The Essential Role of Light in Microscopy
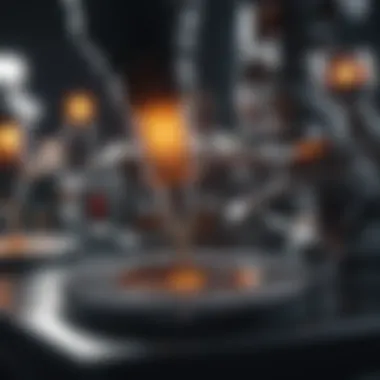
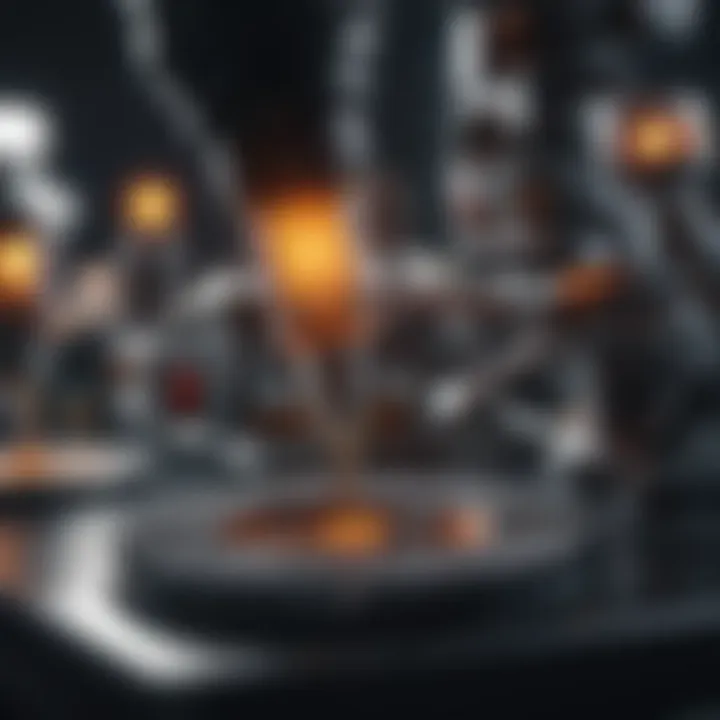
Intro
The world of microscopy begins with the steady glow of light, guiding scientists into the hidden realms of the microscopic universe. Light isn't just a bystander in this field; it plays a pivotal role, influencing how we perceive and understand the intricate details of our world at the cellular and molecular levels. In this article, we will explore the significance of light in microscopy, discussing various light sources, techniques, and their impact on scientific observation and research.
Research Overview
Summary of Key Findings
Illumination is an integral part of microscopy that can define the boundaries of visibility and clarity of the specimens being observed. From the humble bulb to sophisticated laser light sources, these tools enhance visibility by building contrast, which is essential for distinguishing intricate details within samples.
Key findings indicate that the choice of light source, along with optimal illumination techniques, can dramatically improve image quality and information yield in scientific research. For instance, LED-based illumination systems have emerged as a popular choice, offering longevity and versatility, not to mention energy efficiency, which aligns well with modern scientific practices.
Importance of the Research
The implications of light in microscopy extend beyond mere observation. This exploration can revolutionize our understanding in numerous fields, such as biology, materials science, and even medicine. Enhancing microscopy techniques can lead to breakthrough discoveries, such as the identification of cellular structures or even diagnosing diseases at early stages.
Underlying these understandings are several principles related to optics and light behavior. Illuminating our studies of light leads not just to clearer images, but also to deeper insights into the very nature of life and matter.
Methodology
Study Design
The design of this exploration includes a systematic review of existing literature on light sources integrated into various microscopy techniques. Key terms related to illumination mechanics, microscopy applications, and recent technological advancements in light sources have been thoroughly analyzed in order to gather a comprehensive understanding of current practices.
Data Collection Techniques
Data was collected through a combination of scholarly articles, experimental reviews, and case studies, focusing on the applications of different lighting technologies in microscopy. The intent has been to compile a thorough overview that reflects both historical context and contemporary innovations.
"Without light, we would be limited to a dull existence, blind to the intricate designs of nature that lie beneath the surface." In this endeavor, essential keywords such as light microscopy, illumination types, and optical advancements have naturally found their way into the discourse, ensuring a well-rounded analysis.
As we venture further into this narrative, we will explore specific lighting techniques, their effectiveness, and the types of microscopy that benefit most from these enhancements.
Preface to Microscopy
Microscopy acts as a bridge to a realm that remains largely unseen by the naked eye. It transforms the abstract notion of the microscopic world into a practical exploration, revealing intricate details of structures and organisms that populate our planet. The significance of microscopy cannot be overstated; it has paved the way for groundbreaking discoveries in various fields such as biology, medicine, and material science. By shedding light on these microscopic wonders, researchers can deepen their understanding of life processes, develop innovative treatments, and even enhance the quality of materials.
In this section, we will delve into the definition and importance of microscopy, as well as explore its historical evolution, which has shaped the way we approach scientific inquiry.
Definition and Significance
At its core, microscopy refers to the science of observing objects that are beyond the resolution of the naked eye, typically smaller than roughly 200 micrometers. It involves using various types of instruments, commonly known as microscopes, to magnify these objects. The significance of microscopy lies in its ability to illuminate the details of life, allowing scientists to examine cells, bacteria, and even viruses.
The benefits of microscopy are manifold:
- Enhanced Visibility: With the right light and optics, even the smallest structures can appear vividly, aiding in analysis and recognition.
- Research Advancements: Many scientific advancements hinge on the ability to see at micro or nano scales. The study of diseases, cellular behavior, and materials relies heavily on microscopy.
- Targeted Applications: Specific microscopy techniques can be tailored to various fields, from biology to nanotechnology, allowing researchers to probe specific questions in their domains.
Historical Background
The evolution of microscopy is a story steeped in innovation and discovery. It began in the late 16th century when the first simple microscopes were crafted by pioneers like Zacharias Janssen and Hans Lippershey. These early devices, with their rudimentary lens systems, opened the door to observations that would have otherwise been impossible.
However, it was not until the 19th century that microscopy underwent transformative advancements:
- Compound Microscopes: Introduced by Antonie van Leeuwenhoek, these offered greater magnification and clarity, revolutionizing the study of biology.
- Theories and Techniques: The development of the optical theory and improved lenses allowed for the exploration of different wavelengths of light, providing deeper insights into specimen structures.
- Modern Era: The 20th century ushered in sophisticated technologies such as electron microscopy and fluorescence microscopy, which continue to expand the boundaries of what we can observe and understand in the microscopic realm.
"Microscopy is not merely an observational tool but a gateway to understanding the intricate dance of life at a level we could only dream of before."
With this foundational knowledge, we set the stage for exploring the illuminating role of light in microscopy. In the coming sections, we will delve into the fundamental principles that govern light, the various types of light sources used in microscopy, and the optical schemes that enhance our observations.
Fundamental Principles of Light
In the realm of microscopy, understanding the fundamental principles of light is not just academic; it's essential for anyone looking to navigate the microscopic world. Light is the cornerstone of microscopy, transforming obscured structures into visible forms that are crucial for scientific exploration. Knowing how light behaves, its various properties, and the interplay it has with materials lays a solid foundation for effective specimen observation. This section discusses how these principles enhance resolution and contrast, making it possible to discern details that would otherwise remain hidden.
Nature of Light
Light is often thought of simply as a means to illuminate, but its essence is far more intricate. Light behaves as both a wave and a particle, exhibiting properties that can be surprising. This duality leads us into the fascinating world of optics, where everything from colors to reflections comes into play. Light travels in waves at remarkable speeds, around 299,792 kilometers per second in a vacuum. When this light passes through various mediums, such as glass or water, it can bend, change, or even split, as seen in prisms.
- Wavelengths and Colors: Each color we see corresponds to a specific wavelength. The visible spectrum corresponds to wavelengths from about 400 to 700 nanometers, with purple light at the low end and red at the high end.
- Reflection and Absorption: When light hits a surface, some may bounce back, while others are absorbed. This interaction can significantly alter how we perceive a specimen under a microscope. Understanding the nature of light allows scientists to tailor their observation methods accordingly.
In a practical sense, knowledge about light’s nature aids in selecting appropriate illumination techniques and reduces the chances of misinterpretation of specimens due to improper lighting conditions.
Wave-Particle Duality
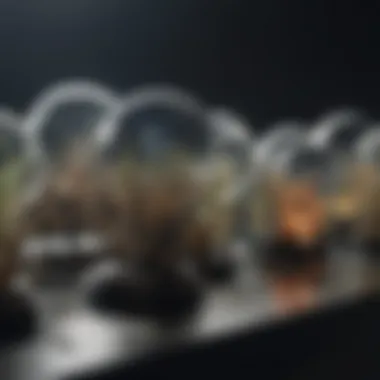
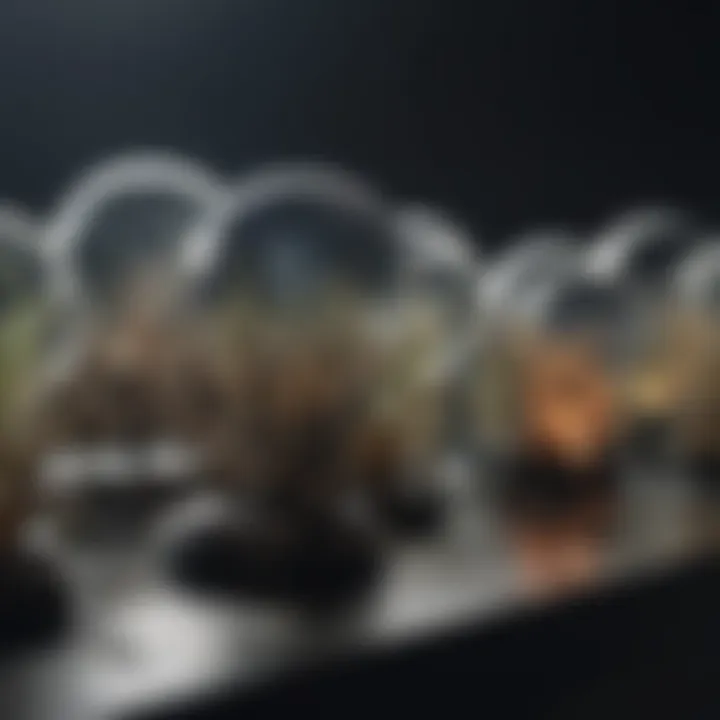
The concept of wave-particle duality is perhaps one of the most mind-bending elements in physics, and it holds pivotal significance in microscopy too. Light, at times, behaves like a continuous wave, while at other times, it acts as discrete packets or particles called photons. This duality is crucial for understanding how microscopes work.
"Light behaves as both a wave and a particle, leading to incredible opportunities in imaging and observation."
- Wave Properties: Common wave properties like interference and diffraction are vital in microscopy. These phenomena can allow multiple light beams to interact, creating interference patterns that yield enhanced imaging capabilities.
- Particle Properties: When considering light as photons, we recognize that these particles can excite electrons in atoms, causing fluorescence, a technique widely exploited in microscopy to highlight specific structures within cells.
Thus, the wave-particle nature of light informs the design and use of specific microscopy techniques. For instance, high-resolution imaging often leverages the wave aspect, while fluorescence microscopy capitalizes on the particle characteristic to selectively illuminate certain components of a specimen.
Understanding these principles not only increases the effectiveness of microscopy but also broadens the horizon for innovative research applications, such as those found in biology or material science.
Types of Light Sources in Microscopy
The significance of light sources in microscopy cannot be overstated. The type of light used in microscopy plays a critical role in how specimens are observed, characterized, and understood. Different light sources provide varying wavelengths, intensities, and qualities of illumination, all of which can affect the final image. Furthermore, understanding the nuances of each type of light source is essential for optimizing visibility, enhancing contrast, and allowing detailed analysis of microscopic structures. This section dives into some common types of light sources, their benefits, drawbacks, and specific applications in microscopy.
Incandescent Lamps
Incandescent lamps, often the most traditional light source in microscopy, produce light by heating a filament within a glass bulb. As electricity flows through the filament, it heats up to a point where it emits light. This method provides a warm, continuous light spectrum; however, it has some significant downsides.
- Spectral Quality: The light spectrum of incandescent sources skews towards the red-orange region, which can limit the contrast in certain samples.
- Heat Generation: These lamps generate substantial heat, which can affect temperature-sensitive specimens. Researchers must be cautious with their use.
- Longevity: They burn out relatively quickly, requiring frequent replacement, which could be cumbersome in long-term experiments.
While incandescent lamps laid the groundwork for many earlier microscopy techniques, their usage has largely shifted due to the emergence of newer technologies.
Fluorescent Lights
Fluorescent lights operate on a different principle. They utilize a gas-filled tube coated with phosphorescent material. When high-voltage electricity passes through this gas, it emits ultraviolet light, which in turn excites the coating. This excitement results in the emission of visible light.
- Enhanced Visibility: Fluorescent illumination allows for significant contrast, making it easier to identify specific components in a sample, especially when stained with fluorescent dyes.
- Lower Thermal Output: Compared to incandescent lamps, fluorescent lights generate less heat, making them more suitable for living specimens.
- Longevity and Cost-Effectiveness: These lamps have a longer lifespan and are more energy-efficient, leading to lower operational costs.
While fluorescent lighting has its share of strengths, proper filters must be used to balance the excitation and emission spectra effectively.
LED Technology
Light Emitting Diodes (LEDs) represent a modern advancement in microscopy illumination. They are compact, energy-efficient lights that can be tuned to emit specific wavelengths. This tunability is particularly advantageous in various microscopy techniques.
- Versatile Emission: LEDs can be designed to emit different colors tailored for specific applications and dyes, enhancing observational capabilities.
- Low Heat Production: Similar to fluorescent lights, LEDs produce minimal heat, thus preserving sample integrity and extending the life of living specimens.
- Extended Lifespan: LEDs can last significantly longer than traditional incandescent lamps, which reduces replacement frequency and costs over time.
Although they may come with a higher initial investment, the benefits of LEDs often outweigh these costs in high-end microscopy applications.
Laser Light Sources
Laser-based light sources are distinguished by their coherence, monochromaticity, and high intensity. These unique properties make lasers exceptionally powerful tools in advanced microscopy techniques.
- Precise Focusing: The parallel nature of laser light allows for high-resolution imaging and the ability to focus on minute details in specimens without scattering, making lasers a go-to choice for techniques like confocal microscopy.
- Selective Excitation: Lasers can be tuned to specific wavelengths for selective excitation of fluorescent dyes, which enhances the quality of the resultant images.
- High Intensity and Depth Penetration: Laser light can penetrate deeper into samples than conventional illumination, facilitating 3D imaging.
While lasers offer incredible capabilities, they often require careful calibration and may have complexities in operation compared to simpler light sources.
Ultimately, the choice of light source significantly impacts the outcome of microscopic examinations. Each type has its own set of advantages and challenges, and understanding these nuances equips researchers and educators to select the appropriate methods for their specific inquiries.
Understanding the capabilities of different light sources is key in microscopic research. This knowledge ensures that scientists can capture the finest details of life at the cellular level.
Optical Schemes in Microscopy
Optical schemes form the backbone of microscopy, enabling scientists to observe specimens that would otherwise remain hidden to the naked eye. The choice of optical scheme significantly influences the quality of images produced and enhances the capability to discern minute details in a sample. A well-chosen optical scheme not only improves contrast and resolution but also aids in the accurate interpretation of biological structures and materials. Moreover, understanding these schemes is essential for researchers looking to adapt their techniques to specific scientific inquiries, ensuring that they get the best possible images from their light sources.
Bright-Field Microscopy
Bright-field microscopy is perhaps the most straightforward technique and is widely employed in laboratories. In this method, the light passes through the specimen, which can absorb or scatter light to varying degrees. As a result, areas of the sample appear darker than the bright background. One of the strengths of this method is its ease of use and the familiarity it brings to beginners in the field. However, it comes with a notable downside: the requirement for staining most specimens to produce adequate contrast. This approach could lead to alterations in the sample's natural setting, raising potential concerns about the validity of the observations made.
Bright-field microscopy presents a fundamental and accessible entry point into the world of microscopy, offering significant insights despite its limitations.
Dark-Field Microscopy
Dark-field microscopy creates a unique imaging technique that differs fundamentally from bright-field methods. This approach restricts direct light from entering the objective, allowing only scattered light to be viewed. The result is a strikingly illuminated specimen against a dark background, enhancing contrast significantly. This method is particularly advantageous for observing living cells, microorganisms, or samples that are difficult to stain. Its ability to provide information about the morphology and the internal structure of the specimen can be invaluable in certain types of biological research, especially when studying motility or dynamic processes.
Phase Contrast Microscopy
Phase contrast microscopy is a technique designed to utilize the differences in the refractive indices of various structures within the specimen. It converts these phase shifts in light into variations in brightness and contrast in the final image. This method is particularly useful for observing live cells and transparent specimens without staining, offering more natural views of cellular structures. The subtle details captured using this technique are ideal for studying cellular components such as organelles, allowing researchers to observe dynamic processes, such as cell division or nutrient uptake, in real time.
Differential Interference Contrast Microscopy
Differential interference contrast microscopy (DIC) takes advantage of the interference of light waves to enhance details in a specimen. DIC adds another layer of sophistication by employing two beams of polarized light that are slightly out of phase. This approach gives a 3D appearance to specimens, making it easier to visualize fine structures without staining. It finds extensive application in observing live specimens, particularly in developmental biology and virology. The technique excels at revealing details and structures that would be lost in traditional bright-field setups, offering more insight into the underlying biology of the sample.
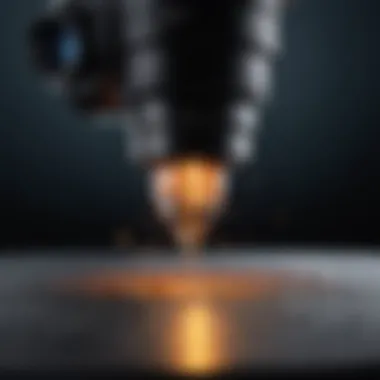
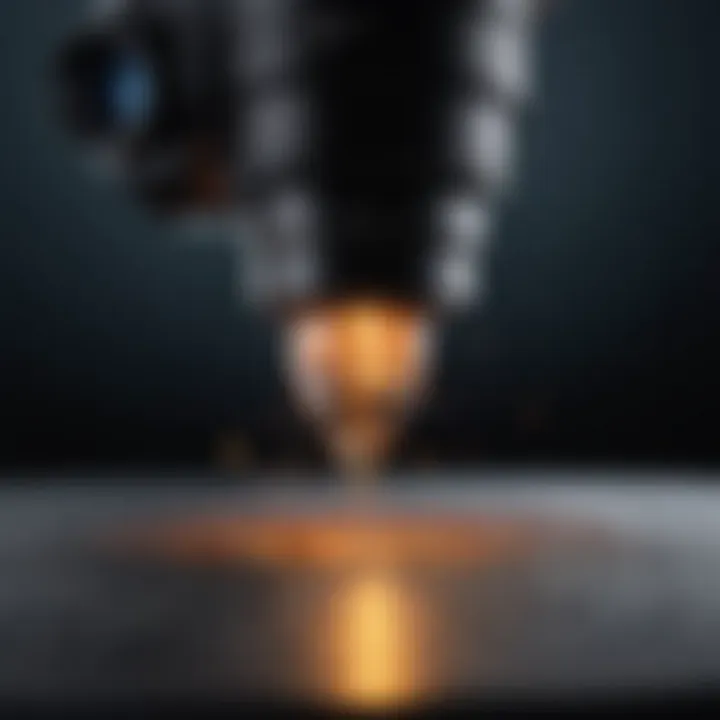
In summary, various optical schemes provide tailored solutions for microscopy, each with its own strengths and weaknesses. Understanding these methods and their implications makes a significant difference in the quality of observations and the conclusions drawn in scientific research.
Light Interactions with Specimens
Understanding how light interacts with specimens is paramount in microscopy as it directly affects the quality of the images produced and the information gleaned from them. Light doesn't merely illuminate; it dances through and around specimens, creating a vivid tapestry of interaction, revealing structures invisible to the naked eye. Grasping these interactions enhances our ability to utilize various light sources and techniques to their full potential.
Refraction and Reflection
Refraction and reflection are fundamental principles that govern how light behaves when passing through different mediums. When light enters a new medium, such as going from air into glass or liquid, it bends, or refracts. This bending of light is critical when using microscopes, as lenses are designed to focus and direct this refracted light to enhance the image clarity.
- Refraction: Light travels slower in denser mediums. Microscopy exploits this phenomenon through various lens designs to magnify specimens effectively. High-quality lenses, like those found in Nikon’s microscopes or Zeiss optics, use refraction for precise imaging, noting how most biological specimens are submerged in a bath of immersion oil. The refractive index of this oil closely matches that of glass, reducing light scattering, thus enabling a clearer view of the sample.
- Reflection: This occurs when light bounces off a surface. In microscopy, reflecting light can provide contrasting details, especially in dark-field microscopy where unscattered light provides a bright image against a dark background. It’s a nifty trick to enhance low-contrast specimens, revealing structures that might otherwise fade into invisibility.
"By understanding the angles and conditions of light's interaction with different materials, one can fine-tune observations and unlock a depth of detail in microscopy that remains hidden to the untrained eye."
Absorption and Transmission
Absorption and transmission are two sides of the same coin, dictating how much light penetrates a specimen versus how much is absorbed, affecting the resultant coloration and visibility of specimens in microscopy.
- Absorption: This is when light energy is taken up by the specimen, causing it to appear opaque or colored depending on the material properties. Biological specimens often absorb certain wavelengths of light more effectively, giving researchers critical insights, especially when using stains or dyes that enhance target structures within cells.
- Transmission: This is particularly crucial in transmission microscopy where light is allowed to pass through the sample. The thicker or denser a specimen, the more it can attenuate transmitted light, leading to varying levels of brightness in the resulting image. Adjustments in light intensity can counterbalance this, enhancing clarity and resolving power.
Breaking down these interactions—refraction bending light to show detail, reflection unveiling hidden aspects, absorption coloring specimens, and transmission revealing transparency—illustrates how adeptly microscopy harnesses light to bridge the observable and the unseen. Ignoring any of these processes would be like using a hammer where a scalpel is needed; understanding the purpose and mechanism is key to effective microscopy.
Enhancing Contrast and Resolution
In microscopy, contrast and resolution play crucial roles in how we perceive and analyze specimens. Enhancing these two aspects not only aids in distinguishing subtle details but also enriches our overall understanding of the microscopic world. When researchers delve into the minutiae of biological structures or materials, having clarity in imagery becomes essential—it allows for precise measurements and an accurate interpretation of results.
The Role of Stains and Dyes
Stains and dyes are indispensable tools in microscopy, allowing scientists to increase contrast, thereby making specific features of biological samples more visible. Different stains target particular biological structures, highlighting them against a contrasting background. For instance, methylene blue stains cellular components, making it easier to identify structures like the nucleus. This contrast is especially important when examining transparent specimens, which might otherwise appear nearly invisible under standard illumination.
The choice of stain can greatly affect the quality of the image obtained. Some dyes, like hematoxylin, interact with cellular components to enhance the visual differentiation between various types of tissues, which can be vital in pathology. Choosing the correct stain requires a deep understanding of both the specimen and the staining process itself, as well as the desired outcome. It is worth noting that over-staining can lead to artifacts, confusing interpretation.
"A good stain can transform the way we see the world at the micro level, providing insights that the naked eye cannot perceive."
The application process itself—whether using dropper methods or immersion—can influence the final results, which adds another layer of complexity. Thus, while stains and dyes significantly enhance contrast, the subtleties of their application and choice merit careful consideration.
Image Processing Techniques
With advancements in technology, image processing techniques are paving new avenues in the realm of microscopy. These techniques enhance image quality and detail far beyond what traditional light microscopy methods could achieve alone. Post-capture processing allows researchers to adjust brightness, contrast, and sharpness, which can highlight features previously overlooked.
Some common image processing techniques include:
- Noise Reduction: This process minimizes graininess that can obscure details, leading to cleaner, sharper images.
- Image Stitching: This combines multiple images to create a panoramic view of a specimen, perfect for larger samples or when high detail across a wide field is needed.
- Deconvolution: Used to improve the resolution of images, this technique counters blurring caused by light scattering.
The software available for performing these techniques has greatly expanded in recent years. Programs like Fiji or ImageJ provide robust platforms for both amateur and professional users to enhance their images. However, user familiarity with these tools is vital; improper handling may yield misleading images, which can hinder scientific conclusions rather than aid them.
In essence, integrating staining methods with sophisticated image processing not only magnifies the clarity of observations but also enhances the understanding of intricate structures. Each layer of enhancement, from chemical interactions of dyes to algorithmic adjustments in images, comes together to enrich the scientific narrative we glean from the microscopic world.
Applications of Lighting Techniques in Microscopy
The study of microscopy wouldn’t be where it is today without the application of various lighting techniques. The importance of effectively illuminating samples cannot be overstated. By employing specific lighting methods, researchers can observe intricate structures and processes within biological samples and materials.
One significant benefit of advanced lighting techniques is the enhancement of contrast, enabling scientists to differentiate between various components of a specimen. Additionally, the use of tailored lighting can aid in minimizing the background noise, while still highlighting essential features for analysis. As technology progresses, the applications of these lighting techniques expand, bringing forth new capabilities essential for modern research.
Applications in Biological Research
Biological research harnesses microscopy and its lighting techniques to explore life at the microscopic level. Whether investigating cellular structures or observing microorganism behaviors, the implications are vast. For instance, fluorescence microscopy allows researchers to visualize specific components of cells by using fluorescent stains that emit light at a particular wavelength, effectively highlighting cellular processes in real time.
Moreover, in pathology, techniques like bright-field and dark-field microscopy help pathologists observe changes in tissue samples, aiding in the early detection of diseases. This method is notable for its ability to enhance contrast without a considerable increase in exposure times, reducing the risk of light-induced damage to sensitive specimens.
"The quality of the light source directly influences the reliability of the results in biological microscopy."
In essence, biological research has greatly benefited from innovative lighting applications.
Applications in Material Science
Microscopy plays an instrumental role in material science, allowing researchers to analyze the structure and properties of various materials at an atomic and molecular level. Here, the choice of lighting makes a world of difference. For example, polarized light microscopy (PLM) is a technique that reveals the optical properties of anisotropic materials. It’s particularly useful for examining crystals or polymers.
Additionally, scanning electron microscopy (SEM) paired with advanced illumination techniques helps material scientists observe surface topography and composition in great detail. The utilization of variable pressure conditions also allows researchers to visualize insulative and beam-sensitive specimens without altering their intrinsic properties.
As we progress in the field of material science, the continuous evolution of lighting technologies invites endless new possibilities for exploration and material characterization.
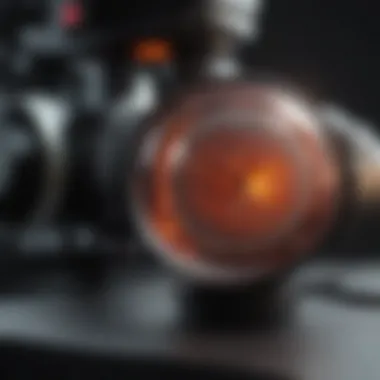
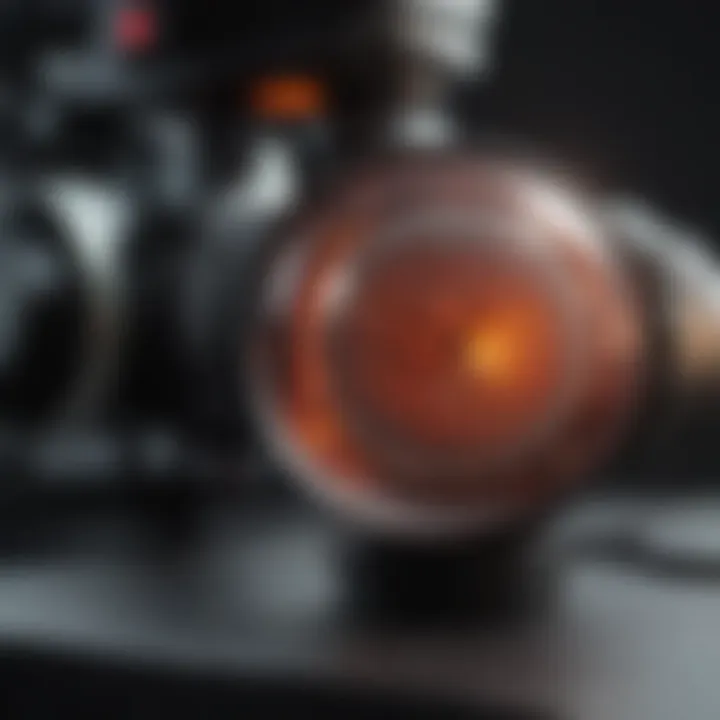
In both biological and material sciences, lighting techniques do not just illuminate; they enlighten understanding of complex phenomena, ultimately driving innovation and discovery.
Recent Advances in Microscopy Lighting
The rapid evolution of microscopy lighting technologies has transformed how scientists interact with the microscopic world. The significance of keeping pace with these advances is crucial, as they can open new avenues for understanding complex biological processes and material properties. With enhanced visualization capabilities, researchers can now observe fine details of specimens that were once hidden from view.
Advancements have yielded benefits including better resolution, increased contrast, and the capability to monitor dynamic processes in real-time. However, these improvements come with their own set of challenges and considerations that must be addressed to fully utilize the potential of these new methodologies.
Super-Resolution Techniques
Super-resolution microscopy has taken center stage, challenging traditional limitations of optical microscopy. Techniques such as Stimulated Emission Depletion (STED) and Structured Illumination Microscopy (SIM) have fundamentally altered the landscape of how we perceive objects at the nanoscale. These methods allow researchers to achieve resolutions beyond the classical diffraction limit, making it possible to discern structures at the molecular level.
For instance, consider a scenario in biological research where scientists examine protein interactions within a cell. Conventional microscopy might only hint at proximity, but super-resolution techniques can illuminate precise spatial arrangements, leading to enhanced understanding of cellular mechanisms. The implications of this are profound; deeper insight into biological functions can drive innovations in drug development and disease treatment.
"Super-resolution microscopy offers a 'window' into the nanoscale, revealing details we previously couldn't fathom."
While the benefits are enticing, implementing super-resolution techniques often demands sophisticated instrumentation and expertise. Moreover, sample preparation can be intricate, as certain staining techniques must be employed to make desired structures visible. Researchers need to weigh the cost and complexity against the invaluable details these methods provide.
Live Cell Imaging
Live cell imaging represents another paradigm shift in microscopy. Unlike traditional methods that often involve fixing or killing cells, live cell imaging permits observation of biological processes in real time. This is particularly advantageous for studying cellular behavior under physiological conditions. One crucial aspect here is the ability to track cellular dynamics—such as cell motility, division, and interaction with other cells—in their natural environment.
Various lighting techniques, including fluorescence microscopy and total internal reflection fluorescence (TIRF), are harnessed to minimize phototoxicity while maximizing signal clarity. For example, researchers studying cancer cells can monitor drug responses live, drawing conclusions about efficacy and understanding resistance mechanisms. While the insights gained here can shape future therapeutic approaches, the complexity of imaging live cells presents challenges related to maintaining sample viability and avoiding light-induced damage.
The continuing evolution of live cell imaging techniques is essential for advancing our understanding of various phenomena, from the mechanics of cell migration to the lifecycle of infectious agents.
In summary, the strides made in super-resolution techniques and live cell imaging not only enhance our capabilities in microscopy but are also paving the way for groundbreaking discoveries. As we look to the future, balancing technological advancements with the ethical and practical considerations will be key in leveraging microscopy for scientific exploration.
In this ever-evolving field, addressing the challenges while harnessing the full capabilities of new lighting technologies holds the promise of revolutionizing research methodologies across disciplines.
Challenges and Considerations
In the intricate world of microscopy, the challenges associated with lighting techniques are as significant as the illumination provided itself. This section aims to explore the potential pitfalls and considerations that researchers, students, and educators must account for when utilizing light in microscopy. By understanding these challenges, one can refine both techniques and results, leading to more reliable and insightful observations.
Thermal Effects on Samples
Thermal effects constitute a key challenge in the field of microscopy. The heat generated by light sources, especially those with high intensity like lasers or incandescent bulbs, can impose serious risks to delicate samples. When exposed to excessive heat, specimens—be they biological or material—can undergo thermal alteration, affecting their structure and integrity. This same energy transfer that allows for detailed imaging can also lead to unwanted destruction.
It's essential to consider the following aspects:
- Sample Sensitivity: Certain types of cells, like live organisms, are particularly vulnerable to temperature fluctuations. Even slight increases in temperature can induce stress responses or cause cell death.
- Duration of Exposure: Prolonged exposure to light sources can aggravate thermal effects. The longer a specimen is under observation, the more it risks damage from heat.
- Cooling Mechanisms: Utilizing cooling stages can effectively mitigate these risks. Implementing techniques to isolate the sample from direct light or using external cooling systems helps in maintaining optimal conditions.
To manage thermal effects, it's imperative for researchers to select appropriate light sources and adapt their techniques based on the unique nature of their specimens. Keeping the thermal load in check translates to more accurate readings and preserves the quality of the research.
Light-Induced Damage
Another critical factor to consider is light-induced damage, which can manifest in various forms depending on the type of specimen and light source used. This term refers to the physical and chemical alterations that occur in samples as a result of exposure to prolonged or intense light. Such damage is not limited to living cells; it includes materials as well.
Several key points highlight the importance of acknowledging light-induced damage:
- Phototoxicity: In biological research, excessive light can lead to phototoxicity, causing bacteria and cells to malfunction or die. It’s particularly concerning in live cell imaging, where maintaining cell viability is paramount.
- Fluorescence Quenching: Over time, certain fluorescent dyes degrade when exposed to light, leading to a reduction in signal intensity. Monitoring the lifespan of these dyes under specific illumination conditions is crucial for reliable results.
- Chemical Reactions: Some samples may undergo unintended chemical reactions upon exposure to light. For instance, photodegradation can affect polymers, metals, and other materials, altering their composition and structural properties.
Addressing light-induced damage requires careful planning and consideration. Researchers should:
- Opt for low-power illumination techniques where feasible.
- Use optimal exposure times and find a balance between visibility and integrity.
- Explore alternative imaging methods to reduce reliance on intense light.
Future Directions in Microscopy Lighting
As the landscape of scientific research evolves, so too does the need for innovative lighting solutions in microscopy. The future of microscopy lighting holds significant promise, offering not just enhancements in illumination techniques, but also paving the way for new discoveries across various disciplines. Advances in this field can lead to improvements in imaging clarity, specimen viability, and overall research outcomes. This section aims to illuminate these emerging prospects, underscoring their importance in elevating microscopic examination and analysis.
Emerging Technologies
The realm of microscopy lighting is witnessing a surge of novel technologies that are transforming how researchers visualize specimens. One standout advancement is the development of adaptive optics. This technique allows for the compensation of optical aberrations, thus improving image quality. Microscope setups employing adaptive optics can fine-tune the focus and enhance resolution even when observing live specimens.
Another critical area is multi-photon microscopy. Utilizing simultaneous excitation from multiple photons, this technology enables deeper imaging of thick biological tissues with minimal damage to the samples. Coupled with new light sources like ultrafast lasers, researchers get unparalleled visibility into subcellular structures.
Moreover, integrating machine learning with imaging technology is becoming increasingly relevant. Algorithms can analyze massive datasets for anomalies or patterns that are often overlooked by the human eye. This capability not only saves time but also allows for real-time adjustments in imaging protocols based on findings.
In summary, emerging technologies in microscopy lighting are not merely innovations; they represent crucial steps towards decoding life's complexities in ever finer detail. The impact of these technologies stretches far beyond the lab bench, influencing medical diagnostics, environmental science, and even materials research.
Interdisciplinary Approaches
The pursuit of advancements in microscopy lighting does not exist in a vacuum. Interdisciplinary collaboration is key to unlocking the full potential of these innovations. For instance, partnerships between physicists and biologists have led to more effective light sheet microscopy, which minimizes photodamage while providing high-resolution images of living specimens.
Additionally, insights from chemistry play a vital role in the formulation of fluorescent markers that can enhance specificity and contrast in imaging. These dyes and stains, often developed through collaborations with chemical engineers, allow researchers to visualize specific cellular components, improving the depth of information gathered during microscopy.
Furthermore, the integration of computer science and data analytics into microscopy is forging pathways for enhanced data management and visualization techniques. As imaging technologies produce increasingly large datasets, the need for sophisticated software solutions becomes paramount. This necessitates a team of experts from various fields working together to create effective platforms for analyzing and displaying complex data in an intuitive manner.