The Role of Superior Carriers in Science
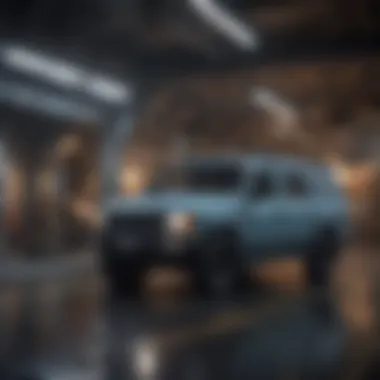
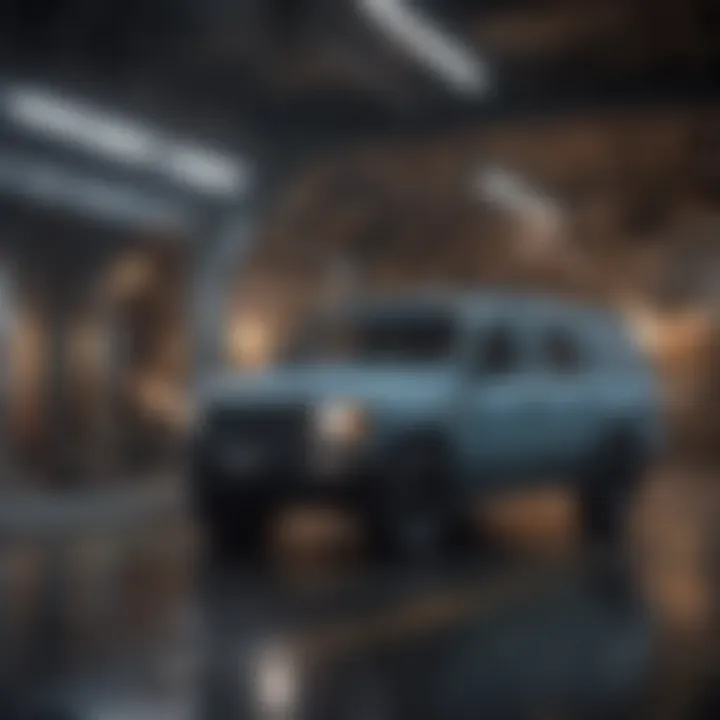
Intro
The concept of superior carriers plays a pivotal role in various scientific landscapes. Understanding these carriers requires a detailed investigation into their structure, function, and the context in which they operate. Superior carriers can be fundamentally identified as entities that enhance efficiency and functionality across biological, chemical, and technological domains. As disciplines evolve and interconnect, the significance of these carriers becomes clear. Biologists may focus on natural carriers such as proteins, while engineers might look towards technological interfaces. This article aims to unravel this complexity, offering insights that elevate the comprehension of this crucial topic.
Research Overview
Summary of Key Findings
In examining the role of superior carriers, several key findings emerge:
- Superior carriers exhibit unique properties that enhance their efficiency.
- They play crucial roles in biological systems, enabling processes like transport and signaling.
- In chemistry, superior carriers might refer to catalysts that optimize reactions.
- Technological designs increasingly incorporate superior carriers, aiding in energy transfer and communication.
The analysis of these findings helps clarify how diverse fields recognize the implications of superior carriers in their respective studies.
Importance of the Research
The importance of exploring superior carriers cannot be understated. They exist at the intersection of many scientific disciplines, driving advances in research and application. Researchers gain deeper insights into the mechanisms of natural systems and innovate new solutions for modern challenges. Understanding superior carriers fosters a holistic approach to science, where biological efficiency informs technology and vice versa.
"A carrier's efficiency can determine the success of a biological or technological system. Recognizing this relationship opens avenues for innovation."
Methodology
Study Design
The approach to studying superior carriers involves a multidisciplinary perspective. This design incorporates methods from biology, chemistry, and engineering to provide a comprehensive view of their roles. By utilizing both qualitative and quantitative research techniques, the study aims to establish a well-rounded understanding of the carriers.
Data Collection Techniques
Data is collected through various techniques. Common methods include:
- Experimental setups in laboratories to observe carrier interactions.
- Computational models to simulate carrier behavior in different environments.
- Literature reviews to contextualize findings within existing research.
This combination ensures the research is grounded in empirical evidence and theoretical frameworks, contributing to the ongoing dialogue in scientific communities.
Foreword to Superior Carriers
The realm of scientific inquiry has brought us to understand the crucial concept of superior carriers. These carriers are fundamental in various disciplines, acting as vehicles for transporting essential substances or energy. Their role is markedly significant across biological, chemical, and physical domains. The exploration of superior carriers enhances our understanding, enabling advancements that might be pivotal in research and technology.
Superior carriers can be seen as facilitators in both natural processes and industrial applications. They operate on several levels, from microscopic molecular movements to larger scale engineering innovations. Recognizing their importance helps to unlock efficiency gains and improve functionality in diverse systems.
Definition and Context
A superior carrier can be defined as a medium or entity that effectively transports components, be it ions, molecules, or energy. In many scenarios, such as in biological systems, these carriers exhibit necessary properties that enhance speed and selectivity during transport processes. For instance, in human cells, certain proteins function as carriers for ions, allowing for proper physiological responses.
In the context of technology, superior carriers hold the potential to improve material efficiency and reduce energy loss in systems. This is not just limited to biological structures; the integration of advanced carriers in material science can revolutionize how we store or transfer energy. Thus, the understanding of their defining features is crucial in all scientific fields.
Historical Perspectives
The historical development of carrier systems provides insights into their evolution and significance. Initial observations of transport mechanisms appeared in studies of cellular biology, where scientists noted how substances move across membranes. Key figures like Jan Evangelista Purkinje expanded our knowledge about cellular transport in the 19th century.
As scientific inquiry progressed, the development of superior carriers shifted from merely observational to experimental approaches. The discovery of ion channels and transport proteins in the mid-20th century marked a significant turning point. These findings laid the groundwork for modern research, influencing subsequent studies in areas such as pharmacology and bioengineering.
In recent years, a multidisciplinary approach emerged. This integration allowed for innovative discoveries still based on these original principles. Modern exploration further enriches the historical narrative surrounding superior carriers, marrying ancient knowledge with cutting-edge technology.
By reflecting on the journey of superior carriers through time, we can better appreciate their impact and potential in future research and applications, emphasizing their critical role in advancing our scientific understanding.
Mechanistic Insights into Superior Carriers
The study of superior carriers is integral to understanding their functionality across various scientific disciplines. These carriers serve as conduits for the transfer of materials, energy, and information, making their mechanistic insights crucial in diverse applications. Focusing on the specific attributes of these carriers allows researchers and practitioners to enhance the effectiveness of processes in fields such as biology, chemistry, and engineering.
Types of Carriers
Molecular Carriers
Molecular carriers are distinct in their ability to transport substances at the molecular level. They are known for their precision and capability to facilitate targeted delivery. This makes them beneficial in areas such as drug delivery, where they help ensure that therapeutic agents reach their intended sites of action. One key characteristic of molecular carriers is their design, which often includes functional groups that enable binding to specific target molecules.
The unique feature of molecular carriers is their versatility; they can be engineered to carry various types of molecules, from small drugs to larger biomolecules. However, one disadvantage is their complexity in synthesis and potential issues with stability in biological environments.
Ionic Carriers
Ionic carriers play a significant role in the movement of ions across membranes, essential in many chemical and biological processes. The key characteristic of ionic carriers is their ability to facilitate ion transport, which is vital for processes such as signaling in neurons and energy transfer in batteries. Their mechanism often involves the selective transport of ions based on size and charge, which improves efficiency.
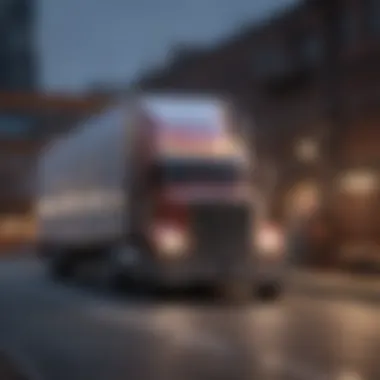
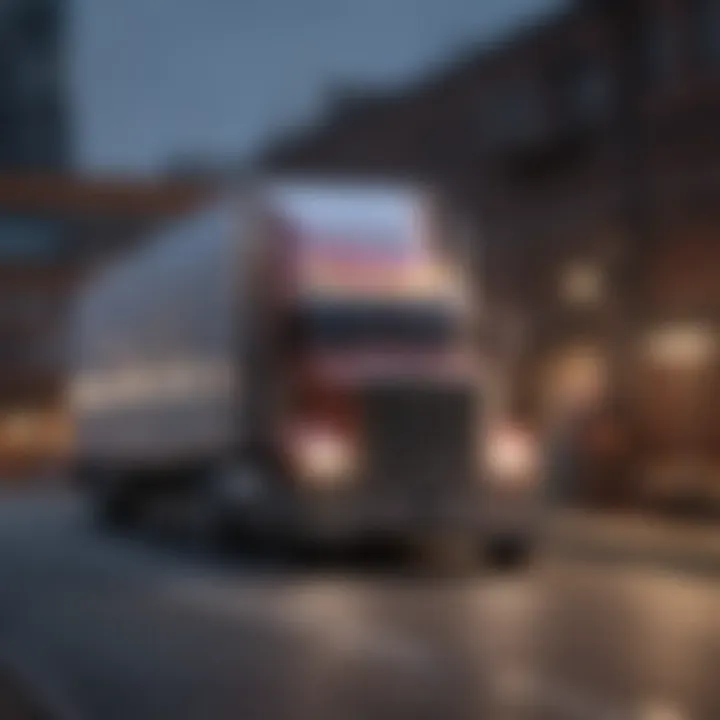
A notable feature is their rapid transport rates compared to other types of carriers. Nevertheless, ionic carriers can be limited by binding interactions with other ions, affecting their performance in certain systems.
Biological Carriers
Biological carriers, such as proteins and vesicles, are crucial in living organisms. They take part in essential functions like nutrient transport and waste removal. Their key characteristic lies in their ability to interact with other biological molecules effectively, ensuring proper cellular function.
One unique attribute of biological carriers is their dynamic nature, allowing them to adapt to varying cellular conditions. Their advantages include specificity and efficiency in cellular environments. However, they may also face challenges such as denaturation or loss of function in non-physiological conditions.
Structural Characteristics
The structural characteristics of superior carriers directly impact their performance. Factors such as size, shape, and surface properties play significant roles in determining how these carriers interact with their environments. For instance, molecular carriers often feature specific configurations that allow for optimal binding to target molecules. In contrast, ionic carriers are defined by their ion selectivity, which can be enhanced by their structural design. Understanding these structural features is critical for advancing research and application in various scientific fields.
"The design and structure of carriers greatly influence their functional efficiency, which is essential for innovation in technology and science."
By examining both the types of carriers and their structural characteristics, one can appreciate their contributions to improving processes across many fields. The understanding of these mechanistic insights paves the way for further exploration and development of superior carriers.
Superior Carriers in Biological Systems
The concept of superior carriers is crucial in biological systems. These carriers enhance processes integral to life. They enable the transport of substances, facilitating key cellular functions. Understanding these carriers can lead to better insights in biological research, potentially improving health outcomes and biotechnological applications.
Role in Cellular Processes
Transport Mechanisms
Transport mechanisms stand as a critical function of superior carriers. They facilitate the movement of ions, molecules, and other necessary compounds across cell membranes. This transport is vital for maintaining homeostasis and allowing cells to adapt to changing environments.
A key characteristic of transport mechanisms is their specificity. Proteins involved often exhibit selectivity for particular substrates. This specificity ensures that essential nutrients are absorbed while waste products are expelled. Consequently, these carriers are an effective choice for study in this article, providing insights into their efficiency and adaptability in various environments.
A unique feature of transport mechanisms is their adaptability. Many transport systems can adjust their activity based on cellular conditions, optimizing performance under different physiological states. This ability proves advantageous in dynamic biological systems; however, it can also present challenges in the context of disease where transport may be disrupted.
Signal Transduction
Signal transduction offers another dimension to the role of superior carriers. This process is essential for cells to communicate and coordinate actions. It involves receptors that transmit signals from the environment through biologic systems prompting responses.
A key characteristic of signal transduction is its integration with transport mechanisms. These systems often work in tandem to relay information and substances where they are needed. This integration is a key reason for highlighting signal transduction in this article, reflecting on how it underpins the effectiveness of cellular responses.
A unique feature of signal transduction is the amplification of signals. A single external stimulus can lead to a cascade of cellular events. This can be both beneficial for cellular responses, as it enhances sensitivity, and disadvantageous if signals become dysregulated, potentially leading to pathologies.
Influence on Metabolism
The influence of superior carriers on metabolism is profound. They play a pivotal role in mediating biochemical reactions and energy exchanges. Carriers are involved in shuttling metabolites and facilitating reactions necessary for energy production and utilization in cells.
This relationship between carriers and metabolism underscores the importance of research in this area. Disruptions in carrier function often lead to metabolic disorders, making it a significant focus area for scientists and clinicians alike. Understanding how carriers operate can lead to breakthroughs in treatment strategies and metabolic engineering.
Applications in Chemistry
The intersection of superior carriers and chemistry represents a dynamic area of research. In this section, we will discuss how these carriers enhance various chemical processes, thus underscoring their significance in both theoretical and practical applications. Superior carriers play a central role in catalysis and material science, driving reactions efficiently and enabling the development of advanced materials. Understanding these applications is crucial for students, researchers, and professionals interested in improving chemical processes and exploring new innovative solutions.
Catalysis and Reaction Mechanisms
Catalysis is a fundamental process in chemistry that involves the acceleration of chemical reactions via the introduction of a catalyst. Superior carriers enhance this process by providing pathways for reactants to interact more effectively, lowering the activation energy required for reactions to proceed.
The use of molecular carriers in catalytic systems has been explored extensively. These carriers can stabilize transition states and provide specific environments where reactions can occur preferentially. For instance, platinum nanoparticles on carbon supports can offer high surface areas, enabling more active sites for catalytic reactions. This results in increased reaction rates and yields, which are essential for industrial applications.
Moreover, the design of ionic carriers can facilitate ion transfer during electrochemical reactions. The efficiency of batteries and fuel cells significantly benefits from these carriers, which ensure swift ionic movement and enhance overall energy conversion.
Key benefits:
- Increased reaction efficiency
- Lower energy requirements for reactions
- Enhanced selectivity towards desired products
Role in Material Science
In material science, the application of superior carriers is equally profound. They are involved in creating and optimizing materials with specific properties for various applications. This includes enhancing the mechanical, thermal, and electrical properties of materials. Superior carriers can incorporate additives that modify the material's features.
For example, the use of biological carriers in developing biodegradable plastics shows promise. These materials, imbued with natural fibers or proteins, support environmental sustainability while maintaining performance. Similarly, the introduction of nanomaterials as carriers can significantly improve the performance of traditional materials.
"Superior carriers transform material performance and enable innovative applications in everyday products."
Applications in material science include:
- Development of advanced composites
- Creation of smart materials that respond to external stimuli
- Enhancement of drug delivery systems using biocompatible materials
Impacts of Superior Carriers in Physical Sciences
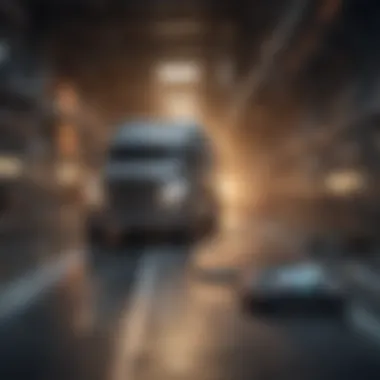
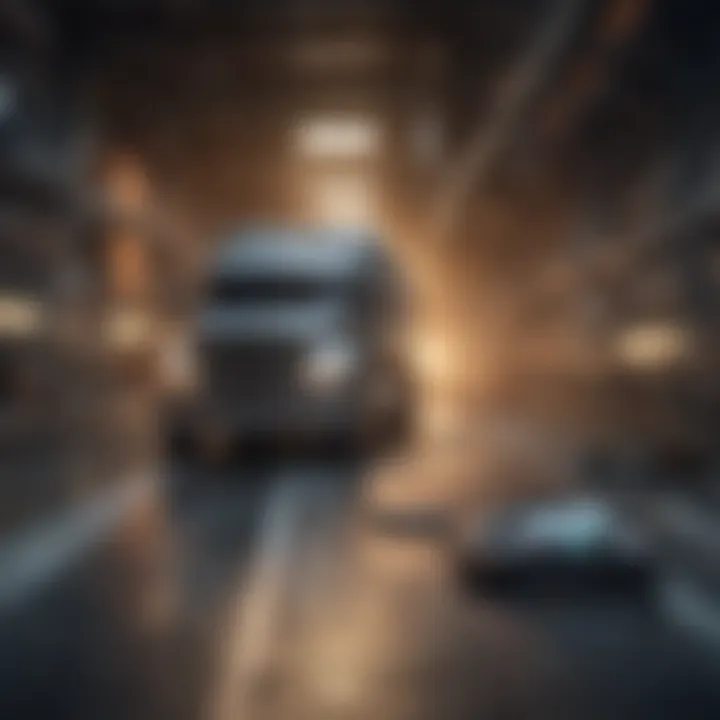
The exploration of superior carriers in physical sciences reveals their central role in enhancing functionality across various applications. These carriers have significant implications for energy efficiency, storage solutions, and advancements in nanotechnology. Their contributions extend beyond theoretical frameworks, influencing practical implementations and innovations in scientific research and industry.
Energy Transfer and Storage
Energy transfer and storage are critical aspects within physical sciences. Superior carriers optimize these processes, resulting in significant improvements in efficiency. For example, materials like graphene and carbon nanotubes are pivotal in energy storage strategies. They facilitate rapid charge transfer, which is essential for high-performance batteries.
The mechanisms employed by these carriers enable improved energy retention, which benefits various applications, from portable electronics to renewable energy systems. Moreover, the selection of the appropriate superior carrier can also have profound impacts on the scalability and sustainability of energy systems. The right choice can lead to reduced losses during energy transfer, making systems more effective.
"Advanced materials can reduce inefficiencies in energy systems, paving the path for future innovations."
Beyond energy storage, superior carriers play a role in smart grid technologies. These systems rely on efficient energy distribution, where superior carriers can enhance the responsiveness and reliability of energy flow. This is particularly crucial in renewable energy integration, where fluctuations in generation must be managed effectively.
Applications in Nanotechnology
Nanotechnology benefits greatly from superior carriers, offering innovative solutions to complex challenges. The incorporation of nanomaterials allows for the development of advanced carrier systems that exhibit unique properties at the nanoscale. These carriers enhance performance in various applications, including drug delivery, environmental remediation, and sensor technologies.
In drug delivery, nanocarriers, such as liposomes and dendrimers, are engineered to transport therapeutic agents in a highly targeted manner. This precision helps in reducing side effects while increasing treatment efficacy. It opens new avenues in personalized medicine, where treatment can be tailored based on the carrier characteristics.
Furthermore, superior carriers can improve the performance of sensors used for environmental monitoring. Their ability to interact with specific molecules enhances detection limits and sensitivity, thus making them invaluable in identifying pollutants or hazardous substances.
Overall, understanding and utilizing superior carriers in nanotechnology fosters advancements that intersect multiple scientific disciplines, inching closer to solving real-world problems in an efficient manner.
Technological Innovations Utilizing Superior Carriers
The advent of superior carriers holds significant potential in various technological fields, particularly in enhancing the efficiency of systems and processes. These innovations are pivotal as they facilitate advancements in communication technologies and improve transportation systems. Understanding how superior carriers function in these contexts can illuminate their role in achieving greater effectiveness and reliability.
Advancements in Communication Technologies
Superior carriers are integral to modern communication infrastructure. Their roles range from facilitating high-speed data transfer to ensuring the integrity of communication signals. For instance, fiber optic cables, which serve as superior carriers of information, allow for data transmission at the speed of light and with minimal signal degradation. This not only enhances internet connectivity but also supports the vast amount of data shared across global networks.
Some specific benefits of using superior carriers in communication include:
- Increased Bandwidth: Superior carriers can support a higher capacity for data, making it possible to transmit large amounts of information simultaneously.
- Reduced Latency: Advanced carriers enhance the speed of communication, resulting in quicker transmission times.
- Improved Signal Quality: With advanced materials and technologies, superior carriers minimize noise and distortion in transmission.
"The evolution of communication technologies driven by superior carriers paves the way for more robust and efficient systems, essential for our increasingly digital world."
These advancements make data and voice communications more efficient, which is especially critical in an era where remote work and global interactions are commonplace.
Improving Transportation Systems
The application of superior carriers extends to the transportation sector as well, where they contribute to the effectiveness of various systems. From freight transport to public transit, superior carriers enhance vehicle efficiency, safety, and reliability.
In the context of transportation, superior carriers can be seen in:
- Smart Logistics: The integration of IoT devices and advanced tracking carriers allows for real-time monitoring of goods in transit. This results in optimized routes and reduced delivery times.
- Sustainable Mobility: Many modern vehicles utilize advanced materials as carriers, which reduces weight and improves fuel efficiency. Electric vehicles, for instance, leverage superior battery technologies that allow for higher performance over longer distances.
- Safety Enhancements: Advanced carrier technologies enable enhanced communication between vehicles and infrastructure, leading to improvements in traffic management and accident prevention.
Such innovations underline how superior carriers are not just a theoretical concept but practical tools that significantly enhance technological systems. Understanding their functionality and implications remains critical for the continued development of efficient and sustainable technological solutions.
The Role of Nanotechnology in Carrier Systems
Nanotechnology has revolutionized the way we understand and implement carrier systems across various scientific domains. Its applications range from enhancing the efficiency of drug delivery systems to improving material properties. The integration of nanotechnology into carrier systems offers distinct advantages that can significantly impact research and application.
One of the most prominent benefits of nanotechnology in carrier systems is the ability to manipulate materials at the atomic or molecular level. This manipulation allows for the design of nanocarriers, which can achieve higher precision in targeting specific tissues or cells. By encapsulating therapeutic agents within nanoparticles, researchers can create tailored solutions that improve the efficacy of treatments.
Nanocarriers in Drug Delivery
Nanocarriers are a specialized application of nanotechnology that focuses on the delivery of drugs within biological systems. These carriers can take various forms, such as liposomes, dendrimers, or solid lipid nanoparticles. Each has unique properties that enable them to transport essential drugs more effectively than traditional methods.
- Targeted Delivery: Nanocarriers can be engineered to target specific cells or tissues, which minimizes side effects while maximizing therapeutic benefits. This is particularly crucial in cancer treatment, where delivering drugs directly to tumor cells improves outcomes significantly.
- Controlled Release: Unlike conventional drug formulations, nanocarriers can provide controlled release profiles. This capability allows drugs to be released over a set period, ensuring sustained therapeutic action while reducing dosing frequency.
- Enhanced Solubility: Many drugs suffer from poor solubility, which can hinder their bioavailability. Nanocarriers can encapsulate these poorly soluble drugs, making them more effective by enhancing their transport through biological membranes.
Despite these advantages, there are several considerations to keep in mind when employing nanocarriers in drug delivery systems. The safety and biocompatibility of nanomaterials remain subjects for ongoing research, as potential toxicity and long-term effects on human health are still not fully understood.
Implications for Research
The integration of nanotechnology in carrier systems opens up numerous avenues for research. This impact can be seen across multiple disciplines, from pharmacology to environmental science.
- Innovative Approaches: Research has shifted towards harnessing nanoscale materials, driving innovation in drug formulations and delivery methods. The exploration of new materials can lead to breakthroughs in treatment methodologies.
- Interdisciplinary Collaboration: Nanotechnology encourages collaboration between fields such as chemistry, biology, and engineering. This interdisciplinary approach can foster holistic solutions to complex problems, thus enhancing scientific inquiry and application.
- Regulatory Considerations: With the advancement of nanotechnology, new regulatory frameworks will need to be developed to evaluate the safety and efficacy of nanocarriers. Understanding how to navigate these regulations is crucial for advancing applications in real-world settings.
"Nanotechnology holds the potential to redefine the landscape of carrier systems within various scientific frameworks, offering pathways to enhanced efficiency and targeted functionality."
Evaluation of Efficiency of Superior Carriers
Understanding the efficiency of superior carriers is vital for their application in various scientific fields. This efficiency governs their performance and usability, influencing both theoretical research and practical implementations. Evaluating this aspect allows scientists and engineers to select the most appropriate carriers, optimize processes, and innovate solutions across biological, chemical, and physical systems.
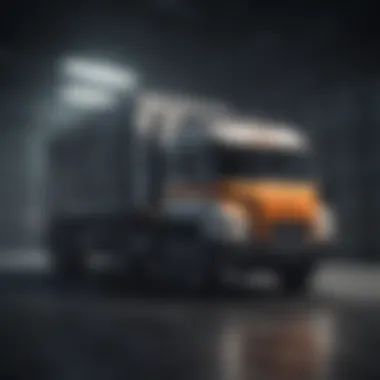
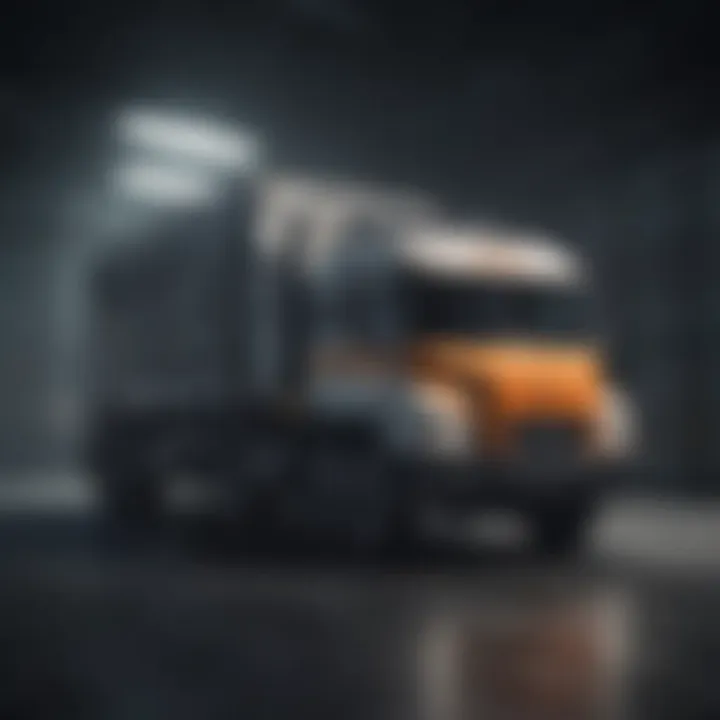
Several specific elements play a crucial role in this evaluation. These elements include measuring transport rates, assessing selectivity and specificity, and comparing traditional systems. By focusing on these parameters, researchers can gain insights into the advantages and disadvantages of different carrier types within their niche disciplines.
The benefits of thoroughly evaluating superior carriers include enhanced transport mechanisms, improved efficiency in reactions or biological processes, and better overall functionality. These factors serve to not only boost efficacy but also to advance the understanding of fundamental scientific principles, paving the way for future innovations.
Measuring Performance Metrics
The performance of superior carriers hinges on several key metrics, primarily the rate of transport and selectivity. Both aspects are integral to not only measuring efficiency but also ensuring that the carriers meet the specific needs of their respective applications.
Rate of Transport
The rate of transport refers to how quickly a carrier can move substances from one location to another. This aspect significantly impacts the overall speed and efficiency of processes. A high rate of transport can lead to faster reactions and improved outcomes in applications such as drug delivery, material science, and chemical reactions.
The key characteristic of the rate of transport is its direct correlation with carrier effectiveness. For instance, in biological systems, a faster transport rate can enhance cellular responses, contributing to overall health and functionality.
However, while a high transport rate is generally favorable, it must be balanced with other factors such as stability and safety. If a carrier acts too quickly, it could lead to unexpected results, which may be disadvantageous in sensitive environments.
Selectivity and Specificity
Selectivity and specificity denote the ability of a carrier to transport particular materials while avoiding undesired substances. This characteristic is crucial in applications ranging from pharmaceuticals to environmental science. Ensuring that a carrier delivers the correct agents while minimizing interactions with other compounds is a prized feature.
The unique feature of selectivity and specificity is its role in optimizing both the efficacy and safety of processes. For instance, in drug delivery, a selective carrier can deliver medication precisely to target cells, providing direct therapeutic benefits while reducing side effects.
Despite its advantages, maintaining high selectivity and specificity can be challenging. Some carriers may require complex engineering or specific conditions to ensure that they perform correctly. This necessity can introduce additional costs and time in the development and application process.
Benchmarking Against Traditional Systems
When evaluating superior carriers, it is essential to benchmark them against traditional systems. This comparison helps identify the strengths and limitations of modern approaches. Traditional systems often embody established techniques, and bringing new carriers into the conversation necessitates clear metrics for evaluation.
In comparison, superior carriers might show improvements in transport rates and selectivity. Using advanced materials and nanotechnology can enhance performance significantly.
Both qualitative and quantitative measures can be used in this benchmarking process. Examples may include:
- Speed of transport
- Specificity of interaction
- Cost-effectiveness
- Safety profiles
Through this evaluation, researchers can determine the effectiveness of superior carriers, guiding future studies and applications.
Future Prospects and Research Directions
The exploration of superior carriers in scientific frameworks presents significant future prospects that warrant careful consideration. As researchers strive to understand and harness these carriers for various applications, the focus on developing efficient systems continues to grow. Innovations in technology and interdisciplinary approaches are likely to enhance the understanding of these carriers.
Emerging Trends in Carrier Research
In recent years, there have been emerging trends in the research of superior carriers. These trends illustrate a shift towards more sustainable and efficient practices. Several areas have gained attention:
- Bio-inspired design: Researchers are looking to nature for inspiration. Biomimicry is being utilized to design carriers that mimic biological processes.
- Smart carriers: Innovations in nanotechnology have led to the development of smart carriers capable of responding to environmental stimuli. These carriers can deliver drugs with increased precision.
- Sustainable materials: Increased focus is on using environmentally friendly materials in the construction of carriers. This change addresses ecological concerns and aligns with global sustainability goals.
As these trends continue to develop, they open new avenues for the effective application of superior carriers in real-world scenarios.
Interdisciplinary Approaches
The future of research on superior carriers is likely to be characterized by interdisciplinary collaboration. Merging knowledge from various fields can lead to significant breakthroughs. Here are a few considerations regarding interdisciplinary approaches:
- Collaboration between biology and engineering: Biological insights can enhance the design of carriers in engineering applications, such as drug delivery systems.
- Integration of chemistry and materials science: Understanding the chemical properties of new materials can lead to innovations in carrier capabilities.
- Engagement with computer science: Algorithms and modeling can help predict the behaviors of carriers in different environments, leading to better design strategies.
"Interdisciplinary research not only fosters innovation but also addresses complex problems from various angles, providing more comprehensive solutions."
Through these combined efforts, the potential applications and efficiencies of superior carriers can be significantly enhanced, paving the way for future advancements in various scientific domains.
Epilogue
The exploration of superior carriers within scientific frameworks represents a crucial convergence of various disciplines, leading to significant advancements in both theoretical understanding and practical applications. This article emphasizes the vital role superior carriers play in enhancing systematic efficiency, underscoring their importance across biological, chemical, and technological domains.
Summary of Key Findings
The research presented in this article highlights several key points:
- Multifunctionality: Superior carriers are not merely transport mechanisms; they facilitate complex functions such as signal transduction, energy transfer, and catalysis.
- Interdisciplinary Relevance: The understanding of these carriers spans across biology, nanotechnology, and material science, indicating their broad significance.
- Innovative Applications: Their implementation in drug delivery systems and communication technologies demonstrates transformative potential, pushing the boundaries of current practices.
- Efficiency Metrics: Measurements of performance, including transport rate and selectivity, are critical for evaluating the effectiveness of superior carriers compared to traditional systems.
Lasting Implications of Superior Carriers
The implications of superior carriers extend beyond immediate scientific advancements. The following considerations illustrate their enduring impact:
- Research Directions: Investigating superior carriers encourages continued interdisciplinary collaboration, fostering advancements in nanoscience and biomedical engineering.
- Broader Scientific Inquiry: Understanding these carriers aids in addressing complex biological systems and developing solutions to contemporary challenges in health and energy sectors.
- Technological Progress: Enhanced materials and systems enabled by superior carriers have the potential to revolutionize existing technologies in communication and transportation.
Overall, the role of superior carriers is foundational in pushing the envelope of scientific inquiries and technological innovations.
"The continued study of superior carriers may unlock new paradigms in science and technology, ultimately leading to enhanced quality of life."
With ongoing research and exploration, the significance of superior carriers will undoubtedly deepen, urging researchers, students, and professionals to invest in understanding their complexity and potential.