Innovative Applications in Plant Sciences
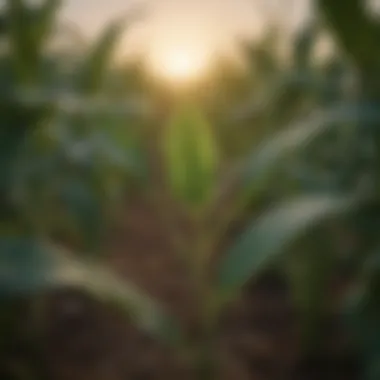
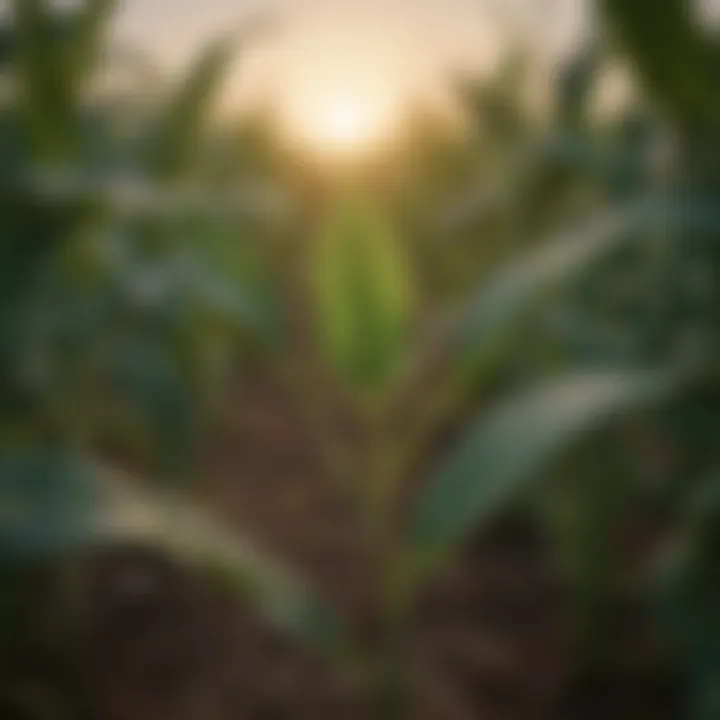
Intro
The field of plant sciences plays a crucial role in addressing many of the challenges faced in modern agriculture and environmental sustainability. With ongoing advancements in technology and research, the integration of fields such as biotechnology and genomics has opened new avenues for improvement in crop yields, disease resistance, and biodiversity preservation. This article seeks to provide a comprehensive understanding of these multifaceted applications by examining key developments, methodologies, and their implications for the future of agriculture and the planet.
Research Overview
Summary of Key Findings
Recent studies highlight several significant applications in plant sciences that have transformative potential. Innovations in genetic engineering, for instance, foster the development of crops with desirable traits, such as drought resistance and higher nutrient content. Research indicates that the use of CRISPR technology can precisely edit plant genomes, leading to faster growth cycles and healthier plants.
Another area of focus is the role of biopesticides. Derived from natural materials, these alternatives to chemical pesticides demonstrate effectiveness while minimizing harm to non-target organisms and the environment. Studies show a marked decrease in chemical runoff in areas where biopesticides are adopted.
Importance of the Research
Understanding the applications of plant sciences is essential for several reasons. The growing global population necessitates an increase in food production. Additionally, climate change poses a challenge to traditional farming practices, making innovative approaches vital to ensure food security.
Moreover, this research contributes to biodiversity. As ecosystems face degradation, sustainable practices in agriculture can help preserve various plant species and promote ecosystem health. Implementing advanced methodologies in plant sciences aligns with global efforts to create a more sustainable future.
Methodology
Study Design
The methodology in these research studies frequently employs both experimental and observational designs. This allows for a comprehensive collection of data on plant responses to various treatments and conditions. Controlled experiments are often conducted in laboratory settings to assess specific variables under precise conditions.
Data Collection Techniques
Data collection techniques vary, but two common methods include:
- Field Trials: These allow researchers to assess the performance of new crop varieties in real-world conditions.
- Laboratory Analyses: Techniques such as molecular profiling help in understanding the genetic makeup of plants and their response to environmental stressors.
Research in plant sciences thus relies on an interplay of innovative design and rigorous data gathering to achieve meaningful results. This systematic approach facilitates advancements that not only enhance agricultural practices but also address broader environmental concerns.
"Advancements in plant sciences not only hold potential for increasing agricultural productivity but also embody crucial strategies for environmental stewardship."
Preface to Plant Sciences
Plant sciences encompass a wide range of disciplines that study various aspects of plant life, including their biology, ecology, and practical applications, particularly in agriculture. The significance of plant sciences lies not merely in the understanding of plants themselves, but also in their substantial implications for food security, environmental sustainability, and biodiversity. As the global population continues to grow, the demand for increased agricultural productivity intensifies. Here, plant sciences play a crucial role in addressing these challenges by providing innovative solutions derived from research and technological advancements.
The field involves several branches, such as plant physiology, plant pathology, and plant breeding. Understanding plant physiology helps in optimizing how crops grow and how they respond to different environmental factors. Similarly, plant pathology focuses on understanding diseases that affect plants, allowing for better management strategies to ensure that crops remain healthy and productive.
Moreover, this field brings forth the integration of biotechnology and genetic engineering, enhancing the ability to develop crops that are more resilient to pests, diseases, and changing climate conditions. This intersection between science and technology is vital now more than ever, as researchers and practitioners seek to unlock the potential of plants to benefit society.
Key areas of focus in plant sciences include:
- Crop enhancement through genetic modifications and selection techniques.
- Disease management employing integrated pest management strategies to combat issues associated with agricultural production.
- Sustainable practices aimed at minimizing environmental impact while maintaining productivity.
The exploration of plant sciences reveals the broader environmental context in which these practices operate. For instance, understanding how plants interact with their ecosystems contributes to effective conservation strategies, vital for maintaining biodiversity. Furthermore, recognizing the effects of climate change on plant systems is imperative for developing adaptive measures to preserve agricultural productivity.
Evaluating the implications of plant sciences is essential. Ongoing research not only enhances our understanding of how plants can be utilized more effectively but also fosters better practices in managing agricultural practices. In this context, informing best practices based on evidence and promoting sustainable approaches are pivotal to future advancements in the field.
"Innovative approaches in plant science are crucial for sustainable agriculture, addressing both the challenges of food production and the imperatives of environmental stewardship."
Historical Context of Plant Sciences
The historical context of plant sciences serves as a crucial foundation for understanding the advancements in this field today. The evolution of our knowledge about plants has occurred over centuries, beginning with early agricultural practices. Ancient civilizations utilized plants not only as food sources but also for medicinal purposes and rituals. This relationship set the stage for the scientific inquiries that would follow.
In the 18th century, notable developments took place, such as the classification of plants by Carl Linnaeus. His binomial nomenclature system provided a systematic way to name and categorize species, which is still in use today. This was significant as it laid groundwork for future research and an organized method to study plant biology.
The 19th century saw a surge in exploration and botanical studies. This era expanded our understanding of plant anatomy and physiology. Researchers like Gregor Mendel contributed to the understanding of heredity, which impacted breeding practices across various crops.
Additionally, the advent of industrial agriculture during the late 19th and early 20th centuries led to extensive research into improving crop yields and pest management. These developments fostered a more systematic approach to agriculture, ultimately leading to more consistent food production.
The 20th century marked a shift towards molecular biology and genetics. Techniques such as DNA sequencing began to transform our understanding of genetic information in plants. The work in this period spawned biotechnological innovations, significantly shaping modern agriculture practices.
In recent decades, the urgency of global challenges, such as food insecurity and environmental degradation, has brought about a renewed focus on plant science. The intersection of environmental science, molecular biology, and biotechnology has fostered advances that aim to solve pressing agricultural issues while ensuring sustainability.
Overall, the historical context of plant sciences highlights the continuous evolution of human interaction with plants, leading to innovative approaches that address both agricultural and environmental concerns. This legacy underscores how past achievements inform current practices, revealing the interconnectedness of historical and contemporary plant sciences.
Current Trends in Plant Research
Current trends in plant research are crucial for understanding how modern science can enhance agricultural productivity and sustainability. The ever-evolving nature of agriculture necessitates constant adaptation to environmental changes, technological advancements, and societal needs. Researchers are focusing on several key areas that have profound implications for how we grow food and interact with the natural world.
Genomics and Gene Editing
One major trend is the advent of genomics and its application in crop improvement. Technologies like CRISPR are at the forefront of this movement, enabling precise modifications to plant genomes. This innovation allows scientists to enhance desirable traits, such as disease resistance and drought tolerance, without introducing foreign DNA, which has been a concern in traditional genetic modification. The manipulation of plant genes can lead to significant increases in crop yields and resilience against climate stresses.
Data-Driven Agriculture
Another trend gaining momentum is data-driven agriculture, which utilizes big data and analytics to optimize farming practices. Precision agriculture tools provide real-time information on soil health, crop status, and environmental conditions. This data can help farmers make informed decisions, reduce resource waste, and improve yield efficiency. The integration of remote sensing, drones, and IoT (Internet of Things) technologies is transforming farming into a more science-based industry.
"Data and technology are no longer optional; they are essential for successful modern agriculture."
Sustainability Practices
Sustainable agricultural practices are also a focal point in current research. Scientists are exploring ways to minimize the ecological footprint of farming. Techniques such as cover cropping, crop rotation, and organic farming are now supported by research that quantifies their benefits. Additionally, there's a growing emphasis on agroecology, aiming to create farming systems that work in harmony with natural ecosystems. This trend is critical for ensuring food security while preserving biodiversity.
Climate Adaptation
As climate change continues to affect weather patterns and growing conditions, researchers are prioritizing studies that mitigate its impacts on plant systems. The development of climate-resilient crops is essential for adapting to anticipated environmental changes. Research in this area focuses on identifying genes associated with resilience traits and deploying them in breeding programs.
Collaborations and Interdisciplinary Research
Finally, interdisciplinary collaborations are becoming increasingly important. Integration of various fields—such as biology, chemistry, environmental science, and engineering—enrich plant research outputs. Collaborative efforts can lead to innovative solutions that tackle complex issues, such as pest resistance and nutrient management.
In summary, the current trends in plant research emphasize a multifaceted approach to both improving agricultural efficiency and promoting environmental sustainability. Advancements in technology and a focus on collaboration are key components that define the future landscape of plant sciences.
Biotechnology and Genetic Engineering
Biotechnology and genetic engineering play crucial roles in modern plant sciences. These fields have enabled significant advances in how we understand and manipulate plant systems. Biotechnology involves using biological processes for various applications, ranging from agriculture to environmental conservation. Genetic engineering takes this a step further by allowing scientists to directly modify the DNA of organisms, enhancing desired traits in plants.
The advantages of incorporating biotechnology and genetic engineering in agricultural practices are numerous. They improve crop yield, increase resistance to pests and diseases, and enhance nutritional quality. With the rising global population, innovative solutions are needed to ensure food security. Moreover, these technologies can lead to sustainable practices by reducing the need for chemical pesticides and fertilizers. However, this also brings ethical questions and public scrutiny regarding the long-term effects on human health and ecosystems.
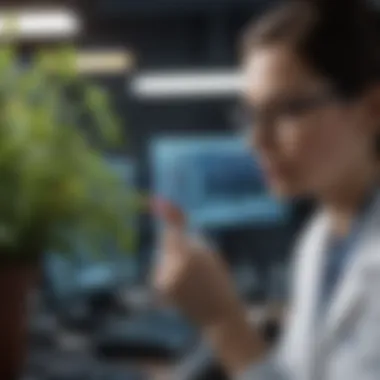
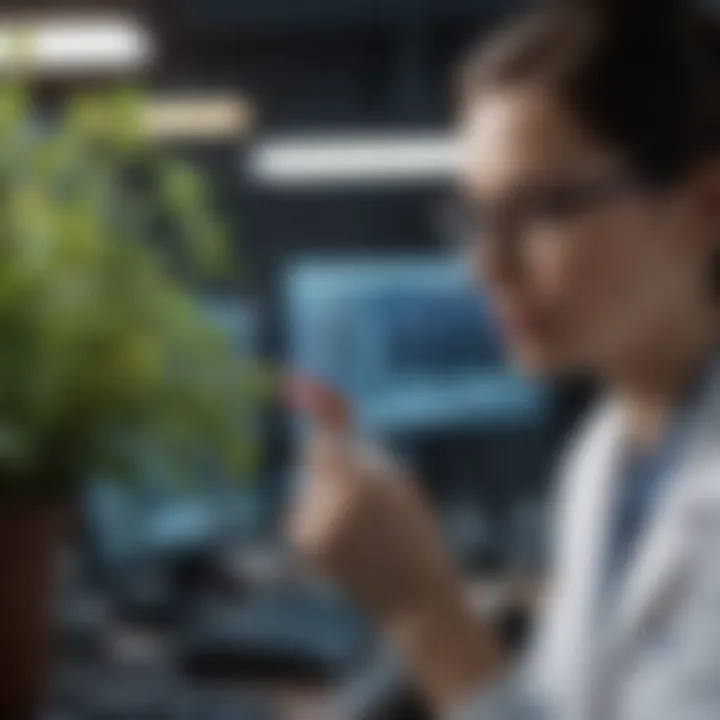
"The integration of biotechnology in plant science presents both challenges and opportunities that must be navigated carefully."
CRISPR Technology in Genomic Editing
CRISPR technology has revolutionized genetic engineering in plants. This precise gene-editing tool allows scientists to make very specific changes to plant genomes, enabling them to enhance traits such as drought tolerance or disease resistance. The ease of use and cost-effectiveness of CRISPR make it an attractive option compared to previous methods.
For instance, researchers can employ CRISPR to introduce or modify specific genes in staple crops like rice or wheat. This results in plants that not only yield more but also manage environmental stresses more effectively. Furthermore, it opens up possibilities for quicker development cycles in plant breeding, leading to faster results.
Transgenic Crops: Benefits and Concerns
Transgenic crops are genetically modified organisms (GMOs) made by inserting genes from one species into another. The primary benefits include improved pest resistance, herbicide tolerance, and larger harvests. Crops like Bt corn and Roundup Ready soybeans are prime examples, allowing farmers to produce food more efficiently and at lower costs.
However, the adoption of transgenic crops is not without controversy. Public concern regarding health safety and environmental impact remains significant. Many consumers are uneasy about the potential long-term effects and ecological balance. Additionally, there are doubts about corporate control over seed supply, raising issues of equity and access for smallholder farmers.
Plant Physiology and Developmental Biology
Plant physiology and developmental biology are crucial fields within plant sciences, offering insights into how plants function and grow. Understanding the mechanics of plant life is vital not only for improving agricultural practices but also for addressing broader ecological and environmental issues. In this section, we will examine the specific elements of plant physiology and developmental biology, focusing on the benefits they provide in agriculture and the considerations that researchers must keep in mind.
Plant physiology centers on the various systems and processes that allow plants to survive and thrive. It includes the study of fundamental processes such as photosynthesis, respiration, and nutrient uptake. These processes are essential for the growth and productivity of plants. The focus on plant growth and development helps researchers identify ways to optimize these processes, leading to enhanced yield and resilience in crops.
"Studies in plant physiology can lead to innovative solutions for increasing agricultural productivity and sustainability."
Photosynthesis Efficiency Enhancements
Photosynthesis is perhaps the most critical process in plant physiology, as it directly impacts how well plants can convert sunlight into energy. Recent advancements aim to enhance photosynthetic efficiency in several ways. Research is exploring methods to increase the light absorption capability of plants or improve the conversion efficiency of light energy into chemical energy.
One approach is the genetic modification of plants to produce more efficient photosynthetic enzymes. By altering the expression of certain genes, scientists can create plants that use light more effectively, potentially resulting in higher biomass production. Moreover, the use of controlled environments, such as greenhouses, aids in maximizing light exposure and optimizing other growth conditions.
The implications of enhancing photosynthesis are significant. Better performing crops can lead to increased yields, which is essential in the context of global food security and climate change.
Role of Hormones in Plant Growth
Plant hormones play a fundamental role in regulating growth and development. They are responsible for a wide variety of functions, including seed germination, root growth, and flowering. Understanding how hormones act in plants allows scientists to manipulate these processes for agricultural benefit.
There are several major plant hormones that researchers focus on: auxins, gibberellins, cytokinins, abscisic acid, and ethylene. Each hormone has a distinct function. For example, auxins influence cell elongation and are key in phototropism, the growth of a plant toward light.
Manipulating these hormones can lead to improved plant responses to various stimuli, including stress factors such as drought or nutrient deficiency.
Research in this area can contribute to developing crops that adapt better to environmental stressors, ensuring food production even in challenging conditions.
Plant Pathology and Disease Management
Plant pathology plays a crucial role in ensuring the sustainability of plant systems and agricultural productivity. It encompasses the study of plant diseases caused by pathogens such as fungi, bacteria, viruses, and nematodes. Understanding these diseases is essential for developing effective management strategies that minimize crop losses and promote healthy ecosystems. Without proper management of plant diseases, food security can be severely compromised, resulting in economic instability for farmers and communities dependent on agricultural production. In this section, we will dive into two core areas of plant pathology: fungal and bacterial diseases, and integrated pest management strategies.
Fungal and Bacterial Diseases
Fungal and bacterial diseases represent significant threats to crops worldwide. Fungi, such as Phytophthora and Fusarium, can devastate entire fields if not promptly addressed. Symptoms might include wilting, leaf spots, and root rot. Bacterial diseases, on the other hand, often manifest as water-soaked lesions or blights. These pathogens can easily spread via water, tools, or through infected seeds, making management challenging.
Strategies for combating these diseases must be multifaceted. Early detection plays a vital role. Techniques such as remote sensing and visual inspections help identify issues before they spread. Additionally, the use of disease-resistant cultivars can significantly reduce the impact of these diseases. Integrated management practices might include crop rotation, sanitary measures, and biological control methods.
"Plant diseases are not just about the symptoms they present. They are part of a larger interaction within the ecosystem."
Integrated Pest Management Strategies
Integrated Pest Management (IPM) is a holistic approach combining various management strategies to control pests and diseases effectively. It aims not only to reduce pest populations but also to minimize harm to the environment and non-target organisms.
Key components of IPM include:
- Cultural Control: This involves practices such as crop rotation and planting resistant varieties to disrupt the life cycles of pests and pathogens.
- Biological Control: Utilizing natural predators or pathogens to manage pest populations is a sustainable alternative to chemical applications.
- Monitoring: Regular assessments of pest and disease populations inform decisions on timely interventions.
- Chemical Control: When necessary, the use of pesticides that are environmentally friendly and target specific pests can be employed.
The essence of IPM lies in its adaptability. Each agricultural situation is unique, requiring tailored solutions based on local conditions and pest pressures. This adaptive strategy not only sustains crop health but also fosters resilience against plant diseases in the context of climate change and environmental variability.
Environmental Stress Responses
Environmental stress responses are critical for understanding how plants adapt to changing conditions. This area of study examines the physiological and biochemical processes that plants use to cope with various stressors. These stressors may include factors such as drought, salinity, extreme temperatures, and pathogens.
By studying these responses, researchers can identify mechanisms that allow plants to survive in less than ideal environments. Understanding how plants interact with their environment provides insights that are crucial for agricultural productivity, particularly in the face of climate change and resource scarcity.
The implications of environmental stress responses extend beyond individual plant survival. They are essential in developing strategies for sustainable agriculture. Enhancing plant resilience can lead to improved crop yields, reduced dependency on chemical inputs, and heightened biodiversity.
Effective management of environmental stress responses is vital for optimizing agricultural practices in a world facing increasing climatic uncertainties.
Drought and Salinity Tolerance
Drought and salinity are two of the most significant abiotic stresses affecting crop production globally. Drought limits water availability, while salinity increases the salt concentration in the soil, both of which can severely hinder plant growth and productivity. Understanding the mechanisms behind drought and salinity tolerance has become paramount for researchers.
Plants have developed various strategies to combat these stresses. Some mechanisms include:
- Root System Adaptations: Expanding root systems allow plants to access deeper water sources.
- Osmotic Adjustment: This involves accumulating solutes that help maintain cell turgor and hydration.
- Stomatal Regulation: Controlling stomatal closure minimizes water loss through transpiration.
Research on drought and salinity tolerance aims to identify specific traits that can be harnessed in breeding programs. Advances in biotechnology are also providing new avenues for developing stress-resistant plant varieties.
Responses to Climate Change
Climate change poses severe challenges for plant systems, affecting growth patterns, flowering times, and overall plant health. Increased temperatures, fluctuating precipitation patterns, and elevated CO2 levels are altering the dynamics of plant ecosystems.
Plants exhibit various responses to these changes, including:
- Phenological Changes: Alterations in the timing of life cycle events, affecting reproductive success and plant fitness.
- Physiological Adjustments: Modifications in photosynthesis rates and water use efficiency to cope with varying climate conditions.
- Genetic Adaptation: Over time, some species may evolve changes in their genetic makeup, enhancing their survival amid shifting climates.
Addressing climate change effects on plants requires a comprehensive approach. It involves integrating research findings across disciplines, employing both traditional methodologies and cutting-edge technologies to enhance understanding and guide future agricultural practices.
Sustainable Agriculture Practices
Sustainable agriculture practices are essential in today’s world as they address numerous environmental and social challenges. They promote agricultural methods that do not deplete natural resources while also ensuring that the needs of future generations are met. Its importance is particularly highlighted in light of climate change, soil degradation, and the need for food security.
Sustainable agriculture encompasses a variety of practices aimed at reducing the negative impacts of farming on the environment. These practices include crop rotation, cover cropping, and reduced tillage. Each of these methods contributes towards maintaining soil health, conserving water, and enhancing biodiversity. By incorporating these techniques, farmers can improve their crop yields while ensuring the ecosystem remains balanced.
"The integration of sustainable practices can lead to enhanced food security and resilience against climate change."
In addition to environmental benefits, sustainable agriculture also has socio-economic advantages. Economically, it can lead to cost savings through reduced reliance on chemical inputs like fertilizers and pesticides. Socially, it fosters community engagement and promotes local economies through support of smaller farms. Understanding these several facets of sustainable agriculture opens up pathways for innovation in food production.
Organic Farming Techniques
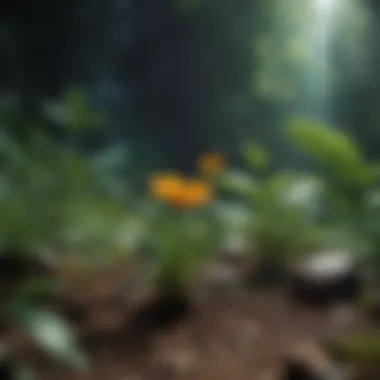
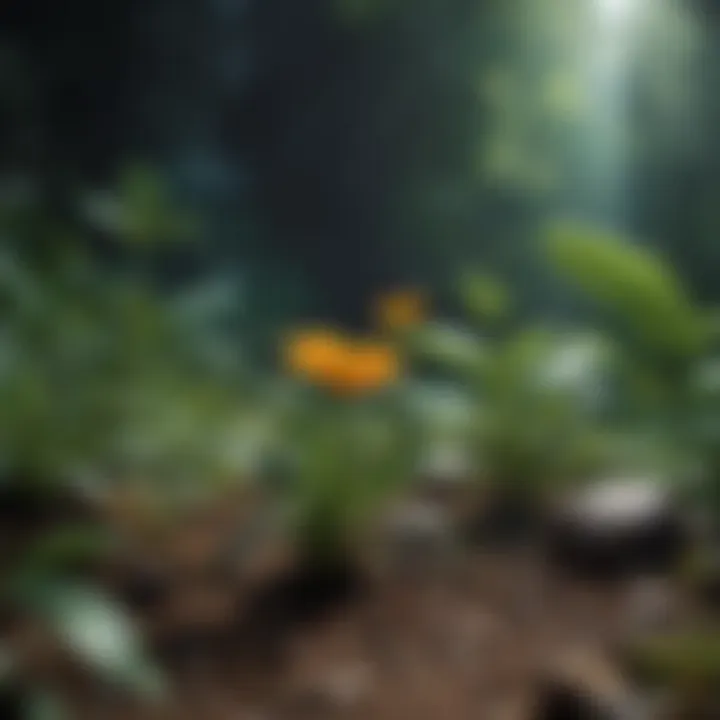
Organic farming techniques focus on producing food without synthetic fertilizers, pesticides, or genetically modified organisms. This method relies on natural processes to enhance soil fertility and manage pests. The use of compost, cover crops, and crop rotation are common practices within organic systems. These techniques not only foster healthier plants but also promote biodiversity by creating habitats for beneficial organisms.
The transition to organic farming has gained traction for several reasons:
- Reduction of chemical pollution in ecosystems
- Possible price premiums for organic products in the market
- Enhanced health benefits for consumers due to reduced chemical residues
Organic certification, although sometimes considered complicated, serves as a cornerstone for consumer trust and market growth. This creates an opportunity for farmers to engage in sustainable practices with a wider recognition.
Permaculture and Agroecology
Permaculture and agroecology are interconnected disciplines that emphasize the design of agricultural systems that mimic natural ecosystems. Permaculture focuses on creating sustainable and self-sufficient agricultural landscapes by utilizing local resources effectively. It involves the design of thoughtful systems combining various agricultural techniques.
Agroecology, on the other hand, emphasizes the application of ecological principles to agricultural practices that enhance sustainability and equity in food systems. It encourages diversity, resilience, and ecological balance, often integrating social and economic considerations into agricultural development.
Some key principles of permaculture and agroecology include:
- Diversity of species to promote ecosystem resilience
- Use of local resources to minimize ecological footprints
- Closed-loop systems that reduce waste
By implementing these models, farmers can create systems that are not only productive but also restore ecosystems and support the surrounding communities. These practices can significantly contribute to sustainable development goals and enhance the overall health of the planet.
Plant Breeding Innovations
Plant breeding innovations play a critical role in increasing crop productivity and sustainability. As global food demand rises, the need for more resilient and higher-yielding plants has become crucial. These innovations integrate traditional methods with modern biotechnological approaches. Utilizing genetics and molecular techniques, these practices aim to produce crops that can withstand various stresses including diseases, pests, and climate variability.
The use of plant breeding innovations helps in addressing food security issues. Such advancements also align with sustainable agriculture goals by minimizing chemical inputs and reducing environmental impact. For example, producing disease-resistant varieties can lead to lower pesticide use, which benefits both the ecosystem and health of consumers.
Key considerations in plant breeding innovations include ensuring genetic diversity and taking into account the potential social implications of these advancements. It is important to consider the acceptance of genetically modified organisms (GMOs) by the public and the regulatory landscape governing their use.
Highlighting both benefits and potential risks is essential in developing responsible breeding practices. The innovations within this field aim not only to enhance crop resilience but also to foster ecological balance.
“Integrating science and traditional knowledge can lead to more robust and sustainable plant breeding strategies.”
Marker-Assisted Selection
Marker-assisted selection (MAS) is a significant innovation in plant breeding. This approach utilizes molecular markers linked to desired traits, enabling breeders to select plants that carry these markers more efficiently. Instead of traditional breeding methods, which can take years to select for specific traits, MAS speeds up the process considerably.
It allows for the identification of traits such as drought tolerance, disease resistance, or improved nutritional quality at an early stage. By employing MAS, breeders can make informed decisions based on genetic data rather than solely relying on phenotypic assessment. This increases precision in breeding and reduces the risk of introducing unwanted traits.
Hybrid Varieties and Their Impact
Hybrid varieties represent another critical aspect of plant breeding innovations. Hybrids are created by crossing two genetically distinct parent lines. The resulting offspring often exhibit superior qualities, such as higher yields and improved disease resistance. This is due to heterosis, or hybrid vigor, where the hybrid offspring perform better than their parents.
The impact of hybrid varieties extends beyond yield improvement. They can also increase resource use efficiency, allowing farmers to grow more food with less water and fertilizer. Despite the benefits, the cultivation of hybrid varieties comes with challenges. Farmers must purchase new seeds each year, which may increase costs; however, the potential yield gains can often offset these expenses.
In summary, plant breeding innovations such as marker-assisted selection and hybrid varieties offer promising solutions for modern agricultural challenges. They enhance the potential for food security while promoting sustainable practices.
Impact of Climate Change on Plant Systems
Climate change stands as one of the most formidable challenges facing plant systems today. As temperatures rise and weather patterns shift, plants are subjected to new environments that can drastically affect their growth and distribution. Understanding these dynamics is critical not only for the survival of various species but also for global food security.
Effects on Plant Distribution
The distribution of plant species is highly sensitive to climate change. As conditions on Earth evolve, some species may find their ideal habitats shifting. For example, warmer temperatures might lead to a northward migration of certain species, while others may struggle to adapt and face potential extinction. Changes in precipitation patterns can also redefine habitats, impacting plant survival.
- Shifts in Range: Certain species will migrate to cooler regions, affecting biodiversity and ecosystem dynamics.
- Alteration of Ecosystems: This migration can lead to competition with native plants, disrupting established ecosystems.
- Threat to Endemic Species: Plants that are specialized to certain climatic conditions may not adapt, resulting in biodiversity loss.
The direct impacts of climate change on plant distribution highlight the importance of monitoring and conservation efforts to mitigate potential losses in biodiversity.
Adaptation Mechanisms in Plants
Plants have developed various mechanisms to adapt to environmental changes. These mechanisms underpin their ability to survive under stress from climate change. Key adaptation strategies include:
- Physiological Adjustments: Many plants adjust their metabolic processes to cope with increased temperatures and varying moisture levels.
- Morphological Changes: Some species may develop deeper roots to access water or change leaf structures to reduce transpiration rates.
- Phenological Shifts: Changes in the timing of flowering and fruiting can allow plants to better align their reproductive cycles with favorable climate conditions.
Understanding these adaptation mechanisms not only informs researchers but also aids in the development of resilient agricultural practices.
Overall, the impact of climate change on plant systems is complex and multifaceted. Ongoing research and adaptive strategies are essential for helping plant systems cope with ongoing environmental changes.
Technological Advances in Plant Sciences
Technological advances in plant sciences play a crucial role in addressing the urgent challenges facing agriculture and the environment. These innovations encompass a variety of tools and methodologies that enhance our understanding of plant biology, improve crop yields, and ensure sustainable practices. As populations grow and climate change becomes more pronounced, the integration of technology in plant sciences is not merely beneficial but essential.
The significance of this topic is evident through specific elements such as precision agriculture and nanotechnology. Both approaches illustrate how technology can be tailored to meet individual crop needs and optimize plant growth conditions while minimizing resource waste. Consideration of both the benefits and potential drawbacks of these innovations is vital for shaping a sustainable agricultural future.
"Technological advances in plant sciences not only improve yields but also contribute to the preservation of natural resources."
Remote Sensing and Precision Agriculture
Remote sensing refers to the use of satellite or aerial imagery to monitor and manage agricultural land. This technology provides farmers with detailed data regarding soil health, moisture levels, and crop conditions. The integration of remote sensing in precision agriculture enables targeted interventions, thereby increasing efficacy and reducing environmental impact.
The primary advantages of remote sensing include:
- Data-Driven Decision Making: Farmers can make informed choices based on real-time data rather than guesswork.
- Resource Management: Precision application of fertilizers and pesticides minimizes excess usage and runoff into nearby ecosystems.
- Increased Yields: By understanding crop stress and growth patterns, farmers can enhance productivity.
Yet, the adoption of this technology comes with considerations such as the initial costs and the need for training. Farmers must be equipped with the skills to interpret the data effectively. This aspect is crucial for ensuring that technological advances yield their full potential benefits.
Nanotechnology in Crop Improvement
Nanotechnology involves manipulating materials on an atomic or molecular scale. In the realm of plant sciences, it has emerged as a groundbreaking approach to enhance crop improvement. Various nanomaterials promote more efficient nutrient delivery and increased stress tolerance in plants.
Key applications of nanotechnology in crop improvement include:
- Nano-fertilizers: These products enhance nutrient uptake efficiency, reducing the amount required and minimizing environmental contamination.
- Nano-pesticides: These formulations can target pests more effectively, resulting in reduced chemical use while maintaining crop protection.
- Disease Detection: Nanotechnology can lead to the development of quick, sensitive diagnostic tools, allowing for early detection of plant diseases.
The benefits of nanotechnology are significant, but it also raises concerns about environmental and health safety. The potential for bioaccumulation of nanoparticles in the food chain necessitates rigorous testing and regulation.
Technological advances in plant sciences, particularly remote sensing and nanotechnology, present an exciting frontier. Their influence extends beyond mere productivity; they fundamentally reshape agricultural practices towards sustainability and efficiency.
Ethical Considerations in Plant Research
Ethical considerations in plant research are paramount as they shape the trajectory of scientific inquiry and innovation. In the modern context, where biotechnology and genetic engineering play a prominent role, the ethical implications of these advancements cannot be overlooked. Examining these considerations helps researchers navigate complex moral landscapes, ensuring that their work benefits society while minimizing harm to the environment and biodiversity.
The significance of ethical evaluations extends to improving public trust in scientific endeavors. When researchers demonstrate a commitment to ethical practices, they foster stronger relationships with the community, encouraging transparency and understanding. This is essential, especially in fields like plant sciences where public perception can heavily influence policy and funding. Ethical considerations also guide the development of sustainable agricultural practices, ensuring that advancements do not compromise future generations.
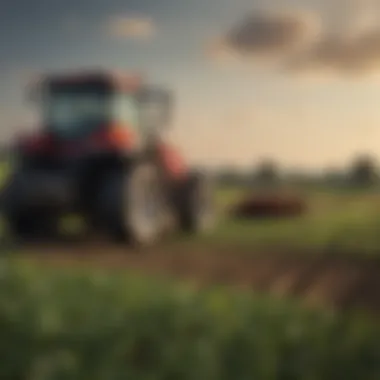
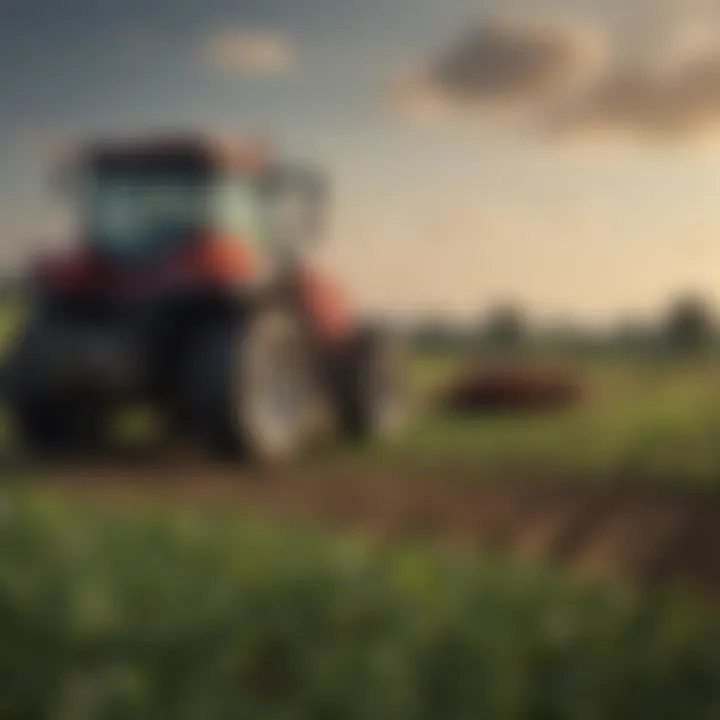
GMOs and Public Perception
Genetically modified organisms (GMOs) are often at the center of ethical discussions in plant research. These innovations hold potential for increasing crop yields and resilience against pests and diseases. However, the public perception of GMOs is frequently marred by controversy. While some view them as a solution to global food security, others express concerns about their safety and environmental impact.
Understanding public views is critical. Research has shown that transparency in the development and application of GMOs can lead to more positive perceptions. Educational initiatives that inform the public about the science behind GMOs and their benefits could mitigate fears.
Some key points regarding GMOs and public perception include:
- Public acceptance is often dictated by knowledge and awareness.
- Misinformation can lead to resistance against GMOs.
- Ethical practices in the research process enhance credibility.
- Trust is critical for encouraging the adoption of GMO technologies.
"Transparency and education are crucial to shaping public perception of GMOs, fostering informed discussions about their role in food security."
Biosafety Regulations and Compliance
Biosafety regulations are designed to protect human health and the environment from potential risks posed by genetically engineered plants. Compliance with these regulations is not only a legal requirement but also an ethical obligation for researchers and organizations involved in plant biotechnology. Understanding biosafety measures is essential for ensuring that innovations do not introduce unintended consequences into ecosystems.
Biosafety measures include rigorous testing of GMOs for ecological impact, assessment of potential risks associated with gene transfer, and monitoring for environmental changes. Some important aspects of biosafety regulations are:
- Risk assessment frameworks that evaluate the impact of GMOs.
- Post-market monitoring to track the effects of GMOs in agricultural settings.
- Collaboration between government agencies, scientists, and the public to develop effective regulations.
The ethical responsibility to comply with biosafety regulations highlights a commitment to responsible science. Such adherence not only protects biodiversity but also builds public confidence in plant research initiatives.
Conservation of Plant Biodiversity
Conserving plant biodiversity is crucial for many reasons. It plays a pivotal role in ensuring ecosystem stability and resilience, which in turn supports various ecological processes. Biodiversity contributes to services like pollination, nutrient cycling, and pest control, which are essential for agricultural productivity. With an increasing human population and the challenges posed by climate change, the conservation of plant biodiversity has become more pressing than ever.
Plant diversity also offers a treasure trove of genetic resources. This genetic variation is vital for food security. It allows for the development of new crop varieties that can withstand stresses such as drought, disease, and pests. Without genetic diversity, plants can become more susceptible to diseases and climate events that threaten food supplies.
Additionally, conserving plant biodiversity helps maintain cultural heritage and traditional practices linked to various plant species. Many communities rely on native plants for food, medicine, and livelihood. Protecting these resources is essential not only for ecological health but also for the cultural identity of these communities.
"The loss of biodiversity can result in the collapse of ecological systems that support human life."
Importance of Gene Banks
Gene banks are facilities that store seeds and genetic material from plants. They serve as a safeguard against the loss of plant species and genetic erosion. Gene banks play a critical role in the conservation of biodiversity. By preserving a wide variety of plant genetic material, they provide a resource for plant breeding programs aiming to develop resilient crops. This is particularly important in the face of climate change and the increasing need for stable food sources.
There are several key functions of gene banks:
- Preservation: Storing seeds in controlled conditions extends their viability.
- Research: Gene banks are centers for research on plant genetics and adaptability.
- Restoration: They can provide seeds for reintroduction into the wild where plant populations have declined.
Thus, gene banks are essential for genetic research and crop improvement. They act as a safety net for food crops that may face extinction due to various threats.
Restoration of Endangered Species
Restoring endangered plant species is a fundamental aspect of biodiversity conservation. Many plants face threats from habitat destruction, pollution, climate change, and invasive species. Restoration efforts aim to bring these plants back to their natural habitats or create new populations in areas where they have declined.
Effective restoration starts with a thorough understanding of the plant’s ecology and its habitat requirements. These initiatives often involve the following steps:
- Assessment: Identify the current status of the species and its habitat.
- Propagation: Grow plants in nurseries before reintroduction into the wild.
- Habitat Preparation: Ensuring the environment is conducive for plants to thrive.
- Monitoring: Continuously assess the success of restoration efforts.
Successful restoration not only helps in bringing back endangered species but also contributes to ecosystem recovery. It enhances biodiversity, providing habitats for various organisms and improving resilience against environmental changes.
In summary, conservation of plant biodiversity is vital. Gene banks and restoration of endangered species play important roles in this effort. Together, they support both ecological balance and food security.
Future Directions in Plant Sciences
The advancement of plant sciences holds profound implications for global challenges, such as food security and climate change. It is essential to consider various emerging trends and interdisciplinary collaborations to promote sustainable agricultural practices and biodiversity. This section will delve into the importance of these future directions and their role in transforming traditional agricultural methodologies.
Emerging Trends and Technologies
Emerging trends and technologies are shaping the landscape of plant sciences today. One of the most notable trends is the increased use of big data and artificial intelligence. These technologies allow researchers to analyze vast datasets in real-time, improving decision-making in plant breeding and resource management.
Precision agriculture is another important trend that enhances efficiency in farming. By utilizing drones and remote sensing, farmers can monitor crop health, soil conditions, and overall farm performance. This data-driven approach can lead to better yield and resource optimization.
In addition, the integration of genomics continues to revolutionize plant sciences. Techniques such as genome sequencing and marker-assisted selection are facilitating the development of crops that are more resistant to diseases and environmental stresses. These advancements are crucial as they have the potential to address global food shortages.
"The future of plant sciences lies in their ability to harness technology for innovative solutions that promise sustainability and resilience for food systems."
Lastly, the application of nanotechnology is gaining traction. This technique enhances crop protection strategies and nutrient delivery systems, allowing for the creation of more efficient fertilizers and pesticides.
Interdisciplinary Collaborations
Interdisciplinary collaborations are vital to the progress in plant sciences. By integrating knowledge from various fields such as biotechnology, environmental science, and policy making, researchers can develop holistic approaches to tackle pressing issues in agriculture.
For example, collaborations between plant biologists and engineers can result in the design of innovative agricultural tools that improve efficiency and reduce labor costs. Similarly, partnerships with environmental scientists can enhance our understanding of ecosystem interactions, leading to improved biodiversity conservation strategies.
Furthermore, collaborations with social scientists can address the socio-economic challenges associated with adopting new agricultural technologies. Understanding the perspectives of farmers and communities can guide the development and implementation of sustainable practices that are both effective and socially accepted.
In summary, addressing the future of plant sciences requires an emphasis on emerging trends like big data and precision agriculture, and fostering collaborations among diverse fields. It is through these synergetic efforts that we can hope to overcome the global challenges of food security and environmental sustainability.
Epilogue
The conclusion of this article synthesizes the critical exploration of plant sciences, emphasizing its increasing relevance in today's world. As global challenges like climate change and food security intensify, understanding the applications and advancements in plant sciences becomes paramount. This section highlights the significance of incorporating various methodologies and technologies, which serve as tools for fostering a sustainable future.
In evaluating the findings, it is clear that interdisciplinary approaches—such as the integration of biotechnology and environmental science—can lead to substantive improvements in agricultural practices and biodiversity conservation. The discussions throughout the article underscore that innovations in plant sciences not only enhance crop yields but also mitigate risks associated with plant diseases, ensuring a resilient food system.
Key components addressed within this conclusion include:
- The strengthening of food security through advanced agricultural techniques.
- The pivotal role of genetic engineering in establishing disease-resistant crops.
- The importance of sustainable practices that align with conservation efforts.
- The ongoing evolution of plant research that encourages adaptive strategies to cope with environmental changes.
Ultimately, this article illustrates that the future of plant sciences is intertwined with societal needs. As researchers and practitioners engage with these critical scientific advancements, they contribute to a landscape where agricultural productivity and environmental sustainability can coexist effectively.
"By bridging the gap between science and practical applications in agriculture, we pave the way for innovative solutions to pressing global issues."
Summary of Key Findings
The exploration of plant sciences revealed several key findings that encapsulate the article's overall message. The advancements uncovered are reshaping the future of agriculture and biodiversity. Notable points include:
- Technological integration: The merging of biotechnology and genomics has revolutionized crop improvement methods. Techniques such as CRISPR technology allow for precise genetic modifications, enhancing efficiency and resilience.
- Sustainable practices: The adoption of sustainable agriculture strategies—such as organic farming and permaculture—addresses both yield optimization and ecological balance, protecting essential ecosystems.
- Disease management: The rise in knowledge regarding plant pathology has led to the implementation of effective pest management systems, reducing dependency on harmful chemicals.
These findings collectively suggest that the trajectory of plant sciences is promising, driving forward innovations that foster global sustainability and food security.
Implications for Research and Practice
The implications derived from the key findings are extensive and hold significant weight for future research and practical applications within the field of plant sciences. Firstly, the emerging trends invite extensive interdisciplinary collaboration among scientists, agriculturalists, and environmentalists. Such collaborations enhance knowledge sharing and innovative approaches to complex issues.
Research focusing on the ethical considerations surrounding genetically modified organisms (GMOs) also becomes critical. As public perception varies widely, it is essential to address concerns through transparent communication and engaging dialogue. This ensures continued support for research that aims to impact food production positively and sustainably.
Moreover, the insights gleaned from advancements in plant sciences should inform educational practices within academic institutions. New curricula that incorporate cutting-edge technologies and sustainable practices can equip future generations with the necessary skills to thrive in this evolving field.