Single Cell DNA Extraction: Methods and Future Directions
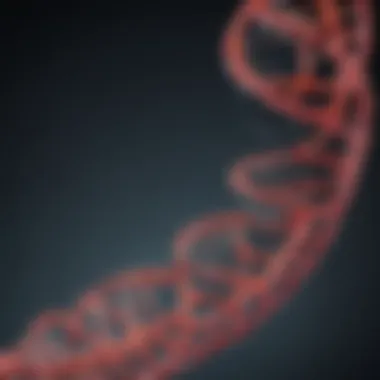
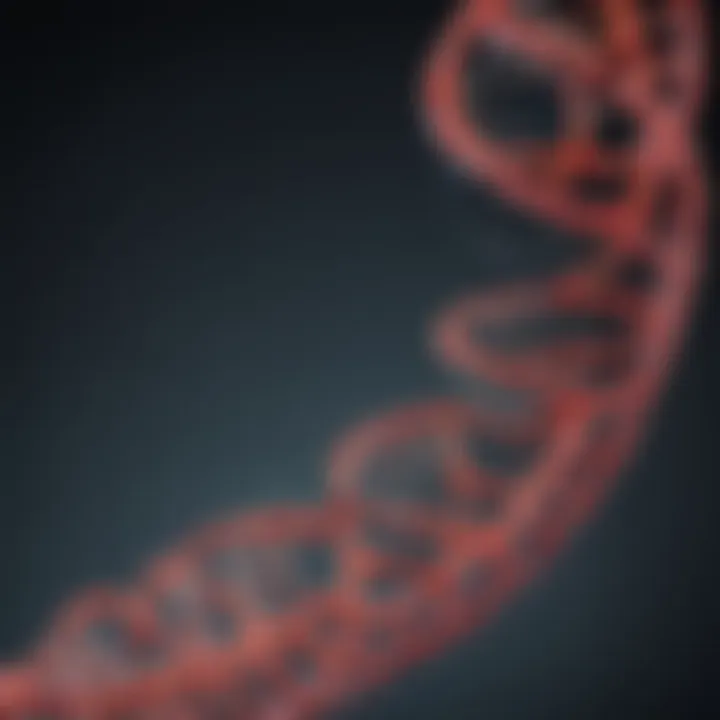
Intro
Single cell DNA extraction is an innovative frontier in molecular biology. The ability to analyze DNA at the single-cell level has significant implications for understanding genetic diversity and complex diseases. As the demand for precision medicine and tailored treatments grows, the extraction and analysis of DNA from individual cells emerges as a crucial technique. Researchers and biologists are increasingly turning to these methods to decipher genetic information that varies across cells, providing insights into cellular functioning and disease mechanisms.
Research Overview
Summary of Key Findings
Single cell DNA extraction techniques have evolved rapidly. Studies show different methodologies like micro-manipulation and laser capture microdissection yield quality results. These methods allow researchers to extract DNA from a single cell with minimal contamination and degradation. It has been identified that single cell analysis reveals heterogeneity in populations of cells that standard bulk analysis may overlook.
Importance of the Research
The significance of this research lies in its applications within genomics and personalized medicine. By understanding genetic variations on a single-cell level, scientists can identify mutations that contribute to diseases such as cancer. This unlocks the potential for targeted therapies that are more effective and tailored to individual patients. Ultimately, it advances our understanding of not just genetics but also personalized healthcare approaches.
Methodology
Study Design
The studies performed in the realm of single cell DNA extraction typically utilize a variety of approaches depending on the goal of the research. Researchers often select methods based on their specific requirements, including throughput, sensitivity to low DNA quantities, and the type of analysis intended.
Data Collection Techniques
Data collection techniques involve various extraction protocols. Methods such as whole genome amplification (WGA) can help generate enough DNA from single cells for downstream analysis. Furthermore, advanced sequencing technologies are employed to examine the extracted DNA. These tools provide comprehensive insights into the genetic makeup of the cells being studied.
The advancements in single cell DNA extraction not only improve our understanding of genetic variance but also pave the way for novel therapeutic interventions.
Prologue to Single Cell DNA Extraction
Single cell DNA extraction is a foundational approach in the field of genomics and molecular biology. This method allows researchers to analyze genetic information from individual cells, leading to enhanced understanding of biological variability. It plays a crucial role in advancing personalized medicine, cancer research, and developmental biology.
In today's scientific landscape, the ability to isolate DNA from single cells highlights the complexity and diversity of cellular populations. Traditional methods often analyzed bulk samples, masking essential insights present at the single-cell level. Therefore, the importance of single cell DNA extraction cannot be overstated, as it provides new opportunities for precision in research strategies.
Definition and Importance
The term 'single cell DNA extraction' refers to the techniques used to isolate DNA from individual cells. It stands out from conventional extraction methods that often overlook the unique genomic variations present in distinct cells. The importance of this technique lies in its potential to unveil
- Genetic diversity: It helps to identify and characterize genetic differences that may influence health and disease.
- Disease mechanisms: Understanding how various cells contribute to diseases can facilitate targeted therapies.
- Personalized medicine: Individualized treatment strategies can be developed by analyzing the unique genetic makeup of patients.
Given these advantages, the significance of single cell DNA extraction continues to grow within both academic and clinical settings.
Historical Context
The development of single cell DNA extraction techniques has evolved significantly over the past few decades. Initially, researchers worked primarily with bulk samples, relying on average genetic representations for analysis. This approach proved limiting, as it ignored the heterogeneity found in tissues and cell populations.
The breakthrough came with advances in technology, particularly microfluidics and laser capture microdissection, which allowed for the isolation of individual cells without contamination. The characterization of human genomes has further propelled interest in single cell sequencing methods.
These historical advancements underscore the transformative impact of single cell DNA extraction on research methodologies, moving from traditional techniques to sophisticated approaches capable of addressing the complex questions in biology today.
Applications in Research
Single cell DNA extraction is at the forefront of various research applications, reshaping our understanding of
- Cancer biology: By isolating DNA from tumor cells, researchers can explore genetic mutations that drive tumor progression.
- Stem cell research: Investigating the genomic characteristics of stem cells can lead to breakthroughs in regenerative medicine.
- Neurobiology: Studying individual neurons aids in uncovering the genetic underpinnings of neurological disorders.
Each of these applications illustrates how single cell DNA extraction provides critical insights that can lead to innovative therapies and a better understanding of biological processes.
Essential Principles of Single Cell DNA Extraction
The principles of single cell DNA extraction are a foundational aspect of understanding how this technology operates in the broader context of genomics. These principles guide the methodology and application of single cell techniques, making it essential to grasp their implications in research and clinical settings. Single cell DNA extraction allows researchers to analyze genetic material from individual cells, which can reveal variations that are otherwise hidden in bulk analysis. The insights gained from this process are valuable for comprehending complex biological systems and disease mechanisms.
Cellular Integrity and Viability
Cellular integrity refers to the preservation of cellular structure and components during the extraction process. This is crucial for ensuring that the DNA collected is representative of the original cell’s genetic material. Maintaining cellular viability is equally important because damaged cells can release contaminants that may interfere with downstream applications and analyses.
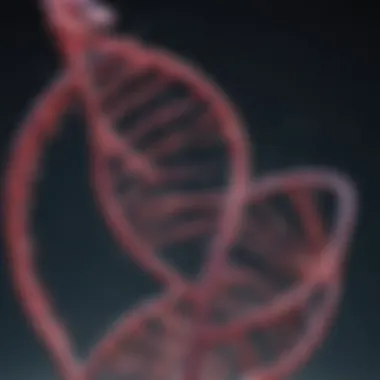
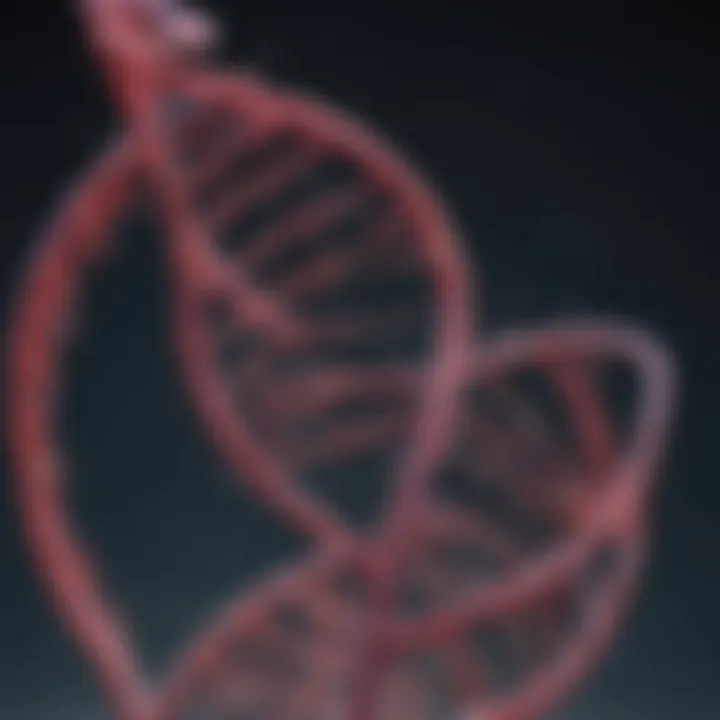
Key aspects to consider in maintaining cellular integrity and viability include:
- Sample Handling: Rapid processing of samples minimizes degradation.
- Environmental Controls: Use of buffers and protective agents can prevent cellular breakdown.
- Temperature Management: Keeping samples at optimal temperatures during extraction can help preserve DNA quality.
The challenge lies in balancing thorough lysis of the cell to obtain DNA while not compromising the integrity of the genetic material. Techniques that induce minimal stress on the cells during extraction will typically yield higher quality DNA.
DNA Isolation Fundamentals
Once cellular integrity is ensured, the next critical step involves isolating DNA from the cell. The fundamental goal of DNA isolation is to separate nucleic acids from other cellular components such as proteins, lipids, and carbohydrates. Several methodologies exist for DNA isolation, each with its own advantages and limitations.
Important methodologies include:
- Lysis Methods: These can be chemical, where detergents break down cellular membranes, or enzymatic, where specific enzymes degrade cellular components releasing DNA.
- Purification Steps: Following lysis, purification is required to eliminate unwanted materials. This often includes precipitation or chromatography methods that yield high-quality DNA suitable for analysis.
It is essential to optimize each stage of DNA isolation to ensure high yield and purity. Even minor errors in the extraction protocol can degrade the quality of the final product, potentially leading to inaccurate subsequent analysis. A deep understanding of these underlying principles equips researchers to make informed choices in their experimental designs and increases the reliability of their findings.
"The meticulous attention to detail in the extraction process creates a foundation for trustworthy genomics research."
In summary, the essential principles of single cell DNA extraction encompass a blend of technical know-how and careful methodology. Mastering these principles lays a pathway to successful research outcomes in the evolving field of genomics.
Methods for Single Cell DNA Extraction
In the realm of single cell DNA extraction, the choice of methods significantly impacts the efficacy and quality of the isolated DNA. Selecting an appropriate technique is paramount to ensure that the resulting data is reliable and reproducible. The methods not only dictate operational efficiency but also influence downstream applications, from sequencing to genetic analysis, making this a critical aspect of genomic research. This section will delve into various methods, considering their unique characteristics and contributions to the extraction process.
Micromanipulation Techniques
Micromanipulation techniques are precise methods that enable the extraction of DNA from individual cells. These techniques focus on manipulating the cellular environment to isolate DNA without compromising the integrity of the sample.
Laser Capture Microdissection
Laser Capture Microdissection, or LCM, utilizes a focused laser beam to isolate specific cells from a tissue section. This technique provides a significant advantage in selectively targeting the cells of interest while maintaining their histological context. One key characteristic of LCM is its ability to capture cells directly from preserved tissues, which is beneficial for studies needing intact cellular morphology. However, LCM can be resource-intensive and requires rigorous training for operators. The need for specialized equipment may limit accessibility for some researchers.
Optical Tweezers
Optical Tweezers function by using laser light to hold and manipulate microscopic objects, including cells. This method allows researchers to grasp a single cell with great precision, enabling detailed study and extraction. One notable characteristic is the non-invasive nature of this technique, which helps to preserve cellular viability during manipulation. Despite these advantages, Optical Tweezers are often complex and may demand a steep learning curve, potentially limiting their practicality in everyday laboratory settings.
Drop-Driven Techniques
Drop-driven techniques focus on utilizing microdroplets for cell manipulation and DNA extraction. These innovative methods offer distinct advantages in efficiency and scalability, making them attractive for high-throughput applications.
Microfluidics
Microfluidics is a technology that involves the precise control of fluids at the microscale. This technique enhances DNA extraction by enabling the handling of small volumes of fluid, resulting in improved sensitivity and specificity. A key characteristic of microfluidics is its potential for automation. This makes it a favorable choice for researchers looking to streamline their workflow. However, challenges such as channel clogging and the need for specialized designs can pose limitations for broader application.
Droplet Digital PCR
Droplet Digital PCR (ddPCR) is a revolutionary method that partitions a sample into thousands of droplets for individual analysis. This method significantly enhances sensitivity and allows for quantification of DNA with high precision. The unique feature of ddPCR lies in its ability to provide absolute quantification without the need for standard curves. Despite its advantages, the high cost of reagents and the need for specialized equipment may restrict its use in some laboratories.
Lysis and Purification Methods
The lysis and purification methods are fundamental to efficient single cell DNA extraction. These techniques focus on breaking down cellular structures to release DNA while ensuring its purity and integrity.
Chemical Lysis
Chemical Lysis employs detergents or enzymes to disrupt the cell membrane. This approach is simple and effective for various cell types. A significant advantage of chemical lysing is its generally lower cost and ease of use. However, handling hazardous chemicals poses safety concerns, which require careful protocol adherence to prevent accidents.
Enzymatic Lysis
Enzymatic Lysis utilizes specific enzymes such as proteases to break down cellular components. This method is highly selective, making it suitable for sensitive applications. The primary benefit of this technique is its ability to preserve DNA integrity due to mild conditions compared to chemical lysis. However, longitudianl process may become more time-consuming in certain instances.
In summary, understanding the various methods for single cell DNA extraction is essential for researchers looking to enhance their experimental outcomes. Each technique has its own set of advantages and limitations, which must be considered in relation to specific research goals. With advancements in technology, the landscape of single cell DNA extraction continues to evolve, promoting a deeper exploration of genetic material.
Comparative Analysis of Extraction Techniques
In the realm of single cell DNA extraction, a comparative analysis of techniques serves vital purpose. Understanding the nuances between various methods not only aids researchers in selecting the most suitable technique but also enhances the overall efficiency and accuracy of genetic studies. This analysis highlights critical aspects such as efficiency, cost implications, and technical limitations — factors that must be considered to ensure successful outcomes in genomic research.
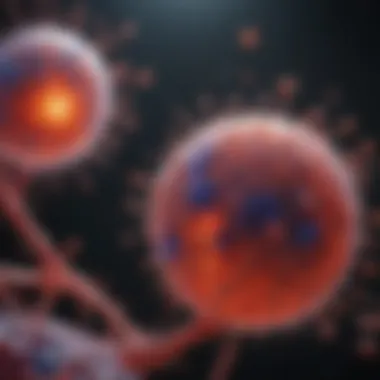
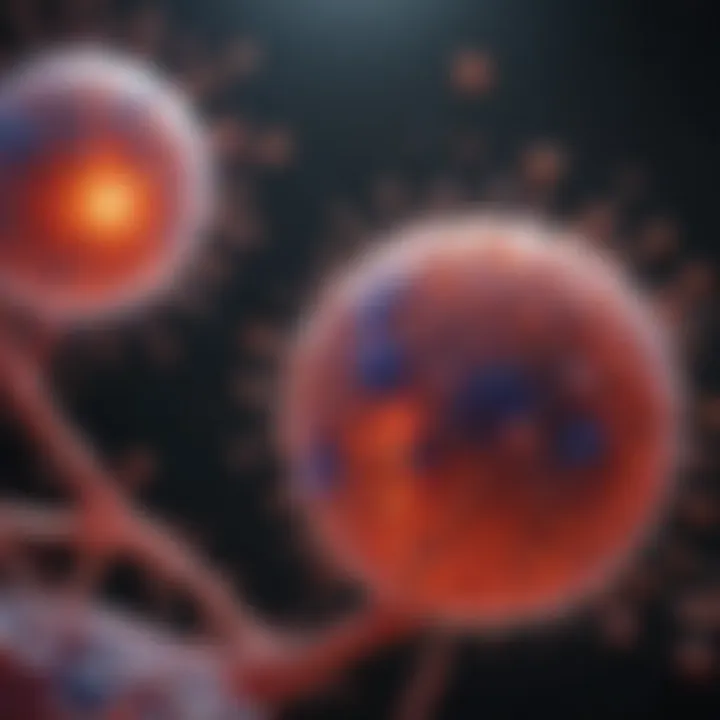
Efficiency of Different Methods
Efficiency in single cell DNA extraction methods can reflect both yield and quality of the extracted DNA. Some techniques, like laser capture microdissection, are highly proficient at isolating specific cells but can be time-consuming. Conversely, methods such as microfluidics may facilitate quicker extraction but can sometimes result in lower DNA quality due to cell stress during the process. Key performance indicators include:
- Yield: Amount of DNA obtained from the process. Higher yields allow for more extensive analysis.
- Quality: Integrity of DNA affects downstream applications like sequencing.
- Time Efficiency: Quick methods promote scalability in experiments.
Ultimately, the choice of method often involves a trade-off between yield and quality, depending on the specific needs of the research.
Cost Implications
Cost analysis plays a significant role in determining which single cell extraction technique a lab may implement. Advanced technologies, such as optical tweezers, can be substantially more expensive due to equipment costs and operational complexities. In contrast, more traditional methods may present lower initial costs but may lack efficiency or precision.
Researchers must consider:
- Initial Setup Costs: Investment in instruments and materials necessary for the extraction process.
- Operational Costs: Ongoing costs for reagents, maintenance, and personnel training.
- Cost per Sample: Important for large scale studies where multiple samples are processed simultaneously.
In many instances, a balance between high-quality results and manageable costs establishes the practical frameworks for laboratory operations.
Technical Limitations
Despite the strides in single cell DNA extraction technology, technical limitations remain a concern. Different methods come with specific constraints that can compromise their application. These might include:
- Contamination Risks: Handling of single cells increases the likelihood of contamination, potentially skewing results.
- Sample Degradation: Prolonged exposure to harsh conditions during extraction can compromise DNA.
- Reproducibility: Variability between samples can affect the reliability of results, key in validating findings.
"Technical drawbacks often dictate the pace at which single cell technologies can advance, necessitating continued innovation and optimization."
Addressing these limitations is essential for improving the robustness of single cell analysis and fostering reproducible results across laboratories.
Understanding these comparative elements in single cell DNA extraction techniques can significantly impact the effectiveness of genomic research. By navigating efficiency, costs, and limitations, researchers can better tailor their approaches to suit specific project needs, ultimately advancing the field of genetics.
Applications in Genomics
The field of genomics is rapidly advancing, largely due to the developments in single cell DNA extraction. This advancement allows researchers to delve deeper into genetic analysis at an individual cell level, providing insights that traditional methods could not achieve. The importance of this topic cannot be overstated, as single cell approaches shed light on the nuances of biological systems.
One primary benefit of single cell DNA extraction is the enhancement of resolution when studying heterogeneity within tissue samples. This is particularly crucial in cancer research, where cell populations can differ significantly in their genetic makeup. By analyzing DNA from individual cells, it becomes possible for researchers to identify subpopulations that may contribute to tumor progression or drug resistance.
Moreover, the ability to perform single cell sequencing allows for comprehensive profiling of cellular states. This profiling aids in understanding cell differentiation and function, revealing the mechanisms underlying various biological processes. Single cell sequencing has expanded the scope of genomics beyond bulk analysis, leading to more accurate representations of cellular diversity.
However, despite its promising applications, several considerations must be kept in mind. The complexity of handling single cells brings technical challenges that impact the quality of results. Additionally, cost implications for single cell methodologies can be significant, requiring careful evaluation of the benefit versus expenditure.
"Single cell DNA extraction revolutionizes our perception of genomic information, enabling us to investigate the unique genetic landscape of individual cells."
As genomics continues to evolve, the integration of single cell DNA extraction techniques becomes essential for pushing the boundaries of scientific discovery. The capacity to analyze the intricate details of cellular genetic content opens new pathways for research and therapeutic innovations.
Single Cell Sequencing
Single cell sequencing represents a cornerstone of modern genomics, allowing for the comprehensive analysis of genetic material from individual cells. This technique enables researchers to capture the diversity of genomic information in a way that bulk sequencing cannot. Each cell's DNA can provide distinctive insights, and the method ensures that even rare cell types are adequately represented in genomic studies.
Methods and Technologies
Numerous methods exist for single cell sequencing, such as 10x Genomics Chromium and SMART-Seq technologies. The key characteristic of these methods is their ability to generate high-quality sequencing data from minute amounts of DNA. 10x Genomics Chromium, for example, employs microfluidic technology to partition cells into droplets, facilitating efficient and scalable sequencing across numerous cells.
A major advantage of these technologies is their high throughput capability, allowing for the analysis of thousands of cells in a single experiment. However, they also come with challenges such as the potential for increased error rates in sequencing outputs. This factor requires careful optimization and validation when interpreting results. Overall, the specificity and sensitivity of existing methods make them a popular choice among researchers pursuing single cell genomic analysis.
Genetic Variation Analysis
Understanding genetic variation is crucial in genomics, and single cell DNA extraction plays an important role in facilitating this analysis. It allows researchers to observe variations among individual cells, providing critical information on genetic diversity within populations. This is particularly significant in studying genetic disorders and complex traits.
Genetic variation can be traced through single nucleotide polymorphisms (SNPs) and copy number variations (CNVs). By employing single cell techniques, investigators can identify these variations with unprecedented precision. This contributes to a broader comprehension of the genetic underpinnings of diseases, opening potential avenues for targeted therapies.
Impact on Understanding Disease
The implications of single cell DNA extraction extend beyond mere analysis; it significantly impacts our understanding of various diseases. With the ability to dissect the genetic profile of individual cells, researchers can unveil the heterogeneous nature of diseases like cancer.
Identifying how genetic variations within individual cells contribute to disease mechanisms allows for more personalized approaches to treatment. Furthermore, studying the evolution of genomic changes in cells over time helps elucidate the pathways that lead to disease progression and resistance.
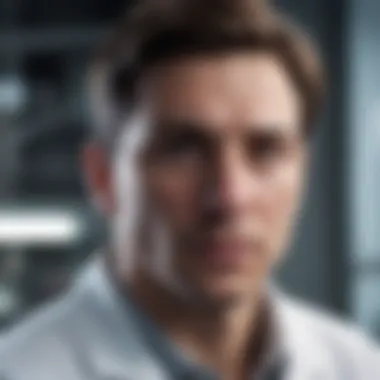
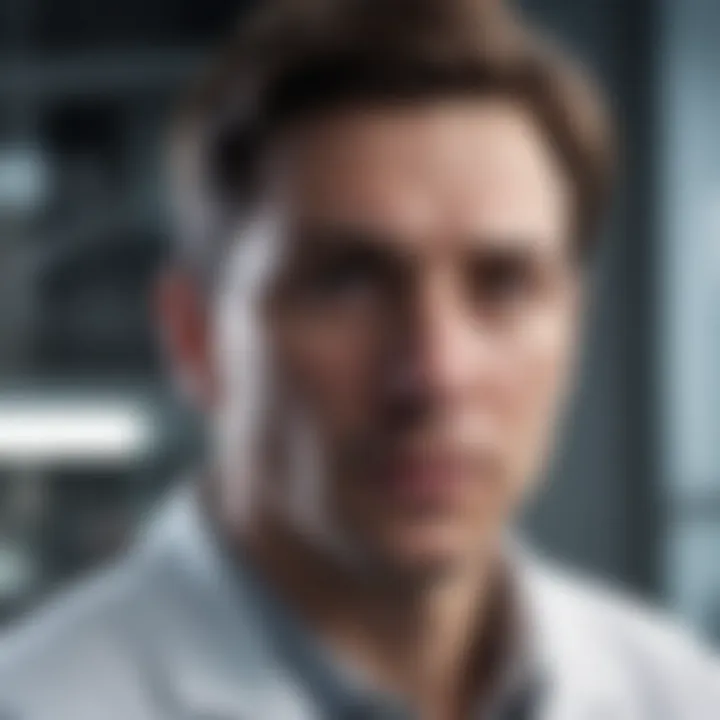
Through single cell analysis, the potential for developing tailored therapies based on the unique genetic landscape of individual patients is a promising frontier in personalized medicine. This approach not only enhances treatment efficacy but also contributes to improved patient outcomes.
Challenges in Single Cell DNA Extraction
The process of single cell DNA extraction is essential, but it is also fraught with challenges that can impact the success and reliability of experiments. Understanding these challenges not only highlights the obstacles researchers face but also points to areas that need innovation and improvement in the field. The importance of this topic rests in its implications for the accuracy of genomic studies and the advancement of personalized medicine. Properly addressing these challenges enhances the overall value of single cell analysis.
Contamination Risk
Contamination is a significant concern in single cell DNA extraction. Even a small amount of extraneous DNA can skew results, leading to misinterpretations of genetic data. The sources of contamination are varied and can include environmental factors, equipment, and even the handling techniques used by researchers. To mitigate this risk, stringent protocols are essential.
- Working in Sterile Conditions: Lab environments should adhere to strict cleanliness standards. This includes using sterile tools and reagents and working in designated clean areas.
- Use of Controls: Incorporating negative controls in experiments can help identify potential contamination. This allows researchers to discern whether observed DNA comes from the target sample or from contaminants.
- Routine Calibration and Maintenance of Equipment: Properly maintained instruments reduce contamination risks, ensuring that samples remain pure throughout the process.
Addressing contamination effectively ensures the integrity of genomic data, which is crucial for reliable analysis.
Yield and Quality of DNA
The yield and quality of DNA extracted from single cells can vary extensively based on the chosen methods and technologies. Low yield hinders downstream applications like sequencing or amplification, while poor quality can result in incomplete or erroneous data.
Several factors influence yield and quality:
- Cell State: The viability and physiological state of the cell at the time of extraction impact DNA quality. Cells that are under stress or dying tend to produce degraded DNA.
- Extraction Techniques: Each method, whether it is micromanipulation or chemical lysis, carries inherent advantages and limitations. For example, while microfluidic techniques offer precision, they may not capture all DNA present.
- Optimization of Protocols: Standardized protocols should be tailored to specific cell types to ensure maximum yield and high-quality DNA.
Investing in methods that enhance yield and quality paves the way for more profound discoveries in genomics and disease research.
Technical Reproducibility
Technical reproducibility refers to the ability to achieve the same results under consistent conditions across different experiments. This is particularly crucial in single cell DNA extraction due to the inherent variability associated with handling and processing individual cells.
Key factors affecting reproducibility include:
- Protocol Consistency: Variability in procedural steps can lead to differing outcomes. Documenting and following a clear protocol is vital for reproducibility.
- Operator Skill: The experience and skill level of the researcher can introduce variability. Training emphasizes the importance of technique to achieve consistent results.
- Environmental Stability: Fluctuating conditions, such as temperature and humidity, can affect DNA extraction processes. Conducting experiments in controlled environments can minimize these risks.
Improving technical reproducibility ensures that findings are reliable, which is critical for both academic research and clinical applications.
"Addressing the challenges in single cell DNA extraction safeguards the reliability of genomic studies and their applications in understanding disease mechanisms."
Future Directions in Single Cell DNA Extraction
Single cell DNA extraction is rapidly evolving. Understanding future directions is crucial for various fields such as genomics, personalized medicine, and molecular biology research. The innovations emerging in this area promise to enhance the efficiency, accuracy, and applicability of single cell analysis. Embracing these advancements can lead to significant breakthroughs in our understanding of genetic diversity and the pathology of diseases.
Technological Innovations
Innovations in technology are key drivers in the field of single cell DNA extraction. Advances in microfluidics, robotics, and automation are reshaping how researchers approach single-cell analyses. For instance, microfluidic devices allow for precise manipulation of fluids at the microscale. This innovation not only improves the yield of extracted DNA but also reduces contamination risk, which is vital for accurate data interpretation.
Additionally, novel techniques like CRISPR-Cas9 and next-generation sequencing are enhancing the capabilities to analyze single cells in unprecedented detail. These methods allow for targeted editing and sequencing, yielding insights into gene function and expression patterns that were previously challenging to achieve. The combination of these technologies makes it possible to gather comprehensive genomic data while maintaining the integrity of the individual cells being studied.
Integration with Other Omics
Integrating single cell DNA extraction with other omics technologies, such as transcriptomics and proteomics, opens new avenues for research. This integrative approach enables a holistic understanding of biological systems. For example, by linking DNA information with RNA expression profiles, researchers can gain deeper insights into gene regulation mechanisms. The combined data sets enhance the power of analysis, allowing scientists to draw more profound conclusions regarding cellular behavior and disease mechanisms.
Moreover, this integration can lead to advancements in biomarker discovery. By understanding how genetic information correlates with expression data and protein profiles, researchers can identify potential therapeutic targets or diagnostic markers more effectively. This approach is not just beneficial in research settings but has potential implications in clinical contexts as well.
Potential in Personalized Medicine
The role of single cell DNA extraction in personalized medicine is growing. Precision medicine targets treatment based on individual genetic profiles. Single cell analyses enable clinicians to tailor therapies by understanding the genetic variability within a patient's tumor or other tissues. This nuanced understanding can lead to more effective treatment plans and better patient outcomes.
Additionally, leveraging single cell extraction techniques, researchers can explore heterogeneity in diseases, especially cancer. The ability to analyze the unique genetic makeup of individual cells within a tumor allows for targeted interventions that consider the distinct characteristics of the tumor’s microenvironment.
"Personalized medicine represents the future of healthcare, emphasizing tailored treatments based on comprehensive genomic resources."
In summary, the future directions in single cell DNA extraction hold immense promise. Technological innovations enhance laboratory practices, while integration with other omics disciplines provides richer datasets for analysis. This direction not only advances basic science research but also substantially impacts clinical practices, paving the way for personalized medicine approaches that could transform patient care.
The End
The conclusion is an essential part of this article, encapsulating the intricate discussions held throughout the various sections. It serves as a moment of reflection on how single cell DNA extraction techniques intertwine with contemporary biological research. The importance of these methodologies cannot be overstated. As they evolve, they continue to impact fields such as genomics and personalized medicine profoundly.
Summary of Findings
The article has highlighted several key aspects about single cell DNA extraction. First, it defined the principles underpinning the techniques and explained their historical context. Notably, the review of various methods such as micromanipulation and lysis techniques showcased the diversity available in this area. Furthermore, the section on comparative analysis brought to light the efficiency, costs, and technical limitations associated with each method.
In terms of applications, single cell sequencing was identified as a groundbreaking advancement. It allows researchers to explore genetic variation with greater precision, thereby enhancing our comprehension of diseases. However, the article also detailed the challenges faced in this field, particularly regarding contamination risks and DNA quality. Simple enhancements in methodology and technology could address these issues.