessible and cost-effective, paving the way for increased applications in research, diagnostics, and personalized medicine.
Summation of Key Points
- Diverse Techniques: Various methods like Next Generation Sequencing and Sanger Sequencing offer unique advantages and challenges. Understanding these techniques is crucial for researchers and clinicians alike.
- Applications: Genome sequencing plays a pivotal role in personalized medicine, enabling tailored treatment plans based on an individual’s genetic makeup. It is also essential in studying genetic disorders and infectious diseases.
- Ethical Considerations: As the use of genome sequencing grows, ethical implications surrounding data privacy and societal impact must be addressed. The benefits must be weighed against potential risks to individuals and communities.
- Future Directions: Innovations in technology, particularly those integrating artificial intelligence, promise to enhance the efficiency and accuracy of genome sequencing, further expanding its applications.
Final Thoughts on the Future of Genome Sequencing
The future of genome sequencing is bright yet complex. As technologies evolve, they bring both opportunities and challenges. The potential integration of AI could revolutionize analysis and interpretation of genomic data, leading to even more personalized and effective healthcare solutions. However, it is essential to remain vigilant regarding ethical and social implications as these technologies become more ubiquitous. The journey of understanding the genome is ongoing, and its implications will resonate across many fields. The next steps taken in this field will determine the balance between innovative breakthroughs and the ethical frameworks needed to support them.