The HIV Genome: Structure, Function, and Implications
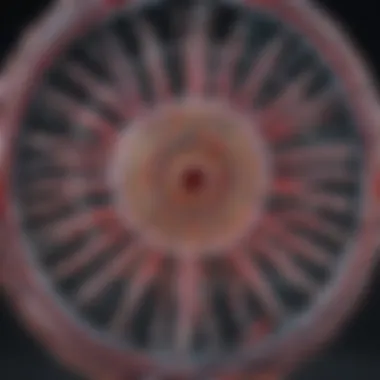
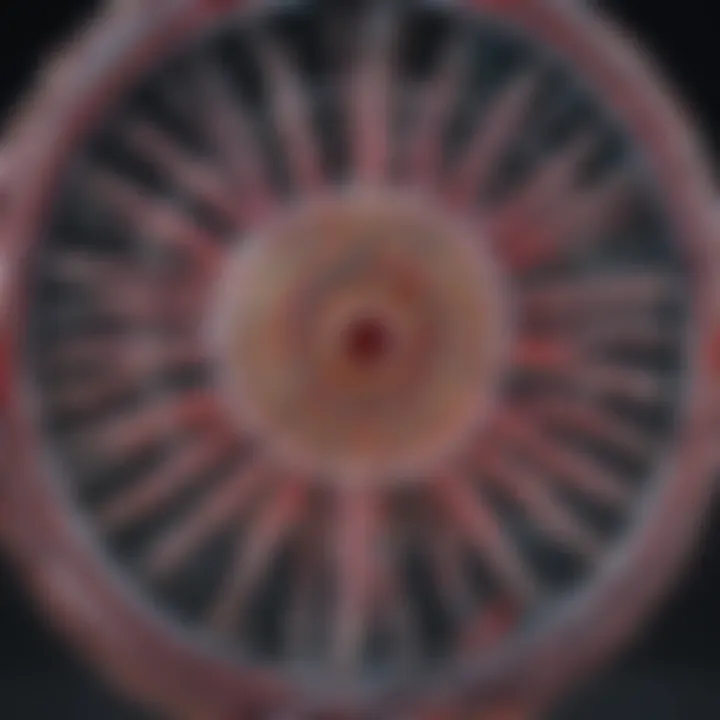
Intro
HIV, or human immunodeficiency virus, presents a global health challenge. Understanding its genome is critical for advancing research and therapeutic interventions. The intricate structure and functionality of the HIV genome play a vital role in its pathogenicity and interaction with host cells. Delving into these aspects not only illuminates the mechanisms behind HIV replication and integration but also reveals potential targets for treatment.
The examination goes beyond mere identification of viral components, addressing how these elements function cohesively to evade host immune responses. These characteristics underline the importance of ongoing research in this domain. To navigate the complex landscape of HIV genetics effectively, academics, clinicians, and professionals must engage with this topic thoroughly.
Research Overview
Summary of Key Findings
Recent investigations into HIV genomic structure have unveiled several critical insights:
- Genome Structure: The HIV genome consists of two identical strands of RNA. This unique feature contributes to its ability to mutate rapidly, presenting challenges for treatment.
- Functional Components: The genome encodes several proteins essential for the virus's life cycle, including reverse transcriptase, integrase, and protease. Each of these plays a pivotal role in viral replication and integration into the host genome.
- Mechanisms of Evasion: HIV employs several strategies to evade the immune system. It integrates its genetic material into the host's DNA, making detection and removal by the immune system exceptionally challenging.
These findings underline the crucial relationship between the HIV genome's structure and its impact on therapeutic outcomes. The ability to replicate and mutate rapidly underscores the urgency for innovative research and treatment strategies.
Importance of the Research
The implications of understanding the HIV genome extend beyond academic interest. They are essential for:
- Developing Therapeutics: Insights gleaned from genomic studies inform the creation of antiretroviral therapies targeting specific viral proteins and functions.
- Enhancing Prevention Strategies: Knowledge of viral genetics aids in the development of vaccines and other preventive measures, contributing to global health initiatives aimed at reducing transmission rates.
- Guiding Patient Treatment: Personalizing treatment plans based on an individual's viral genotype can improve therapeutic efficacy and manage resistance.
The intersection of genomics and therapeutic strategies highlights the continuous evolution of HIV management. Advancements in genome sequencing technologies further augment this landscape, allowing researchers to identify new patterns and variations in the virus, guiding future responses.
Methodology
Study Design
This article synthesizes data from numerous studies, encompassing diverse methodologies ranging from bioinformatics analyses to clinical trials. By evaluating previous research efforts, the article aims to create a cohesive understanding of the HIV genome's role in both research and therapy.
Data Collection Techniques
Research into the HIV genome employs several techniques:
- Next-Generation Sequencing (NGS): This technology allows for the rapid and detailed mapping of the HIV genome, enabling researchers to identify mutations and variations over time.
- CRISPR-Cas9: Utilized for gene editing, this approach helps in understanding the genetic makeup of HIV and exploring new avenues for treatment.
- Clinical Trials: Findings from these trials inform current therapeutic strategies and elucidate the impact of various treatment regimens on viral load and resistance.
Through these methodologies, researchers gather essential data that contribute to a deeper understanding of HIV and its implications for public health.
Prolusion to the HIV Genome
Understanding the HIV genome is essential for grasping how this virus interacts with host cells and drives the global epidemic of HIV/AIDS. The HIV genome comprises critical information that dictates viral replication, pathogenesis, and the evolution of the virus in response to host defenses and therapeutic strategies. Here, we explore its structure and functionality, emphasizing the distinct roles these components play in both research and therapeutic development.
Overview of HIV
Human Immunodeficiency Virus, commonly referred to as HIV, is a retrovirus primarily known for attacking the immune system, specifically the CD4+ T cells. This virus leads to the gradual deterioration of immune function, culminating in Acquired Immunodeficiency Syndrome (AIDS) if untreated. The significance of studying HIV lies not just in understanding its behavior as a pathogen but also in exploring the broader implications for public health and scientific research.
HIV exists in two main types: HIV-1 and HIV-2. HIV-1 is more prevalent globally and is associated with the majority of AIDS cases, while HIV-2 is primarily found in West Africa and is noted for its slower progression to AIDS. Every variant of the virus carries distinct genomic features, reflecting its adaptability and the challenges posed in creating effective treatments and vaccines.
Importance of Understanding the Genome
The genome of HIV is a vital factor in its pathology. It consists of single-stranded RNA which is reverse transcribed into DNA, allowing incorporation into the host's genome. This process complicates treatment efforts, as the viral genome can mutate rapidly, leading to drug resistance. Understanding the structure and function of the HIV genome helps in multiple areas:
- Therapeutic Targeting: Knowledge of the viral genome informs strategies for drug development and gene therapies.
- Vaccine Development: Insights into genetic variability enable researchers to design better vaccines that can prompt immune responses against diverse strains.
- Epidemiological Tracking: Genomic information sheds light on the transmission dynamics of HIV, helping in public health planning and resource allocation.
In essence, the HIV genome acts as a blueprint for the virus, dictating its behavior, interactions with host cells, and responses to therapeutic interventions. A deep understanding of these elements is crucial for advancing research and improving patient outcomes.
Structure of the HIV Genome
The structure of the HIV genome is crucial for understanding how the virus operates. The specific way in which the HIV genome is organized contributes not only to its replication but also to its ability to evade the immune system. By studying the structure, researchers can identify potential targets for therapy and gain insights into the characteristics that make HIV such a resilient pathogen. Here, we will explore the genomic organization and the key genetic components that are pivotal in the life cycle of the virus.
Genomic Organization
HIV's genomic organization is defined by its single-stranded RNA makeup, which is reverse-transcribed into DNA during the replication cycle. This RNA genome is approximately 9,700 nucleotides long. It is packaged within a viral particle alongside essential enzymes. The organization consists of specific genes that encode for different proteins, which are crucial for the virus's infectivity and pathogenicity. Understanding this organization helps researchers to pinpoint areas for potential intervention in therapeutic strategies.
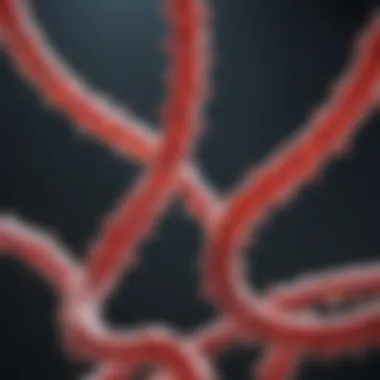
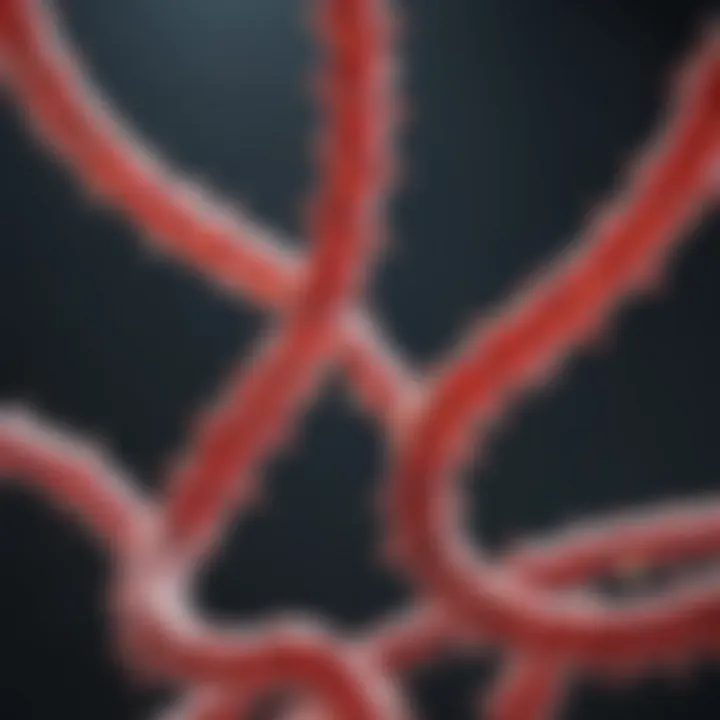
Genetic Components
The genetic components of the HIV genome encompass various proteins, each playing a significant role in the virus's lifecycle. Three primary structural proteins are notable: Gag, Pol, and Env. They are essential in forming the viral particle and ensure the virus can replicate and spread efficiently.
Gag Protein
The Gag protein is integral to the formation of the viral core. It primarily functions to encapsidate the viral RNA and enzymes during the assembly of new virions. One key characteristic of Gag is its ability to self-assemble into the virus particle, making it a popular target for research focused on disrupting virus formation. A unique feature of Gag is its interaction with the host cell membrane, which aids in the budding process, allowing the virus to exit the host cell. The advantages of targeting Gag lie in its necessity for viral assembly; however, potential disadvantages include the risk of developing resistance when targeting such a fundamental protein.
Pol Protein
The Pol protein encodes the essential enzymes that include reverse transcriptase and integrase. Its significance lies in facilitating the reverse transcription of viral RNA into DNA, a pivotal step in the HIV replication cycle. A key characteristic of Pol is its multi-functionality, which is critical for replication and integrating the viral genome into the host's DNA. This makes it a valuable target within therapeutic contexts. One unique aspect of Pol is its high mutation rate, which can lead to drug resistance. While the targeting of Pol can be effective, researchers must consider the variability and adaptability of the virus associated with this protein.
Env Protein
The Env protein is responsible for the formation of the viral envelope and plays a critical role in the attachment and entry of HIV into host cells. It is distinguished by its ability to facilitate the fusion of the viral envelope with the host cell membrane. This characteristic makes it a primary target for vaccines and therapies aiming to block infection. The unique feature of Env is that it possesses a highly variable region, which helps the virus evade the host immune response. While targeting Env can provide therapeutic benefits, the rapid mutation rate can pose challenges for vaccine development and antiviral strategies.
Regulatory Elements
Regulatory elements within the HIV genome significantly influence gene expression and viral replication.
LTR Regions
The Long Terminal Repeat (LTR) regions are found at both ends of the HIV genome and serve as key regulatory elements for transcription. The LTR regions are crucial in the integration of viral DNA into the host genome as they contain necessary signals for RNA polymerase binding. A notable characteristic is their role in regulating the timing and efficiency of viral replication. Due to these features, they are often considered in the design of gene therapies targeting HIV. The downside is that manipulation of LTR can lead to unpredictable outcomes in gene expression.
Enhancers
Enhancers present in the HIV genome increase the transcriptional activity of viral proteins. They interact with transcription factors to enhance the expression of critical genes like those encoding for the structural proteins. Enhancers are beneficial in understanding how the virus maintains robust production of virions, a significant consideration in developing antiretroviral strategies. However, their action can also result in unwanted side effects on host cells when targeting specific pathways.
Silencers
Silencers are elements that inhibit the transcription of certain HIV genes, playing a role in the balance between latency and active infection. They can limit viral production when HIV is in a dormant state. This characteristic makes silencers a critical focus for researchers aiming to reactivate latent virus reservoirs for therapeutic purposes. The challenge lies in selectively modulating silencers without adversely affecting host cell function or triggering unintended viral replication.
HIV Replication Cycle
Understanding the HIV replication cycle is fundamental for comprehending how HIV thrives within host cells. This process allows the virus to reproduce and spread, which is crucial for its survival and pathogenicity. Comprehension of this cycle not only aids in recognizing strategies the virus employs to evade the immune system but also informs the development of effective therapeutic measures. The steps involved in this cycle—entry, reverse transcription, integration, transcription and translation, and assembly and budding—are vital elements to study within the context of HIV research and treatment strategies.
Entry and Uncoating
Entry begins when HIV interacts with the host cell. The virus recognizes CD4 receptors on T cells, initiating fusion between the viral and host cell membranes. This process is facilitated by the viral envelope glycoproteins, gp120 and gp41. Following fusion, the viral capsid enters the cytoplasm of the host cell. Uncoating occurs as the capsid dissolves, releasing the viral RNA and proteins into the cell. This critical step prepares the viral components for subsequent processes and sets the stage for the next stage of the replication cycle.
Reverse Transcription
Reverse transcription is a pivotal aspect of the HIV lifecycle. The viral RNA genome is reverse-transcribed into DNA by the enzyme reverse transcriptase, which is part of the viral machinery. This process is complex and susceptible to errors, contributing to the high mutation rate of HIV. The resultant DNA molecule, known as complementary DNA (cDNA), is produced as a double-strand, integrating into the host's genetic material later. Understanding reverse transcription is crucial when designing antiretroviral therapies aimed at inhibiting this step, thus impeding viral replication.
Integration into Host Genome
Integration involves the insertion of viral cDNA into the host genome. The enzyme integrase facilitates this process, where the viral DNA becomes an integrated part of the host DNA. This insertion can lead to the establishment of a silent proviral state, whereby the virus can remain dormant within host cells for long periods. Understanding the integration mechanism is vital, as it underscores the difficulty of completely eradicating HIV from the body. Novel therapeutic targets are being explored to disrupt this process and reduce HIV's persistence within the host.
Transcription and Translation
After integration, the proviral DNA can be transcribed into mRNA, a process essential for producing viral proteins. Host cell machinery is hijacked for this purpose, allowing the virus to utilize cellular resources for its own replication. The mRNA is then translated into necessary viral proteins, including structural proteins and enzymes. This stage has critical implications for vaccine development and therapeutic interventions that can block the expression of these viral components.
Assembly and Budding
The final stage in the HIV replication cycle is assembly and budding. Newly synthesized viral proteins and RNA genomes assemble at the cell membrane. The host cell then facilitates the budding of these mature viral particles, which eventually leads to the release of new virions into the extracellular space. This enables further infection of additional host cells. Targeting this step can be key for therapeutic strategies aimed at limiting the spread of HIV within the body.
"By understanding the intricacies of the HIV replication cycle, researchers can develop targeted strategies that could potentially disrupt viral propagation at multiple points."
This cycle is a prime area for research, with implications for advancing treatments, improving patient outcomes, and understanding HIV pathogenesis more comprehensively.
Genetic Variability of HIV
The concept of genetic variability in HIV plays a crucial role in understanding the dynamics of infection and treatment. This variability directly influences the virus's behavior within the host, its ability to evade the immune system, and the development of antiretroviral resistance. As a retrovirus, HIV possesses an exceptionally high mutation rate, driven by its replication process. This aspect of its genetics not only complicates treatment but also presents challenges for vaccine development.
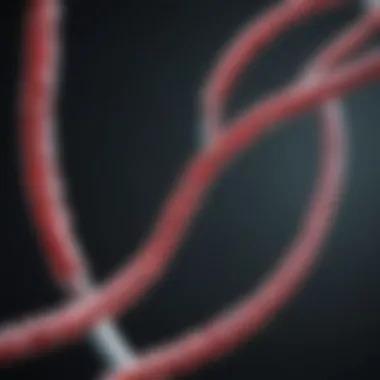
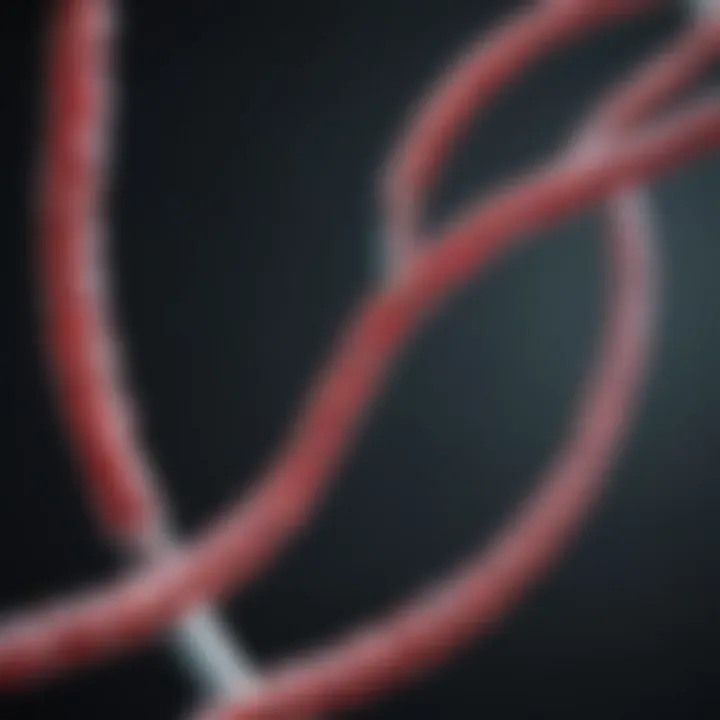
Mutation Rate
HIV has one of the highest mutation rates among known viruses. The mutation rate is approximately 1 to 10 mutations per viral genome per round of replication. This rapid rate is largely due to the reverse transcriptase enzyme, which lacks proofreading capabilities. Consequently, during the transcription of viral RNA into DNA, errors frequently occur. These mutations can be advantageous, allowing the virus to adapt swiftly to changing environments, including the host's immune response.
The high mutation rate leads to a diverse pool of viral variants within a single infected individual, complicating the immune system's response. Variants that successfully evade immune detection can proliferate, making it difficult for the body to mount an effective defense. This genetic diversity also has implications for the selection of treatment regimens, as certain viral strains may be resistant to specific antiretroviral drugs.
Quasispecies Concept
The quasispecies concept refers to the population of genetically related viral variants derived from a common ancestor. In an HIV-infected individual, the quasispecies are in a constant state of flux due to the ongoing mutations. The notion emphasizes that instead of a single viral entity, there is a complex mixture of closely related, yet distinct, viruses present. This variability can influence disease progression and response to therapy.
Researchers use the quasispecies model to better understand how HIV evolves and adapts. This approach helps in identifying which viral variants dominate during treatment failures. It also plays a role in vaccine development, as it supports the idea that an effective vaccine must account for the diversity inherent in HIV populations.
The challenge posed by genetic variability and quasispecies highlights the need for ongoing research and innovation in therapeutic strategies. Successful management of HIV not only requires effective drugs but also must consider the constantly changing landscape of viral genetics.
"Understanding the genetic variability of HIV is fundamental to deciphering its complexity and developing effective treatments."
In summary, the genetic variability of HIV significantly impacts its replication, virulence, and response to treatment. This variability must be a key consideration for researchers and healthcare professionals engaged in combating HIV/AIDS.
Host Interaction with the HIV Genome
Understanding the interactions between the host and the HIV genome is critical for evaluating the virus's pathogenicity and the efficacy of therapeutic measures. HIV's ability to manipulate host cellular mechanisms not only facilitates its survival but also challenges the immune system. Exploring these interactions offers insights into possible interventions that could help manage or eradicate the virus.
Immune Evasion Mechanisms
HIV employs various strategies to evade the host immune response, making it a particularly resilient virus. One prevalent mechanism involves rapid mutation. The high mutation rate of HIV leads to a diverse pool of viral variants. This genetic variability allows the virus to escape recognition by host antibodies and T-cells, undermining immune system efforts.
Additionally, HIV targets key components of the immune response. For instance, the virus preferentially infects CD4+ T-cells, which play a vital role in orchestrating the immune response. By depleting these cells, HIV effectively weakens the body's ability to mount a strong defense against it.
The ability of HIV to alter and adapt its genomic sequence is one of the most significant challenges in developing effective treatments.
Moreover, some viral proteins interfere with host signaling pathways. For example, the Nef protein down-regulates CD4 receptor expression on the cell surface, impairing T-cell activation and proliferation. Understanding these evasion tactics is crucial for designing vaccines and therapies that can outmaneuver HIV's strategies.
Impact on Host Cell Function
The interaction of the HIV genome with host cellular machinery has profound implications on cell function. Upon entry, the HIV genome integrates into the host's DNA, leading to a permanent presence in the host cell. This integration alters the normal functions of host cells, particularly in the immune system.
The insertion of the HIV genome can disrupt critical cellular pathways. For instance, the integration event can lead to chromosomal instability. This instability may contribute to oncogenesis, increasing the risk of cancers in individuals with chronic HIV infection. Besides, infected cells can exhibit altered cytokine production. Cytokines are important for cell signaling and coordination of the immune response.
Finally, the presence of the viral genome can induce a state of chronic inflammation. This is partly due to the immune system's constant attempt to detect and eliminate the virus. Chronic inflammation can lead to additional health complications, enhancing the importance of understanding these mechanisms.
In summary, the host interaction with the HIV genome is multifaceted and integral to the virus's lifecycle and impact on the host. Ongoing research into these interactions is essential, paving the way for innovative therapeutic strategies that can enhance patient outcomes.
Sequencing Technologies and Their Impact
Sequencing technologies have significantly transformed our understanding of the HIV genome. Their development helps in unraveling intricate details of the virus’s genetic material, enabling breakthroughs in research and therapy. As the fight against HIV continues, these technologies play a pivotal role in understanding the virus's behavior and evolution.
Advancements in Genomic Sequencing
The evolution of genomic sequencing methods has reached remarkable milestones over the past decades. Traditional Sanger sequencing laid the groundwork for a more comprehensive analysis of genomes. However, next-generation sequencing (NGS) technologies have revolutionized the field. These platforms allow for a more rapid and cost-effective sequencing of large amounts of genetic data. Importantly, techniques such as Illumina and Ion Torrent offer high-throughput sequencing capabilities, permitting researchers to obtain millions of reads in a single run.
These advancements facilitate a more detailed inspection of the HIV genome. For instance, NGS can identify minor variants in the viral population, providing insights into mutation rates and the existence of quasispecies. Understanding these variations is essential, as they may influence treatment strategies. Moreover, the ability to sequence multiple HIV strains from a single patient enhances the understanding of how the virus adapts to antiretroviral drugs or the host immune response.
Applications in HIV Research
The applications of sequencing technologies in HIV research are vast and vital. They enable scientists to:
- Characterize Viral Diversity: Sequencing allows for the analysis of genetic variability among different HIV strains. This understanding is crucial for developing effective vaccines and therapies.
- Monitor Treatment Efficacy: Regular sequencing of viral genomes can uncover resistance mutations, leading to timely adjustments in therapy.
- Study Molecular Epidemiology: By sequencing HIV from various populations, researchers can track transmission dynamics and identify outbreaks more efficiently.
"Advancements in sequencing technologies not only enhance our comprehension of HIV but also drive innovation in treatment pathways."
Furthermore, sequencing is instrumental in studying the evolution of the virus within an individual over time. This longitudinal approach assists researchers in understanding how HIV might evade immune responses and develop resistance, providing insights critical for future therapeutic developments. Overall, sequencing technologies hold endless potential in advancing the fight against HIV, shaping both current and future strategies against this persistent global health threat.
Therapeutic Strategies Targeting the HIV Genome
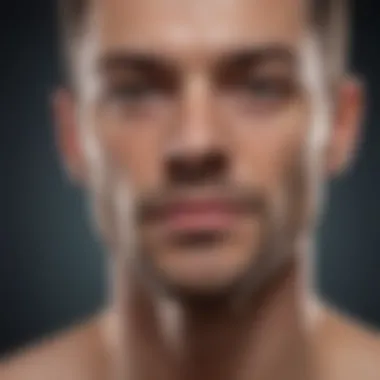
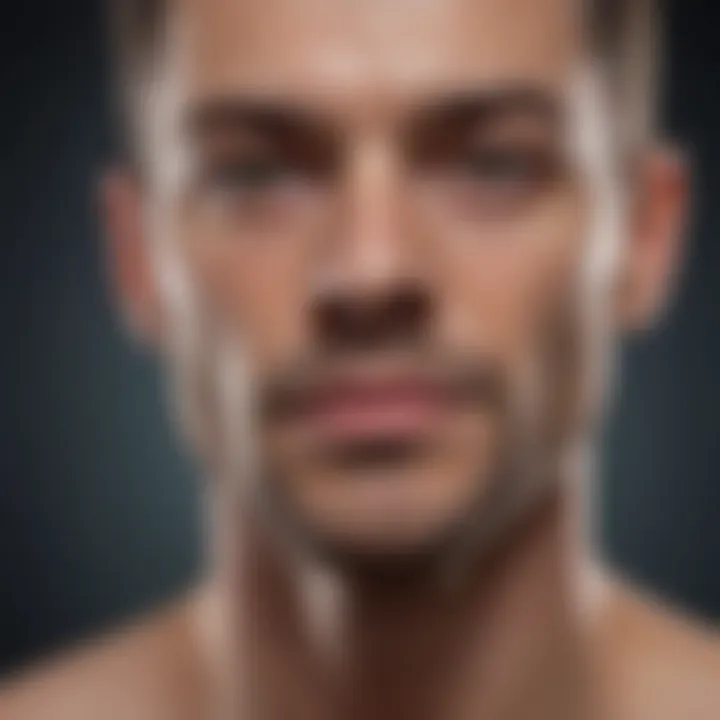
Therapeutic strategies that target the HIV genome are vital in the ongoing efforts to combat HIV/AIDS. These strategies not only enhance treatment efficacy but also aim at preventing the replication and spread of the virus within the host. Given the complexities of HIV biology, these methods focus on both reducing the viral load and improving the immune response against the virus. Understanding these strategies enables researchers and healthcare professionals to implement effective protocols for managing infected individuals.
Antiretroviral Therapy
Antiretroviral therapy (ART) represents a cornerstone in the treatment of HIV infection. It involves the use of multiple antiretroviral drugs to suppress the virus and prevent progression to AIDS. The primary goal of ART is to keep the viral load low, ideally to undetectable levels. This not only protects the immune system but also reduces the risk of HIV transmission.
The main classes of antiretroviral drugs used in ART include:
- NRTIs (Nucleoside Reverse Transcriptase Inhibitors) — These block reverse transcription, disrupting HIV's ability to replicate.
- NNRTIs (Non-Nucleoside Reverse Transcriptase Inhibitors) — They also inhibit reverse transcription but in a different manner, providing an alternative mechanism of action.
- PIs (Protease Inhibitors) — These prevent the maturation of viral particles, effectively keeping the virus inactive.
- Integrase Inhibitors — They block the integration of viral DNA into the host genome, a crucial step in HIV's life cycle.
Effective use of ART leads to viral suppression and allows individuals to lead healthy lives. However, adherence to therapy is crucial, as missing doses can lead to the emergence of drug-resistant HIV strains.
Gene Editing Techniques
Gene editing techniques present a novel approach to targeting the HIV genome. These techniques aim to modify the viral genome or the host cell's genetic material to eliminate the virus altogether. Among these techniques, CRISPR/Cas9 and TALENs are the most notable for their precision and effectiveness.
CRISPR/Cas9
CRISPR/Cas9 is a groundbreaking gene-editing tool that allows for the specific targeting and alteration of DNA sequences. Its contribution to HIV research is significant, as it can directly target the viral genome.
One key characteristic of CRISPR/Cas9 is its ability to create double-strand breaks at specific locations in the DNA. This unique capability allows it to disable the HIV genome effectively. The simplicity and versatility of this technology make it a popular choice. It is widely regarded for its potential in both research and therapeutic settings. However, some limitations exist, such as possible off-target effects that can unintentionally alter other important genes in the host genome.
TALENs
Transcription Activator-Like Effector Nucleases (TALENs) are another gene-editing approach that shows promise in targeting HIV. TALENs are engineered proteins that facilitate targeted modifications in the DNA, disrupting the HIV genome to hinder its replication. Their key characteristic lies in their modular design, allowing for precise targeting of nearly any DNA sequence, which is crucial for effective HIV intervention.
The unique feature of TALENs is their high specificity in binding to target DNA, significantly reducing the chances of off-target effects compared to some methods. Despite their effectiveness, TALENs are generally more complex to design and implement than CRISPR/Cas9, which may limit their widespread adoption in clinical settings.
Vaccination Efforts
Vaccination efforts against HIV remain a critical area of research. The goal is to stimulate a robust immune response that can prevent infection or control the virus effectively in those already infected. Current challenges include the high genetic variability of the virus and its ability to evade the immune system. Nonetheless, ongoing research continues to optimize vaccine candidates to enhance their efficacy and applicability to a broader population.
Future Directions in HIV Research
The landscape of HIV research is evolving rapidly. Understanding the complexities of the HIV genome is crucial as it informs both scientific inquiries and therapeutic interventions. Future research directions are essential for developing effective treatments and improving patient outcomes. This section delves into the significance of continued research and the potential for novel therapeutics.
Importance of Continued Research
The pursuit of knowledge in HIV research is more vital now than ever. Despite significant advancements in treatment, HIV remains a global health challenge. Continuous research helps identify new aspects of the virus that can be targeted for therapeutic purposes. Specific areas of focus include:
- Viral reservoirs: Understanding how HIV persists in the body despite treatment. This could lead to strategies for eliminating these reservoirs.
- Resistance mechanisms: Studying how the virus develops resistance to antiretroviral drugs is crucial for improving current therapies.
- Host genetic factors: Exploring how individual genetic makeup affects HIV infection and treatment responses. This can lead to personalized treatment approaches.
The ongoing commitment to research is necessary to uncover these aspects and develop better strategies for managing HIV, ultimately improving the quality of life for those affected.
Potential for Novel Therapeutics
Emerging therapeutic strategies show promise for combating HIV more effectively. Researchers are exploring various innovative approaches:
- Broadly neutralizing antibodies (bNAbs): These antibodies have the ability to neutralize diverse strains of HIV. They could be used in combination with existing treatments for enhanced effectiveness.
- Therapeutic vaccines: Unlike preventive vaccines, therapeutic vaccines aim to boost the immune response in already infected individuals. The goal is to control the virus without continuous antiretroviral therapy.
- Gene therapy: Techniques like CRISPR/Cas9 offer exciting possibilities for editing the HIV genome directly. This could potentially lead to the eradication of the virus from infected cells.
In summary, the future of HIV research holds great promise. Continuous inquiry is essential to unveil new therapeutic opportunities. Expanding our understanding of the HIV genome will provide critical insights while guiding practical applications. The intersection of research and therapy presents an opportunity for significant strides in controlling and potentially eradicating HIV.
End
The study of the HIV genome is vital for various reasons. Understanding its structure and function has significant implications for developing therapeutic strategies and treatment approaches. The complexity of the viral genome, with its unique components and regulatory mechanisms, offers insights into how HIV interacts with host cells and evades the immune response.
Moreover, this understanding is not merely academic. It translates to tangible benefits in the clinic. Researchers can identify novel targets for therapies that disrupt the viral life cycle or enhance host immune responses. Such insights are essential in the ongoing fight against the HIV/AIDS epidemic, where effective treatment remains a critical need.
The advancement of genomic technologies has further amplified our ability to study the HIV genome in detail. This paves the way for better vaccine designs, refined antiretroviral therapies, and innovative gene-editing techniques. All these strategies depend on a robust understanding of the virus's genetic makeup.
Summary of Key Points
- The HIV genome plays a crucial role in the virus's replication and integration into the host's DNA.
- Understanding the components and structure of the HIV genome is pivotal for developing effective treatments and vaccines.
- Advances in sequencing technologies have revolutionized HIV research, allowing for more detailed exploration of the viral genome.
- Targeted therapeutic strategies, including antiretroviral therapy and gene editing, hinge on in-depth knowledge of the HIV genome.
Final Thoughts on HIV Genome Research
Research into the HIV genome remains a dynamic field. The more we learn, the better equipped we are to combat this virus. Continuous research efforts are necessary to uncover the complexities of HIV genetics and their implications for treatment.
As scientists strive towards key innovations, collaboration across disciplines will be vital. There is also the need for ethical considerations when deploying new technologies to ensure they meet the needs of affected populations. The insights gained from this research not only contribute to stopping the virus but also foster advancements in our understanding of viral diseases in general.