Comprehensive Guide to the GFP Trap Protocol
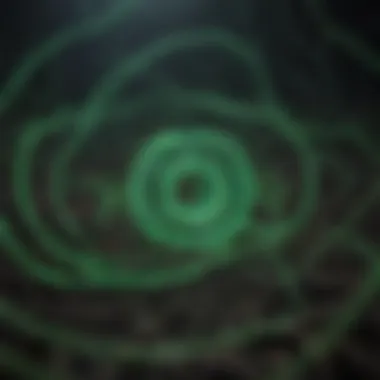
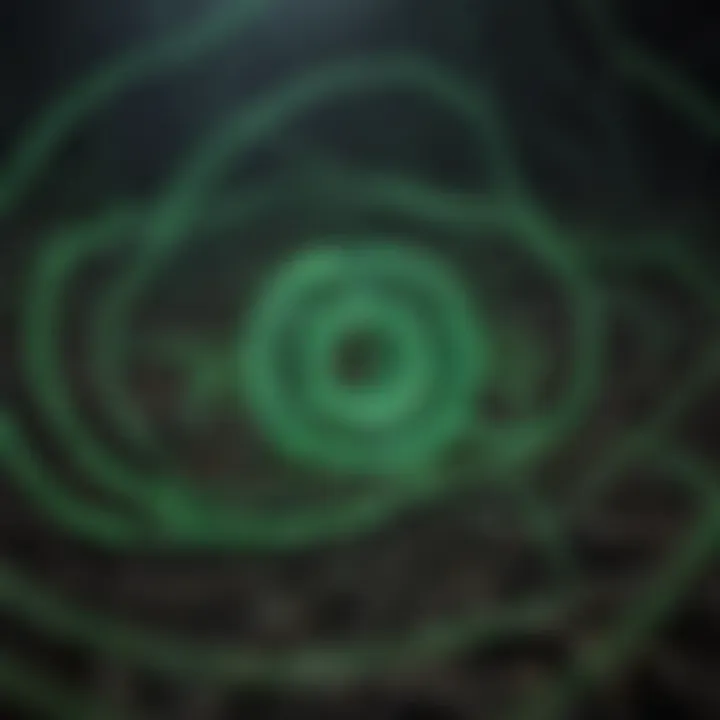
Intro
The GFP trap protocol stands as a pivotal technique in molecular biology. Its ability to track protein interactions and localizations within live cells opens a myriad of avenues for research. The insights gained through this approach significantly broaden our understanding of cellular processes. In this article, we strive to detail every step involved in the GFP trap protocol, from foundational concepts to practical applications and potential limitations.
Research Overview
Summary of Key Findings
The GFP trap protocol has demonstrated several key findings that enhance our knowledge in protein research. It allows for the visualization of protein interactions, facilitating real-time studies within living cells. This technique has been instrumental in revealing the dynamics of protein engagement in critical cellular processes, including signaling pathways and structural organization.
Importance of the Research
Understanding protein interactions is crucial for various fields, including biochemistry, genetics, and cell biology. The GFP trap protocol provides a method to observe these interactions in a native context, crucial for interpreting biological functions accurately. As researchers strive for precision and depth in protein studies, tools like the GFP trap protocol become indispensable.
Methodology
Study Design
A well-structured study design is essential for the implementation of the GFP trap protocol. Typically, this involves selecting the appropriate cell line and ensuring that the cells are genetically modified to express the desired GFP-tagged proteins. The design should include control experiments to validate results and minimize potential confounding factors.
Data Collection Techniques
Data collection in the context of the GFP trap protocol comprises several strategies.
- Fluorescence Microscopy: Utilizing high-resolution fluorescence microscopy is paramount. It enables researchers to visualize GFP-tagged proteins in real-time, providing critical insights into their functional dynamics.
- Image Analysis Software: Post-acquisition analysis generally involves software tools that assist in quantifying fluorescence intensity, protein localization, and interaction kinetics, crucial for accurate interpretation of results.
"The GFP trap protocol not only reveals static images of proteins but allows observation of their dynamic interactions in living cells."
By merging these methodologies, researchers can generate robust and reproducible data that can drive forward our understanding of cellular biology.
End
In sum, the GFP trap protocol is a powerful technique that blends simplicity with sophistication, allowing for a direct observation of protein behavior in living organisms. By exploring its principles and methodologies, researchers can enhance their experimental approaches, leading to groundbreaking discoveries.
This article aims to provide clarity and guidance, helping students, educators, and professionals navigate the complexities of this technique and its implementation.
Prelims to GFP Trap Protocol
The GFP Trap Protocol stands as a cornerstone technique in molecular biology, primarily utilized for examining protein dynamics and interactions within live cells. This protocol leverages the unique properties of green fluorescent protein (GFP) to facilitate direct observation of proteins in their native environments. Understanding the GFP Trap Protocol is essential for researchers aiming to dissect complex biological processes, as it provides insights into protein localization, interaction networks, and cellular behavior under various conditions.
Overview of GFP Technology
GFP technology harnesses the bioluminescent property of the GFP, originally derived from jellyfish. Researchers have adopted this powerful visualization tool for tagging specific proteins within the cells. The beauty of GFP lies in its fluorescent nature; when exposed to the appropriate wavelength of light, it emits a vivid green glow. This capability allows scientists to follow individual proteins in real time, opening avenues for numerous experiments in cell biology.
By integrating GFP with proteins of interest, one can visualize spatial and temporal dynamics that would otherwise remain invisible. This tagging does not traditionally interfere with the protein's function, making it a favored method among researchers.
Significance in Molecular Biology
The significance of the GFP Trap Protocol extends across various dimensions of molecular biology. Primarily, it enables a deep understanding of protein localization, which is crucial for appreciating cellular function and processes. By knowing where proteins reside within the cell, researchers can ascertain their roles in different cellular pathways and reveal underlying mechanisms of disease or abnormal cellular behavior.
Furthermore, the GFP Trap Protocol allows for the study of protein-protein interactions, thereby aiding in the construction of interaction networks. These interactions have vital implications for understanding signaling pathways and developmental processes.
"The GFP trap protocol serves as a bridge between biochemistry and cell biology, offering a holistic perspective on protein functionality."
Additionally, the protocol has key implications in therapeutic research, particularly targeting diseases where protein malfunction is a hallmark. By elucidating the roles of specific proteins in various pathways, scientists can develop targeted treatments, enhancing the precision of therapeutic approaches.
Principles of GFP Trap Protocol
The principles underlying the GFP trap protocol form the foundation for its application in molecular biology. The ability to observe and study protein behavior in live cells is crucial not only for understanding cellular functions but also for uncovering the underlying mechanisms of various biological processes. The GFP trap utilizes the distinctive fluorescent properties of green fluorescent protein (GFP) to tag proteins of interest, enabling researchers to visualize interactions and localization in real time. This section elaborates on the essential components and benefits of the GFP trap protocol, as well as key considerations for its successful implementation.
Fluorescence and Protein Tagging
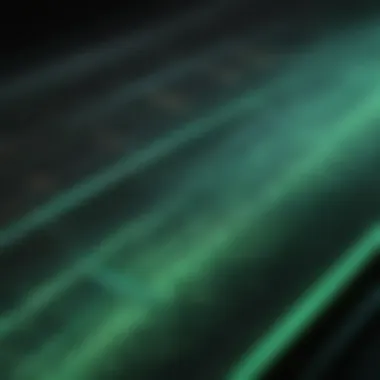
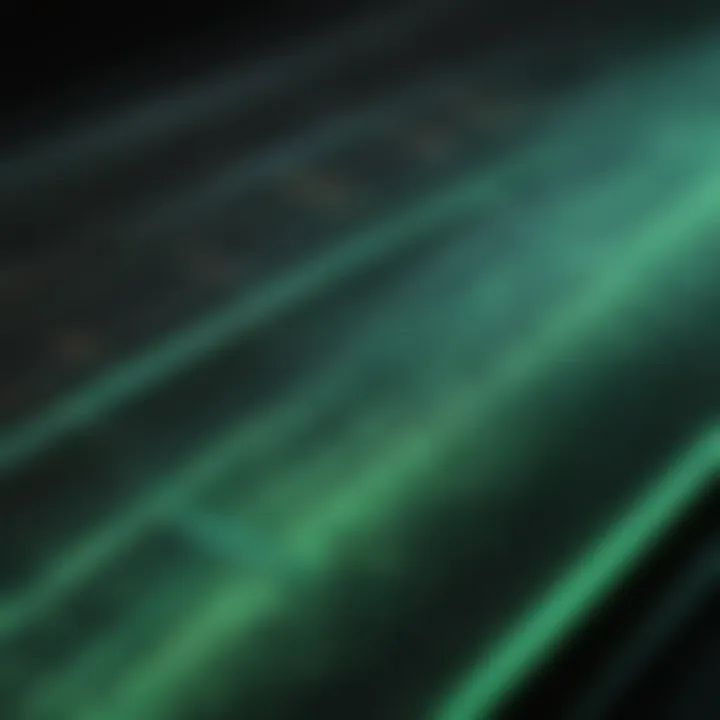
Fluorescence is at the heart of the GFP trap protocol. Fluorescence occurs when a molecule absorbs light at a specific wavelength and re-emits it at a longer wavelength. GFP, originally derived from the jellyfish Aequorea victoria, has become a widely acclaimed marker in cellular biology due to its minimal toxicity and ease of gene fusion.
When a protein is tagged with GFP, it becomes fluorescent. This allows scientists to track the protein inside cells without disrupting cellular processes. The non-invasive nature of this tagging is a key benefit. Here are some advantages of using GFP in protein studies:
- Real-time visualization: Researchers can observe protein movement and interactions as they happen.
- Stability: GFP typically remains stable under various experimental conditions, making it suitable for long-term studies.
- Compatibility with various techniques: GFP can be combined with other biophysical methods, allowing for a multifaceted approach to study protein dynamics.
However, there are also important considerations. The size of the GFP tag can potentially interfere with protein function or localization. Careful experimental design is necessary to ensure that tagging does not alter the native behavior of the protein. This highlights the importance of validating the functionality of tagged proteins in the context of biological roles.
Binding Mechanisms of the GFP Trap
The GFP trap works by applying specific binding mechanisms that facilitate the capture of tagged proteins. The protocol often employs a scaffold or resin designed to bind the fluorescently labeled proteins effectively. Understanding these binding interactions is pivotal for optimizing the protocol.
The GFP trap generally relies on affinity chromatography, utilizing a capture system that specifically interacts with the GFP tag. This may involve:
- Specific affinity ligands that recognize GFP, ensuring that only desired protein complexes are isolated.
- Elution strategies to recover bound proteins while minimizing loss or degradation.
For efficient binding, several factors must be controlled:
- pH and ionic strength of the buffer solutions play a crucial role in the interaction affinity.
- Temperature conditions may be optimized to enhance binding efficiency without sacrificing protein integrity.
Maximizing the success of the GFP trap protocol requires a thorough understanding of these binding mechanisms and careful control over the experimental conditions.
Understanding the principles of the GFP trap protocol enables researchers to effectively explore protein behaviors and interactions, broadening the horizon for molecular biology research.
Materials Required
Understanding the materials required for the GFP Trap Protocol is crucial for researchers intending to implement this technique effectively. The selection of appropriate equipment and reagents is pivotal not only for the success of the experiment but also for obtaining reliable and reproducible results. This section details the essential components necessary to conduct the GFP Trap Protocol, emphasizing their roles and importance in obtaining high-quality datasets.
Essential Equipment
Several pieces of equipment are fundamental to executing the GFP Trap Protocol. Each item listed below fulfills a specific function that contributes to the overall efficacy of the experiment.
- Fluorescence Microscope: This instrument is vital for visualizing GFP-tagged proteins in live cells. It must be capable of providing the necessary excitation wavelengths and capturing high-resolution images of fluorescent signals.
- Cell Culture Incubator: Maintaining optimal growth conditions for cells is essential. An incubator must provide the right temperature, humidity, and CO2 levels, which allows for the maintenance of live cell cultures during the experiment.
- Centrifuge: A centrifuge is important for separating cellular components during sample preparation, in particular, isolating proteins from cell lysates. Choosing a model with appropriate speed and capacity is necessary for efficient sample processing.
- Pipettes and Tips: Precision in liquid handling is crucial for obtaining accurate concentrations of reagents. Using high-quality pipettes and corresponding tips minimizes variability in experimental conditions.
- Spectrophotometer: This piece of equipment can assist in quantifying protein concentrations and assessing the purity of the samples, ensuring that they are suitable for analysis.
Each piece of equipment plays a critical role in the preparation and execution of experiments. Choosing the right tools ensures researchers can focus on interpreting results rather than troubleshooting technical issues.
Reagents and Consumables
In addition to equipment, specific reagents and consumables are also necessary for the GFP Trap Protocol. These materials support the experimental design and help achieve desired outcomes.
- GFP-Binding Protein: A crucial component, this reagent is responsible for binding to the GFP-tagged proteins. It is essential to select a protein with high specificity and affinity to the GFP to ensure effective trapping.
- Lysis Buffer: This reagent is used to lyse cells and release the target proteins into solution. The composition of the lysis buffer must be carefully chosen based on the target proteins’ solubility and stability.
- Washing Buffers: After trapping proteins, washing buffers help to remove unbound or non-specific interactions. The formulation of these buffers can affect signal quality, thus it’s important to optimize their conditions.
- Blocking Agents: These are necessary to prevent non-specific binding during the experiment. Using appropriate blocking agents can enhance the specificity and sensitivity of detection.
- Detection Antibodies: For analysis, employing specific antibodies that can recognize the target proteins is fundamental. This specificity will ensure that the signals detected are relevant to the intended biological interactions.
In summary, carefully selecting and preparing the materials required for the GFP Trap Protocol enhances the chances of successful experimental outcomes. Considering both equipment and reagents ensures that researchers can effectively analyze protein interactions and localizations with clarity and precision.
"The success of the GFP Trap Protocol relies heavily on the quality of materials used, thus meticulous planning is key."
By keeping these materials in mind, researchers can set the stage for fruitful experimentation in molecular biology.
Step-by-Step Protocol
The Step-by-Step Protocol section serves as a practical foundation for implementing the GFP trap protocol. Having a clear protocol is essential for molecular biology studies, as it allows researchers to reproduce results and minimize error. This section will outline three critical components: sample preparation, setting up the GFP trap, and data collection and analysis. Each step elucidates considerations necessary for the effective application of the protocol, ensuring that users achieve reliable and valid findings.
Preparation of Samples
Sample preparation is pivotal in the GFP trap protocol. Properly prepared samples lay the groundwork for successful protein interactions and localization studies. The first step involves cell selection and transfection with a GFP-tagged protein of interest. Utilize techniques such as lipofection or electroporation for efficient transfection. Ensure that the transfection efficiency is high, as this influences the abundance of the expressed protein within cells.
Note: It is critical to include controls during sample preparation. Negative controls can highlight background signals that may confuse data interpretation.
Next, the cells should be harvested and washed gently to remove any unincorporated GFP tags. Adding a buffer solution such as phosphate-buffered saline can help maintain physiological relevance. Post-wash, the cellular pellet should be lysed using a suitable lysis buffer to release proteins while preserving their functional states. This step is crucial, as improper lysis can lead to degradation or alteration of the proteins being studied.
Setting Up the GFP Trap
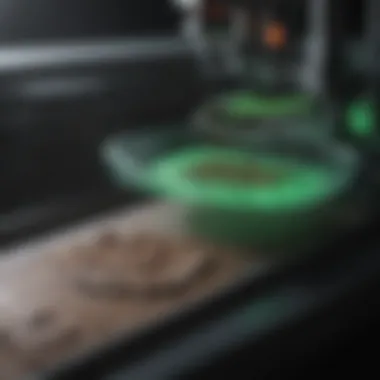
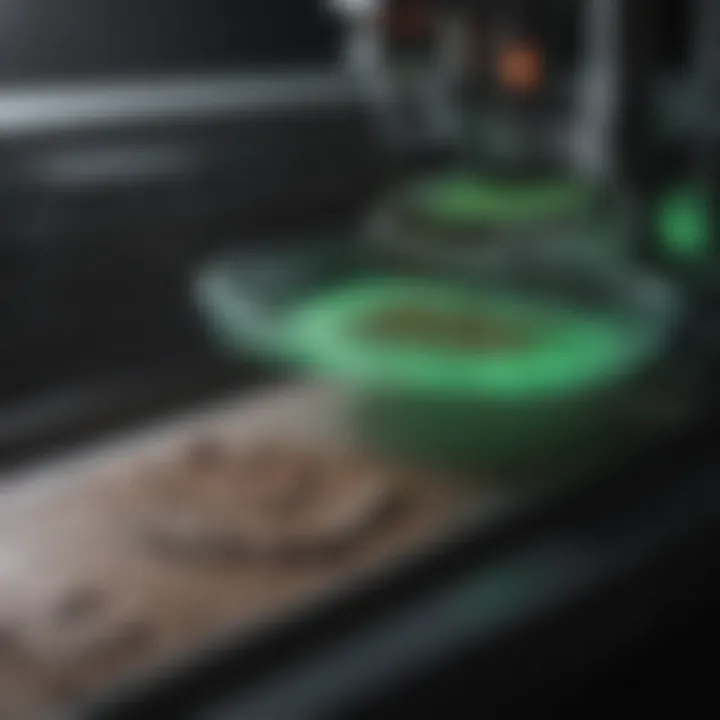
Once the samples are ready, the next phase consists of setting up the GFP trap. The GFP trap apparatus generally includes specialized affinity reagents that specifically bind to GFP. In this setup, selecting the right affinity matrix is important. Common choices may include antibodies against GFP, which can be immobilized on a column for purification.
The process begins by mixing the lysed samples with the affinity matrix, allowing sufficient incubation time for binding. Typically, this duration may vary based on the specific reagents utilized and the concentration of the GFP tag in the sample. During this stage, it is important to maintain optimal temperature and gentle mixing to promote efficient binding without inducing protein denaturation.
After the binding phase, wash the matrix to remove non-specifically bound proteins. This step is crucial to enhance the purity of the samples. Depending on the specific needs of the experiment, elution buffer can then be applied to recover the GFP-tagged proteins for downstream applications.
Data Collection and Analysis
The final part of the protocol entails data collection and analysis. This segment is critical; it translates experimental data into meaningful results. Collect fluorescence data using appropriate imaging equipment capable of detecting GFP signals. Confocal microscopy and flow cytometry are frequently employed techniques due to their high sensitivity and specificity.
Once the imaging or flow results are gathered, data analysis comes into play. Software such as ImageJ or Fiji can facilitate quantitative analysis of fluorescence intensity. It’s beneficial to measure background fluorescence and account for any that may skew results. Additionally, statistical analysis should be conducted to determine the significance of observed interactions.
In summation, the Step-by-Step Protocol segment elaborates on critical phases of the GFP trap protocol, enabling researchers to effectively study protein interactions. Ensuring robust preparation, careful setup, and thorough analysis will underpin successful syntheses in molecular biology.
Optimization of the GFP Trap Protocol
Optimization of the GFP trap protocol is crucial to enhance the quality and reliability of results in protein studies. As the protocol can involve various stages, fine-tuning can help minimize errors and improve overall efficiency. Specific elements to consider include improving sample preparation, adjusting incubation times, and modifying fluorescence settings. Typically, small adjustments in these areas can lead to significant improvements in data quality. The benefits of successfully optimizing the protocol are manifold: enhanced signal-to-noise ratios, clearer visualizations of protein interactions, and more reproducible results. These aspects are vital for researchers aiming to derive meaningful conclusions from their experiments.
Tuning Experimental Conditions
Tuning experimental conditions involves adjusting parameters such as temperature, pH, and the concentration of reagents. These factors significantly affect the binding efficiency of the GFP trap. For instance, small variations in temperature can influence protein stability and affinity for binding partners. Conducting preliminary experiments can help determine the ideal conditions for specific samples.
It is also important to consider the duration for which the samples are incubated with the GFP trap components. Longer incubation times may result in increased binding; however, they can also lead to non-specific binding, which must be managed carefully. Researchers often benefit from creating a matrix of different conditions to assess their effects on GFP performance. This way, it is easier to identify the most promising conditions for optimization.
Improving Signal Detection
Improving signal detection is another critical aspect of protocol optimization. It involves enhancing the visibility of the GFP signal against the background noise. One effective strategy is to optimize the illumination source. Using lasers with appropriate wavelengths can enhance the fluorescence signal.
Another consideration is the choice of filters, which can selectively allow specific wavelengths associated with GFP while blocking others. Additionally, employing high-quality optics will ensure that the collected images maintain clarity and contrast.
Finally, calibration of imaging systems should not be overlooked. Regular checks ensure that instruments function within expected parameters and help in mitigating any systematic flaws that might distort results. By systematically addressing these components, researchers can effectively improve their GFP trap outcomes, yielding more informative and high-quality data.
"Optimization is not just about achieving immediate results; it is about paving the way for reliable and meaningful discoveries in research."
Engagement in optimization practices can greatly enhance the robustness of findings in studies. It subsequently allows practitioners to explore novel avenues in protein interaction and localization research.
Applications of the GFP Trap Protocol
The GFP trap protocol serves a pivotal role in molecular biology, particularly within the contexts of protein interactions and cellular localization. By applying this technique, scientists can gain invaluable insights into the dynamic behaviors and functions of proteins in their natural environments. The versatility of this protocol allows it to be tailored for diverse research needs, offering both depth and breadth in its applications.
Benefits of Using GFP Trap Protocol
- Real-time Analysis: With the GFP trap protocol, researchers can observe protein interactions as they happen within living cells. This feature is crucial for understanding the temporal dynamics of biological processes.
- Minimized Artifacts: By utilizing this method, investigators can reduce potential artifacts often associated with traditional assays. This leads to more reliable data reflecting true biological phenomena.
- Ease of Use: The GFP tag allows researchers to easily track proteins throughout various cellular compartments without significant disruption to the cell's normal functions.
The GFP trap protocol is not just a technical procedure; it is a lens through which biologists can explore the intricate web of life at the molecular level.
Protein Interaction Studies
In protein interaction studies, the GFP trap protocol can elucidate how proteins communicate and function together within complex cellular networks. It helps in visualizing co-localization of proteins, providing essential clues about functional partnerships. The fluorescent tagging permits researchers to monitor direct interactions, often involving protein-protein interactions (PPIs) which are crucial for virtually all biological processes.
- Analyzing Complex Formation: By observing the fluorescence emitted from tagged proteins, scientists can detect whether proteins of interest form complexes. This analysis can guide hypotheses regarding their roles in signaling pathways.
- Quantifying Interactions: This method allows quantification of the interactions through intensity measurements and decay rates of the fluorescence. Such quantification can inform the strength and significance of the interactions observed.
- Dynamic Interactions: Unlike static experimental techniques, the GFP trap protocol provides insights into transient interactions, offering a real-time view of how proteins dynamically associate and dissociate within cells.
Cellular Localization Investigations
The GFP trap protocol is also instrumental in determining the cellular localization of proteins, which is fundamental for understanding their biological roles. Localization can significantly influence a protein's functional capacities, and improper localization could indicate pathophysiological conditions.
- Tracking Developmental Changes: Researchers can use the GFP trap to observe how proteins are distributed during different stages of cellular development or in response to external stimuli.
- Identifying Subcellular Structures: By visualizing where a particular protein resides within the cell, scientists can infer its involvement in specific cellular functions or pathways. For instance, proteins targeted to mitochondria or the nucleus may perform very different roles.
- Correlating Localization with Function: Understanding where a protein localizes can help elucidate its function and interaction dynamics. For example, proteins that localize to the membrane are likely involved in signaling, while those in the cytosol may play roles in metabolic pathways.
By applying these approaches through the GFP trap protocol, researchers can uncover intricate details about protein behavior and localization that contribute to a broader understanding of cellular dynamics in health and disease.
Limitations and Challenges
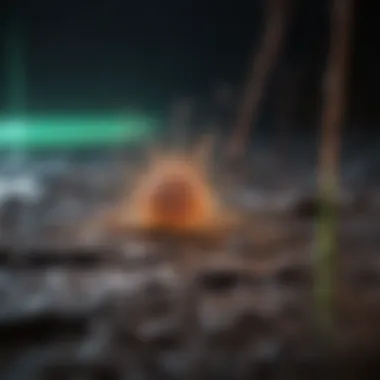
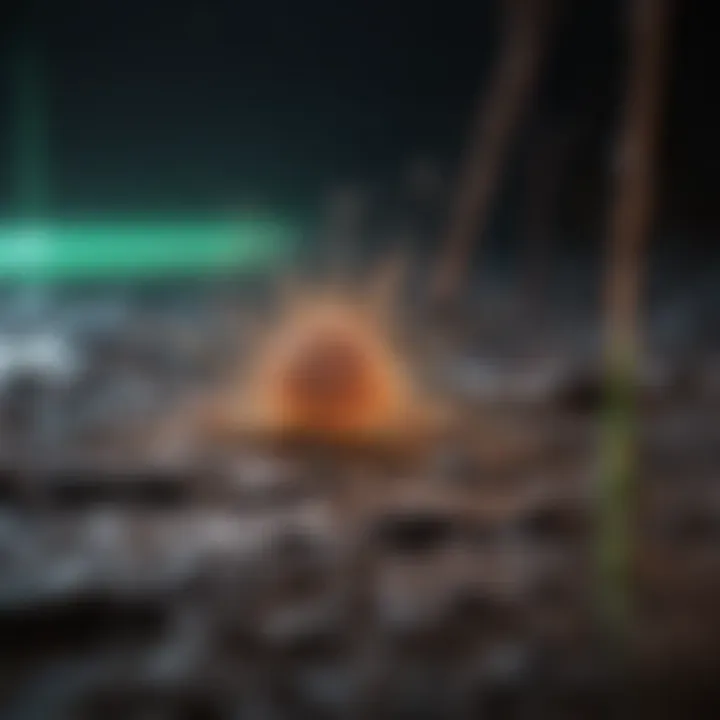
The GFP trap protocol, while powerful and widely utilized, is not without its limitations and challenges. Understanding these issues is crucial for researchers and practitioners using this technique, as it directly influences the reliability and validity of their results. Highlighting these elements allows for more informed decision-making when applying the GFP trap protocol in various studies.
Potential Technical Issues
One common technical issue when using the GFP trap protocol is the variability in expression levels of the tagged proteins. Overexpression can lead to artifacts in data interpretation, while underexpression can limit detection sensitivity. Inadequate tagging may also influence the protein's functionality or localization, resulting in misleading conclusions. Thus, determining the optimal conditions for expressing the tagged protein is essential.
Another technical problem stems from the potential for non-specific binding. The GFP trap relies on targeted binding interactions, but contaminants can interfere with these processes. This misidentification complicates the understanding of genuine protein interactions. To mitigate these risks, it is important to include proper controls and running parallel experiments. Regular calibration of equipment is also necessary to maintain accuracy in fluorescence measurements.
Finally, the photostability of GFP can significantly impact results. High-intensity excitation light can photobleach the protein, leading to loss of signal and affecting quantitative analyses. Employing imaging techniques that minimize exposure or utilizing more stable variants of GFP can reduce this risk.
"Understanding and addressing the potential technical issues inherent in the GFP trap protocol is vital for obtaining reliable data in protein research."
Biological Considerations
Biological considerations also represent important challenges when implementing the GFP trap protocol. The biological context of the study can influence protein behavior and interactions. Cellular conditions, including pH, temperature, and ionic strength, can significantly alter protein folding and stability, leading to different results in varying environments. Therefore, it is essential to consider and control these variables as much as possible.
Another issue is the potential for cellular stress induced by the tagging process. Introducing a GFP tag can affect cell viability and function. Researchers must evaluate how the presence of the GFP tag may influence the physiological state of the cells. Performing a thorough assessment of cell health post-tagging can provide insights into whether observed results are indeed reflective of biological interactions.
Lastly, the selection of appropriate cell lines for experiments is critical. Different cells can present heterogeneous responses to the GFP tag, affecting the generalizability of findings. Using widely recognized and validated models can help to ensure that results are applicable across biological contexts.
Understanding limitations and challenges is essential in harnessing the full potential of the GFP trap protocol. By being aware of these technical and biological considerations, researchers can take proactive steps to improve their experimental design and data interpretation.
Future Directions in GFP Research
The field of GFP research is dynamic, constantly evolving with new technological advances and discoveries. Understanding future directions is crucial for researchers and practitioners who aim to utilize the GFP trap protocol more effectively. Addressing the limitations and challenges faced today opens the door for innovations and applications that will enhance molecular biology.
Innovations in Protein Tagging
Novel approaches to protein tagging are being developed to expand the utility of GFP. Recent innovations include engineered variants of GFP that exhibit improved brightness and photostability. These enhancements offer better visualization of proteins in live cells without compromising cell health.
One specific area of advancement involves the use of split-GFP technology. This technique allows proteins to be tagged in a way that is less disruptive to their natural function. Split-GFP tags can be introduced separately into different protein partners, and when they interact, a fluorescent signal is produced. This opens pathways for finer resolution studies in protein interactions.
Furthermore, advancements in optogenetics—a field that employs light to control cellular processes—are incorporating GFP tagging for real-time monitoring of protein dynamics inside cells. By harnessing the principles of synthetic biology, researchers can also design proteins that release fluorescence only when certain biological conditions are met, thus providing insights into cellular pathways.
"Continued innovations in protein tagging not only expand the capabilities of GFP technology but also push the boundaries of our understanding in cellular dynamics."
Expanding Applications Across Disciplines
The applications of the GFP trap protocol are not confined to molecular biology alone. The principles of protein tagging are being adapted for use in diverse fields such as neuroscience, pharmacology, and cancer research.
In neuroscience, GFP is often used to label neurons and observe their activity in real time. By combining the GFP trap protocol with advanced imaging technologies such as two-photon microscopy, researchers can analyze complex neural circuits, leading to insights about brain function and development.
Pharmacology is incorporating GFP tagging to evaluate drug interactions at the cellular level. Using the GFP trap, researchers can track how newly developed pharmacological agents affect specific protein functions in live cells, allowing for quicker assessments of drug efficacy and safety.
Cancer research benefits as well through the exploitation of the GFP trap protocol in imaging tumor microenvironments. Understanding protein interactions within tumors can identify potential therapeutic targets and contribute to the development of more effective treatments.
As these applications expand, areas like environmental science and biotechnology are also starting to utilize GFP technology for monitoring pollutants or enhancing crop traits, demonstrating the versatility and scope of the GFP trap protocol in contemporary research.
Epilogue
The conclusion serves as a critical summary of all the insights gathered from the GFP Trap Protocol. Notably, it consolidates the knowledge accumulated in earlier sections and emphasizes the implications of the findings. Understanding this conclusion aids researchers in appreciating the multifaceted applications of this technique in molecular biology.
Summary of Key Insights
The GFP Trap Protocol successfully enables the examination of protein interactions in live cells with accuracy and efficiency. Some essential insights include:
- Fluorescent Protein Technology: The efficacy of green fluorescent protein as a marker for live imaging is significant. This facilitates the observation of dynamic cellular processes.
- Binding Mechanisms: Understanding how proteins adhere to GFP tags remains crucial for accurate analysis. It ensures the validity of experimental results.
- Optimized Methodologies: Tailoring experimental conditions can mitigate common technical challenges. Results can be consistent and reliable when the procedure is fine-tuned adequately.
Overall, the combination of these findings forms a solid foundation for both practical application and further exploration in the field of molecular biology.
Importance of Continued Research
Continued research into the GFP Trap Protocol is paramount for several reasons. As science progresses, enhancing the methodology can lead to more precise data and innovative uses.
- Advancing Techniques: Innovations in protein tagging are possible with ongoing studies. Improved tagging methods could yield enhanced detail in cellular imaging and protein interactions.
- Broader Applications: The versatility of GFP technology allows for exploration in various biological disciplines. To enhance understanding of cellular processes, scientists can apply this technology to different research themes.
- Interdisciplinary Collaborations: Cooperation among biologists, chemists, and data scientists can drive progress. Sharing findings and techniques enriches all fields involved and expands applications.
Ultimately, continued exploration and innovation within the GFP Trap Protocol open new avenues for research, fostering a deeper understanding of protein dynamics in living systems.