An In-Depth Analysis of Trypsin: Function and Applications
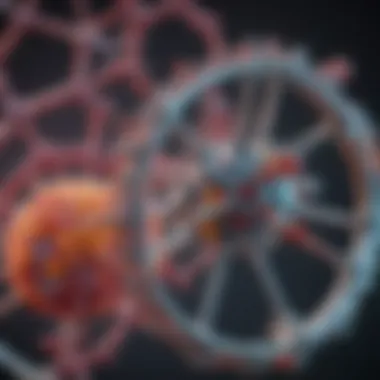
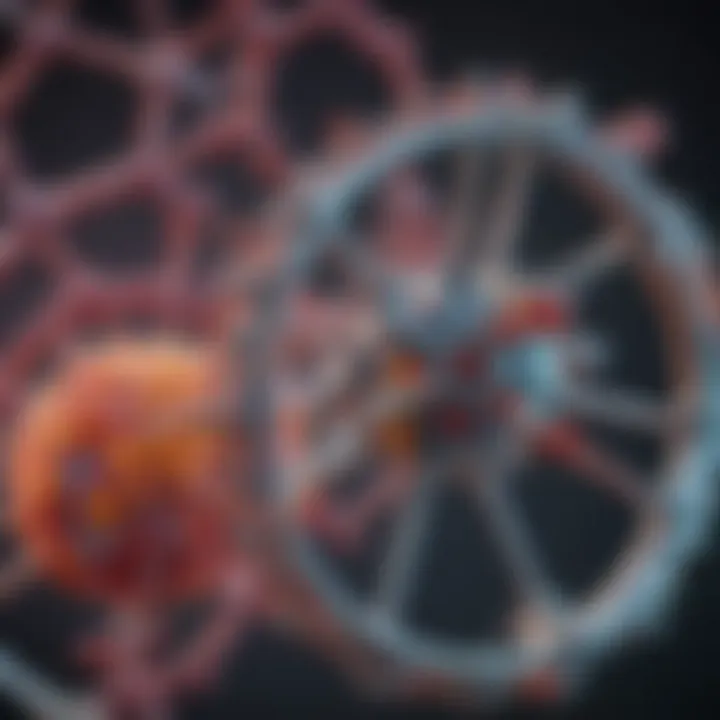
Intro
Trypsin is an essential serine protease that plays a critical role in various biological processes. It is primarily known for its function in digesting proteins, breaking them down into smaller peptides in the small intestine. This article aims to explore trypsin's structure, enzymatic properties, and significance in both biological and biotechnological contexts. The complex mechanisms of trypsin regulation are of keen interest for researchers because they can reveal insights into protein metabolism and the implications of disorders linked to trypsin dysfunction.
Research Overview
Understanding trypsin can lead to breakthroughs in multiple scientific domains, from digestion to therapeutic applications. This section discusses key findings and highlights the importance of research related to this enzyme.
Summary of Key Findings
- Enzymatic Properties: Trypsin exhibits specificity for basic amino acids, cleaving after lysine and arginine residues.
- Role in Digestion: It is a critical component of the digestive system, contributing to protein absorption.
- Biotechnological Applications: Trypsin is frequently used in laboratory protocols, such as cell dissociation and protein digestion for mass spectrometry.
Importance of the Research
The function of trypsin extends beyond digestion; it serves as a model for studying protein interactions and enzymatic activities. Enhancing our understanding of trypsin can provide valuable insights into proteolytic enzymes in general, which has far-reaching implications for health and disease management.
Methodology
This article incorporates various research methodologies to provide a robust understanding of trypsin's roles and mechanisms.
Study Design
The design focuses on both experimental and analytical approaches to investigate trypsin's biochemical functions and applications. Relevant studies range from in vitro experiments that highlight enzymatic kinetics to computational models that offer insight into its structure.
Data Collection Techniques
Data is gathered from a range of peer-reviewed sources, experimental studies, and established scientific databases. Techniques such as enzyme assays, kinetic analysis, and molecular modeling are employed to analyze trypsin’s functionality comprehensively.
"Gaining deeper knowledge of trypsin not only enriches biochemical understanding but may also open avenues for innovative therapeutic strategies."
Understanding Trypsin
Understanding trypsin is crucial for comprehending various biological processes, especially in the context of digestion and protein metabolism. This article section aims to provide insight into trypsin's definition and its historical journey, illustrating how this enzyme has become a pivotal component in both normal physiology and applied sciences. The importance of trypsin lies not only in its catalytic function but also in its regulatory mechanisms and clinical implications.
Definition of Trypsin
Trypsin is a serine protease enzyme produced in the pancreas. Its primary function is to catalyze the hydrolysis of peptide bonds, specifically targeting the amino acids lysine and arginine, which are found in many proteins. Trypsinogen is the zymogen form of trypsin, synthesized in the pancreas and secreted into the small intestine. Once in the intestine, it gets activated by another protease, generally enterokinase, converting it into its active form, trypsin. This activation process is essential as it initiates a digestive cascade that facilitates the breakdown of dietary proteins into absorbable amino acids.
"Trypsin plays a fundamental role in protein digestion, influencing metabolic processes and nutrient absorption."
Historical Background
The history of trypsin can be traced back to the late 19th century. Notably, in 1876, Austrian physiologist Wilhelm Kühne coined the term "trypsin" when he studied pancreatic extracts' enzymatic properties. Following Kühne's work, researchers began to realize the importance of trypsin in digestion.
In the 20th century, advancements in biochemistry and enzyme kinetics led to a deeper understanding of trypsin's structure and catalytic mechanism. The work of scientists like James B. Sumner and John H. Northrop, who ultimately received the Nobel Prize for their studies on enzymes, propelled trypsin research into new territories. Their findings laid the groundwork for recognizing trypsin not just as a digestive enzyme but also as a tool in various biotech applications, which have expanded significantly in contemporary science.
Molecular Structure
The molecular structure of trypsin is fundamental to understanding its function and applications. It encapsulates the specific arrangement of atoms and the three-dimensional conformation that dictate trypsin's activity as a proteolytic enzyme. Analyzing this structure provides insights into how trypsin interacts with substrates and regulators, facilitating its role in various biological processes. The information gleaned from the examination of trypsin's structure can inform everything from its biochemical behavior to its machinist's applications in biotechnology and medical diagnostics.
Amino Acid Composition
The amino acid composition of trypsin is critical when considering its structural and functional dynamics. Trypsin is primarily composed of 223 amino acids. Its sequence includes a significant presence of serine, aspatic acid, and histidine, which are essential for its catalytic activity. The specific arrangement of these amino acids allows for the formation of a functional active site that is instrumental in enzymatic hydrolysis of peptide bonds.
Characteristics of amino acid composition include:
- Hydrophobic and polar amino acids: These create various interactions within the enzyme, stabilizing its structure.
- Essential residues: The presence of serine 195 is crucial, as it participates directly in the cleavage of peptide bonds.
- Zymogen form: Trypsin exists as trypsinogen in the pancreas, which is an inactive form that undergoes proteolytic activation to function in digestion.
Understanding the amino acid composition informs the design of experiments in proteomics and drug development, assisting in elucidating the functionality of trypsin across various applications.
Enzymatic Active Site
The enzymatic active site of trypsin plays a pivotal role in its function. Located within the structure, the active site includes essential residues that facilitate substrate binding and catalytic activity. It exhibits a specificity for basic amino acids like lysine and arginine. The configuration of the active site is specifically developed to recognize the p1 position of the substrate, showcasing the enzyme's substrate specificity.
Key features of the enzymatic active site include:
- Structural pocket: This pocket allows trypsin to accommodate substrates with appropriate charge and size.
- Catalytic triad: The interaction between serine, histidine, and aspartate residues optimally positions them for peptide bond cleavage, making it an efficient catalyst.
- Stability: Conformational stability of the active site ensures that trypsin remains functional across different physiological conditions.
The precise structure and function of the active site are vital for unraveling the mechanisms of trypsin in biological systems and for innovative applications, such as targeted drug design in therapeutic contexts.
Biological Role
The biological role of trypsin is vital to understanding its functions within various physiological processes. Trypsin is a serine protease that plays a critical role in the digestive system. It is primarily responsible for breaking down proteins into smaller peptides, which can then be further digested into amino acids. These amino acids are essential for numerous biological processes, including tissue repair, hormone production, and enzyme synthesis. The efficiency of trypsin in protein digestion directly impacts nutritional absorption and overall metabolic health.
Digestive Function
Trypsin is synthesized in the pancreas as an inactive precursor known as trypsinogen. Once it is secreted into the small intestine, trypsinogen is activated by the enzyme enterokinase. The active form of trypsin then hydrolyzes peptide bonds, particularly after lysine and arginine residues, to facilitate the breakdown of dietary proteins. This process significantly increases the surface area available for subsequent enzymes to act, enhancing the efficacy of digestion.
This enzymatic action not only promotes the breakdown of food but also prepares the carbohydrates and fats for further digestion by other enzymes. As a result, trypsin contributes to a well-coordinated digestive cascade that is crucial for maintaining nutritional homeostasis.
Additionally, adequate trypsin activity is essential for preventing malnutrition and associated disorders. Malfunction or deficiencies in trypsin production or activity can lead to conditions such as protein deficiency, exacerbating issues like growth impairment and immune dysfunction. Therefore, understanding the digestive function of trypsin provides valuable insights into gastrointestinal health and disorders.
Protein Homeostasis
Apart from its digestive roles, trypsin has a significant impact on protein homeostasis within the body. Protein homeostasis, or proteostasis, refers to the regulation of cell protein levels and their proper folding, maintenance, and degradation. Trypsin is involved in these processes by regulating the turnover of various cellular proteins.
Through its proteolytic activity, trypsin can remove improperly folded or damaged proteins, which is critical for maintaining cellular function. Misfolded proteins can accumulate and result in cellular stress, potentially leading to diseases such as Alzheimer’s or Parkinson’s. By ensuring the efficient degradation and recycling of proteins, trypsin aids in cellular health and longevity.
In summary, the biological role of trypsin encompasses both digestive function and contributions to protein homeostasis. These functions are crucial for overall health, emphasizing the importance of this enzyme in biological systems. Understanding trypsin's roles provides foundational knowledge that can guide further research in fields such as nutrition, digestive disorders, and proteostasis-related diseases.
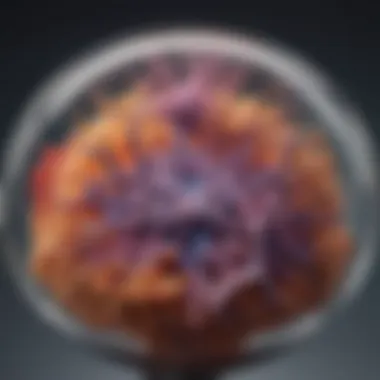
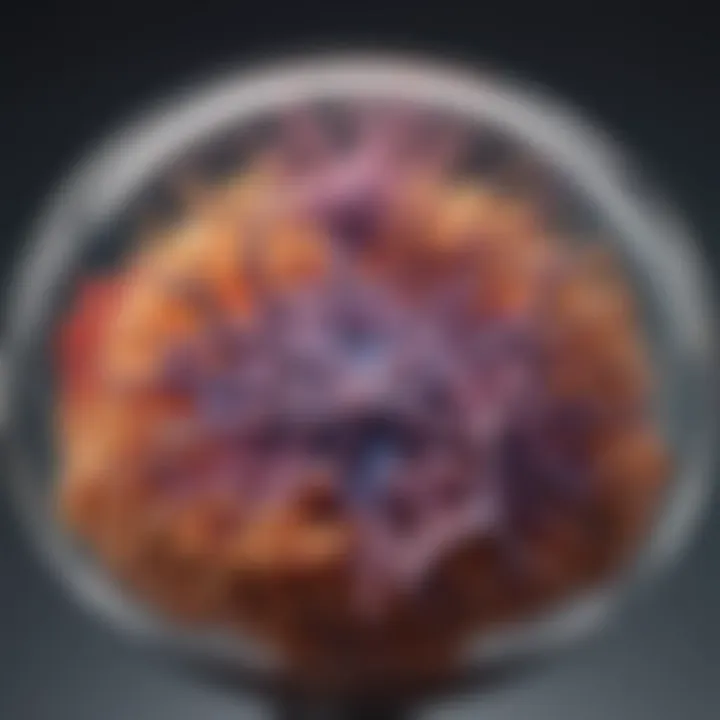
Mechanism of Action
Understanding the mechanism of action of trypsin is crucial in grasping how this enzyme influences various biological processes. Trypsin functions primarily as a serine protease, which means it cleaves peptide bonds in proteins. The ability of trypsin to specifically target and digest proteins is vital for many physiological processes, including digestion and cellular functions.
Substrate Specificity
Trypsin’s substrate specificity is a key element of its function. This enzyme preferentially cleaves peptide bonds at the carboxyl side of the basic amino acids arginine and lysine. Such specificity makes trypsin an essential player in the proteolytic pathways necessary for protein digestion. The enzyme’s active site is structured to accommodate these amino acids, allowing it to effectively break down proteins into smaller peptides.
In biological systems, this targeted action is important for several reasons:
- Nutrient Absorption: By breaking down dietary proteins into absorbable peptides, trypsin aids in nutrient absorption in the intestines.
- Regulatory Functions: The digestion of proteins can release bioactive peptides, influencing various physiological functions like hormones and immune responses.
The understanding of substrate specificity also leads to its applications in experimental and clinical contexts. By knowing how trypsin interacts with specific proteins, researchers can design experiments and therapeutic strategies accordingly.
Activation and Regulation
The activation and regulation of trypsin are essential for its biological roles. Trypsin is synthesized as an inactive precursor called trypsinogen, which is activated in the intestine. This activation is typically triggered by another enzyme called enterokinase. Once activated, trypsin can, in turn, activate other proteolytic enzymes, showcasing a cascade effect which is critical for protein digestion.
Several regulatory mechanisms ensure that trypsin activity is properly controlled:
- Inhibitors: Proteins like trypsin inhibitor play a crucial role in regulating trypsin activity. These inhibitors can bind to trypsin and prevent it from acting on proteins before they are ready for digestion.
- Physiological Conditions: The local environment, including pH and substrate concentration, influences trypsin’s activity. It operates optimally in the alkaline conditions of the small intestine.
Understanding these activation processes and regulatory mechanisms helps ensure that trypsin is only active when and where it is needed, preventing unwanted damage to bodily proteins and tissues. Therefore, knowledge of trypsin’s mechanism of action is not only of academic interest but also of practical importance in fields ranging from biochemistry to medicine.
Regulatory Mechanisms
Understanding regulatory mechanisms governing trypsin activity is essential in comprehending its biological significance. These mechanisms ensure that trypsin functions efficiently while preventing potential damage that could result from unregulated enzymatic activity. Disruptions in such regulation can lead to various diseases, highlighting the importance of studying these mechanisms meticulously.
Inhibitors of Trypsin
Trypsin is regulated primarily through specific inhibitors, which impede its activity. One of the most notable inhibitors is punstatin, a small protein derived from plants. Punstatin can bind to the active site of trypsin, effectively blocking substrate access. This prevents unnecessary degradation of vital proteins, which could disrupt cellular processes.
Moreover, serpin family proteins, such as alpha-1 antitrypsin, also play a crucial role as endogenous inhibitors. These proteins modulate trypsin activity in physiological conditions, ensuring proper protease activity balance. Their effectiveness is vital for maintaining homeostasis in physiological processes, especially in the digestive system and immune responses. Elevated levels of these inhibitors can therefore have therapeutic implications, such as in treating conditions where excessive trypsin activity is detrimental.
Physiological Regulation
The physiological regulation of trypsin involves several factors that maintain its activity within tight limits. Hormonal factors, particularly cholecystokinin, stimulate the release of pancreatic enzymes, including trypsin. This intricate signaling ensures that trypsin is activated only in the presence of adequate food substrates, thus minimizing premature activation.
Furthermore, local pH levels and the presence of bile salts facilitate trypsin’s activation from its inactive precursor, trypsinogen. This activation process occurs in the duodenum, where the conditions are optimal for trypsin’s functionality.
Key Concept: The regulation of trypsin not only relates to its inhibitors but also involves interactions with other hormones and local environmental conditions, underscoring the complexity of enzymatic regulation.
In summary, understanding the regulatory mechanisms of trypsin—through inhibitors and physiological factors—provides critical insights into its role in health and disease. Disruptions in these regulatory pathways can lead to pathological conditions, emphasizing the need for continued research in this area.
Clinical Significance
The clinical significance of trypsin extends well beyond its role in digestion. Understanding trypsin is essential for medical research and practice, particularly concerning its involvement in various diseases and diagnostic applications. Its enzymatic activity and regulation can reveal insights about pathological conditions and potential therapeutic strategies. Given the increasing demand for precision medicine, knowledge about trypsin's clinical roles is becoming increasingly critical.
Trypsin and Disease
Trypsin has been implicated in several diseases, particularly those related to improper protein metabolism. Pancreatitis, for example, is a condition characterized by inflammation of the pancreas and can lead to the premature activation of trypsin within pancreatic tissues. This aberrant activation can cause self-digestion of the pancreas, leading to severe digestive and systemic complications. Additionally, trypsinogen, the inactive precursor of trypsin, has been associated with inflammatory bowel diseases. In these situations, dysregulation of its activation can significantly affect gut health.
Moreover, abnormal trypsin activity is linked to other disorders such as certain cancers. Changes in trypsin levels or activity can serve as biomarkers in cancer diagnostics, indicating the presence or progression of the disease. Understanding the direct relationship between trypsin and these conditions is crucial for developing targeted therapies and improving patient outcomes.
"The connection between enzymatic activity and disease states highlights the pivotal role of enzymes like trypsin in clinical diagnostics and treatment options."
Diagnostic Applications
The diagnostic applications of trypsin are quite expansive. For instance, measuring trypsinogen levels in the blood can help diagnose acute pancreatitis. Elevated levels often indicate an active inflammatory process. Moreover, in the context of gastrointestinal disorders, trypsin assays can reveal information about digestive efficiency and enzyme activity. This can help guide dietary planning and therapeutic interventions.
In addition to these traditional applications, advances in proteomics are paving the way for trypsin's role in biomarker discovery. Its ability to cleave proteins at specific sites makes it invaluable in mass spectrometry for identifying and quantifying proteins in biological samples. This capacity allows researchers to understand disease mechanisms better and develop new diagnostic tests based on protein signatures.
The clinical relevance of trypsin is evident in research and practical applications. Its dual role in disease development and diagnostics underscores the importance of continued investigation into this enzyme. Understanding trypsin in clinical contexts is crucial for enhancing both disease management and therapeutic developments.
Trypsin in Research
The study of trypsin in research offers significant avenues for understanding various biological processes. As an important serine protease, trypsin plays critical roles in protein digestion and metabolism, making it a focal point in proteomics. The insights gained from studying trypsin can contribute to advancements in biotechnology and pharmaceutical development.
Role in Proteomics
Proteomics is the large-scale study of proteins, particularly their structures and functions. Trypsin is frequently utilized in proteomics for its ability to cleave proteins at specific amino acid residues, namely lysine and arginine. This property makes it invaluable for digesting complex protein samples into smaller peptides, which are easier to analyze through techniques like mass spectrometry.
The systematic approach of using trypsin not only enhances the efficiency of protein identification but also increases the accuracy of quantifying proteins within different biological samples. This is especially beneficial when conducting studies related to cell signaling, gene expression, or protein interactions. The use of trypsin can thus foster better understanding of the underlying mechanisms of diseases at the molecular level.
Applications in Drug Development
In drug development, trypsin is central to a range of applications. Its proteolytic activity is employed in designing and testing new therapeutic agents. By modifying trypsin's function or structure, researchers can develop inhibitors that target specific pathways involved in various diseases, such as cancer or autoimmune disorders.
Additionally, trypsin's role in the formulation of biopharmaceuticals is of great interest. Its use in drug delivery systems has been explored to improve the bioavailability of therapeutic compounds. Testing the interactions between drugs and proteins using trypsin-based assays enables the identification of potential drug candidates.
"Understanding the applications of trypsin in drug development can streamline the discovery of effective treatments, especially for complex diseases."
These applications underline the versatility of trypsin in research settings, showcasing its valuable contributions to both fundamental science and practical applications in healthcare.
Biotechnological Applications
Biotechnological applications of trypsin are significant due to its useful enzymatic properties. This section discusses its roles in two major fields: the food industry and biopharmaceuticals. Understanding these applications sheds light on trypsin's potential to improve processes and products in these industries, making it an essential enzyme in modern biotechnological practices.
Use in Food Industry
Trypsin plays a key part in food processing. It is utilized primarily for protein hydrolysis in various food products. By breaking down proteins into smaller peptides, trypsin enhances digestibility and absorption of nutrients.
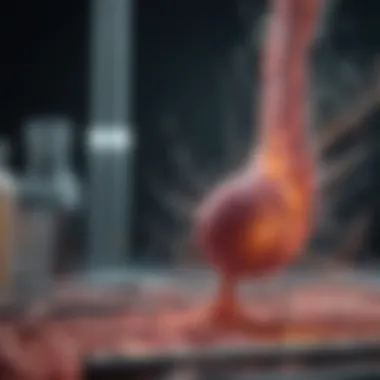
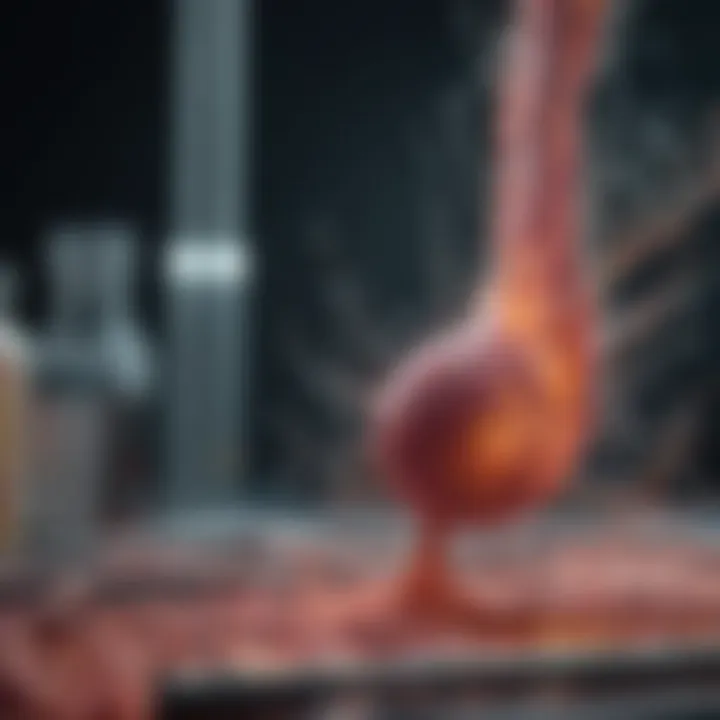
Some of the specific applications include:
- Meat Tenderization: Trypsin is used to tenderize meat. The enzymatic breakdown of muscle fibers improves texture, making it more appealing to consumers.
- Cheese Making: In cheese production, trypsin can help achieve the desired texture and flavor by aiding in the hydrolysis of casein proteins.
- Protein Supplements: In the production of protein hydrolysates, trypsin is used to create supplements that improve absorption rates in the human body.
These applications not only improve quality but also make food products more nutritious and digestible.
Applications in Biopharmaceuticals
In the biopharmaceutical field, trypsin is essential for various processes. Its effectiveness in protein digestion translates to critical roles in the development of therapeutic proteins and vaccines. Here are some notable uses:
- Insulin Production: Trypsin is involved in the processing of proinsulin to form active insulin, a vital hormone for glucose metabolism.
- Vaccine Development: Trypsin-activated cell cultures are often used in vaccine production, helping to propagate viruses in a controlled manner.
- Diagnostic Tools: Trypsin is utilized in assays for immunological and biochemical testing, aiding in disease diagnostics.
In biopharmaceutical manufacturing, the reliability and efficiency of trypsin make it a critical component in ensuring the quality of therapeutic products.
Understanding the biotechnological applications of trypsin reveals its crucial role in enhancing food quality and developing biopharmaceuticals. Beyond these applications, ongoing research seeks to uncover new uses and optimize existing processes, underscoring the enzyme's continuing relevance in biotechnology.
Experimental Techniques Involving Trypsin
Understanding the experimental techniques involving trypsin is pivotal to exploring its functionality. These techniques are essential for researchers who investigate various biochemical processes. Knowing how trypsin can be applied in experiments offers insight into its behavior and roles within living organisms. Not only does this knowledge enhance our understanding of trypsin itself, but it also opens doors for advancements in biotechnological applications.
Enzyme Assays
Enzyme assays are critical in studying trypsin. They measure the activity of the enzyme, providing quantitative data that researchers can analyze to understand how trypsin interacts with different substrates. In general, an enzyme assay involves mixing trypsin with a specific substrate and measuring the rate of reaction over time. There are different approaches to enzyme assays:
- Colorimetric assays: These assays use color change to determine enzyme activity. When trypsin acts on a substrate, the resultant product can change color, indicating reaction progress.
- Fluorometric assays: Here, trypsin cleaves a substrate that releases a fluorescent tag. This fluorescence is then measured to determine the enzyme's activity.
- Kinetic assays: These assess how fast the reaction proceeds. They can also help identify optimal conditions for trypsin activity, such as pH and temperature.
One must consider factors like substrate concentration and potential inhibitors during these assays. Accurate experimental design is vital to ensure reliable results. These enzyme assays are fundamental to understanding trypsin's role in digestion and various metabolic processes.
Protein Digestion Protocols
Protein digestion protocols using trypsin are a staple in both research and industry settings. These protocols leverage trypsin's ability to hydrolyze proteins into peptides, making it valuable for proteomics and other applications. Understanding the steps involved in this process is essential for researchers:
- Sample Preparation: The protein sample must be denatured, which unfolds the protein structure, allowing trypsin access for digestion.
- Trypsin Addition: The trypsin enzyme is added in a controlled manner. Optimal enzyme-to-substrate ratio is crucial for effective digestion.
- Incubation: The mixture is incubated under specific conditions, typically at 37°C, to promote enzymatic activity. The duration of this incubation can affect the extent of digestion.
- Termination of Reaction: After sufficient time, the reaction must be stopped; this can be done using heat or specific inhibitors to ensure no further digestion occurs.
- Analysis: The resulting peptides are analyzed using techniques like mass spectrometry to identify and study their properties.
These protocols are not just academic exercises; they have practical implications in creating peptides for drugs or understanding disease mechanisms. Knowing how to effectively use trypsin in digestion is key for successful applications in various scientific fields.
Future Research Directions
Research on trypsin continues to be a vital area of inquiry, reflecting its fundamental roles in both biological systems and practical applications. Understanding future research directions not only illuminates areas of potential growth in enzymology but also highlights the broad implications of these studies across various fields.
Understanding Trypsin Variants
One significant avenue for further investigation revolves around the various variants of trypsin. These variants can arise from different sources, including species differences, genetic polymorphisms, or post-translational modifications. Identifying and characterizing these variants can yield important insights into their specific functions and regulatory mechanisms. Studying trypsin variants also opens pathways to personalized medicine, as variations may affect how individuals respond to treatments that involve proteolytic activity.
The exploration of trypsin variants necessitates advanced molecular characterization techniques such as mass spectrometry and sequence homology analyses. These methodologies allow researchers to elucidate the structure-function relationships that distinguish each variant. The benefits extend beyond academic interest; understanding these variants can enhance approaches in drug design and therapeutic interventions, especially for disorders where trypsin dysregulation is implicated.
Innovations in Trypsin Applications
Another crucial area is the development of innovative applications of trypsin, particularly in biotechnology and pharmacology. With the advancement of synthetic biology, researchers are increasingly interested in engineering trypsin for specific functions that can improve its efficacy and specificity. This could include the design of novel peptides or use in targeted drug delivery systems that exploit the unique properties of trypsin.
Furthermore, innovations have been seen in food processing where trypsin is utilized to enhance protein digestibility and nutritional value. The food industry continually seeks to improve product formulations, and the application of recombinant trypsin offers possibilities for more sustainable and efficient processes.
The intersection of technology and trypsin research is promising. It creates opportunities for developing more robust diagnostic tools and therapeutic interventions. Exploring these innovations not only contributes to scientific knowledge but also drives economic implications in health care and food industry sectors.
"Research on trypsin variants and innovative applications reflects a pivotal crossroad of biology and technology, promising to unlock new therapeutic avenues and improve product efficacy across industries."
Ethical Considerations
Understanding the ethical considerations surrounding the use of trypsin is important for several reasons. The multifaceted applications of trypsin in research, biotechnology, and clinical settings call for a responsible approach. Ethical scrutiny ensures that the use of this enzyme aligns with not only scientific objectives but also moral standards concerning health and safety. Examining these considerations leads us to two main areas: biosafety and regulatory frameworks. Both elements are critical in guiding the appropriate and responsible use of trypsin in various settings.
Biosafety in Trypsin Use
Biosafety is a significant concern when working with trypsin, especially in research and clinical environments. The enzyme is derived from pancreatic sources, and as such, poses potential risks related to allergenicity and contamination. It is essential to adhere to strict biosafety protocols to minimize these risks while handling trypsin.
Some key aspects of biosafety in trypsin use include:
- Risk Assessment: Identifying potential hazards associated with the use of trypsin, including biological and chemical risks.
- Containment: Employing appropriate containment measures to prevent accidental exposure to the enzyme.
- Training: Ensuring that all personnel involved in handling trypsin are well-trained in safety protocols and understand the potential risks.
- Waste Disposal: Adopting proper disposal methods for trypsin waste to avoid environmental contamination.
These practices are crucial for maintaining a safe working environment and protecting individuals from unforeseen risks associated with trypsin exposure.
Regulatory Frameworks
Regulatory frameworks are instrumental in guiding the ethical use of trypsin in the laboratory and industry. Different countries have established their regulations regarding the use of enzymes in research and products. Ensuring compliance with these regulations is necessary for promoting safety and efficacy in various applications.
Key components of regulatory frameworks surrounding trypsin include:
- Approval Processes: Obtaining necessary approvals before utilizing trypsin in clinical trials or commercial products.
- Labeling Standards: Accurate labeling of products containing trypsin to inform consumers and healthcare providers about its presence and potential risks.
- Monitoring and Compliance: Regular inspections and assessments to ensure that entities using trypsin comply with established safety standards.
These regulatory measures not only safeguard public health but also enhance the credibility and reliability of research findings and product development involving trypsin. In summary, ethical considerations surrounding biosafety and regulatory frameworks are essential in ensuring responsible practices in the utilization of trypsin.
Educational Perspectives
The study of trypsin greatly contributes to educational frameworks, particularly in fields like biochemistry, molecular biology, and biotechnology. Understanding trypsin offers students a practical example of enzyme functionality and its role in various biological processes. In academic settings, the inclusion of topics related to trypsin can enhance learning experiences, illustrating complex biochemical concepts through a concrete subject. This section explores the significance of teaching trypsin within different curricula, as well as the resources available for educators to enrich their teachings.
Teaching Trypsin in Curricula
Teaching about trypsin allows educators to bridge theoretical knowledge with real-world applications. Students learn about protein metabolism, the digestive system, and the implications of enzyme actions in health and disease. The incorporation of trypsin-related content can lead to discussions on a variety of essential subjects, including:
- Enzyme mechanisms: Students can grasp general principles of enzymatic action, such as substrate specificity and catalytic efficiency.
- Clinical relevance: Discussing trypsin's role in diseases fosters critical thinking as students connect biochemistry with health sciences.
- Biotechnological applications: Students gain insights into how enzymes like trypsin are utilized in industry, from food production to pharmaceuticals.
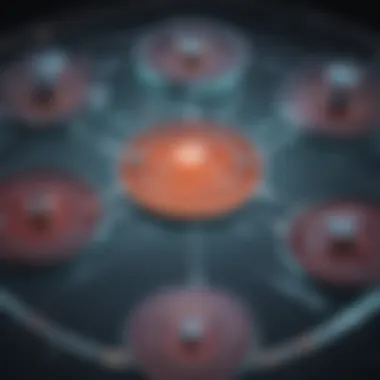
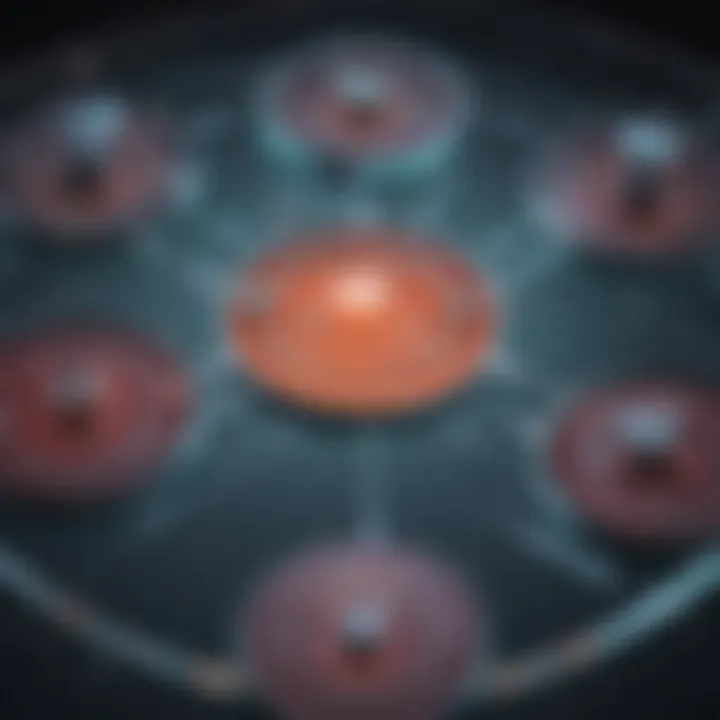
By integrating trypsin into different topics, educators provide a multifaceted perspective. This not only promotes a deeper understanding of enzymology but also showcases its relevance in various scientific domains.
Resources for Educators
To facilitate effective teaching, educators need access to a variety of high-quality resources that provide information on trypsin. Key resources include:
- Textbooks on enzymology: Comprehensive materials furnish foundational knowledge of enzymatic processes. Titles like "Biochemistry" by Berg, Tymoczko, and Stryer are beneficial for students.
- Online databases and journals: Websites such as britannica.com and en.wikipedia.org serve as excellent sources for up-to-date information and research findings on trypsin and related topics.
- Lab experiment protocols: Hands-on experiments reinforce theoretical knowledge, offering students practical skills. For example, enzyme assays and protein digestion protocols can help them visualize trypsin's effects. Resources can often be found on educational platforms or through university repositories.
- Video tutorials: Short educational videos can aid visual learning, making complex processes more digestible.
Ultimately, providing robust resources empowers educators to teach trypsin effectively, enhancing students’ comprehension and interest in enzymatic functions.
"Engaging students with real-world examples of enzymes can enhance their learning experience and motivation."
By focusing on these educational perspectives, we not only honor the scientific significance of trypsin but also prepare students to engage critically with biochemical concepts.
Comparative Enzymology
Comparative enzymology serves as a vital lens through which researchers can analyze the functional capabilities and biochemical characteristics of various enzymes, including trypsin. This field enables a detailed examination of enzymes' similarities and differences, which is crucial for understanding how specific enzymes, such as trypsin, operate within biological systems. By exploring comparative enzymology, scientists can gain insights into enzyme specificity, reaction mechanisms, and the evolution of enzymatic functions.
Trypsin Versus Other Proteases
Trypsin, as a serine protease, is often compared to other proteases to highlight its unique properties. Unlike pepsin, which functions in acidic environments, trypsin operates optimally in basic conditions, reflecting its role in the small intestine. This specificity for basic pH conditions allows trypsin to effectively cleave peptide bonds in polypeptides, particularly those adjacent to the amino acids lysine and arginine.
Some key differences between trypsin and other proteases include:
- Specificity: Trypsin's selectivity for specific amino acid residues is distinct from other proteases, such as chymotrypsin, that may exhibit broader substrate recognition.
- Mechanism of Action: The catalytic mechanism of trypsin involves the formation of an acyl-enzyme intermediate, which is a different approach compared to some cysteine proteases.
- Regulation: The inhibition of trypsin showcases a complex regulatory mechanism often influenced by physiological factors in the digestive system, in contrast to other proteases that may have simpler inhibition pathways.
Through comparative analysis, the understanding of trypsin can be greatly enhanced, leading to its application in medical diagnostics and treatments of digestive disorders. This necessitates clear distinctions in how different proteases function, which underlines the importance of trypsin in the proteolytic landscape.
Functional Diversity Among Enzymes
The functional diversity observed among enzymes illustrates the adaptation of these proteins to various biological roles. Trypsin is a prime example of such diversity, as it is tailored for specific reactions in diverse environments. Factors contributing to this functional variation include:
- Protein Engineering: Advances in biotechnology allow for modifications of enzymes to enhance their activity or stability. For example, engineered trypsin variants may show increased tolerance to temperature or pH extremes.
- Evolutionary Adaptations: Understanding the evolutionary trajectory of trypsin and its relatives provides insights into how different proteases have adapted to their roles across different species. This can lead to discoveries in enzyme function and applications.
- Biochemical Pathways: Enzymes often function in concert within metabolic pathways. An understanding of trypsin's role can reveal its interplay with other enzymes in protein digestion and metabolism.
"The study of enzyme functionality not only furthers our understanding of biological processes but also enhances our ability to manipulate these processes for therapeutic purposes."
The significance of comparative enzymology cannot be understated, as it enriches the knowledge base regarding trypsin and furthers applications in biotechnology, pharmaceuticals, and diagnostics. This knowledge is invaluable for researchers and professionals aiming to leverage enzyme efficiencies in various scientific fields.
Implications for Biotechnology
Understanding the implications of trypsin in biotechnology is of great significance. This enzyme is not only pivotal in biological systems but also serves as a fundamental tool in various biotechnological applications. Its properties offer unique benefits that resonate in fields such as molecular biology, protein engineering, and therapeutic development. By exploring these implications, we can appreciate trypsin's role in enhancing biotechnological processes and innovations.
Trypsin and Genetic Engineering
Trypsin plays a substantial role in genetic engineering. It is widely used in gene expression studies, especially in cloning and vector systems. Through its unique ability to cleave proteins after specific amino acids, researchers can manipulate protein structures, facilitating the study of protein functions and interactions. For instance, trypsinization is used to digest proteins into smaller peptides, which is essential for mass spectrometry and peptide mapping. This step is crucial for analyzing protein changes caused by genetic alterations.
Furthermore, trypsin is also applied in the production of recombinant proteins. By using trypsin to cleave fusion tags, scientists can purify desired proteins from complex mixtures effectively. This is particularly beneficial in therapeutic protein production, where high specificity and purity are required.
In summary, trypsin's unique characteristics make it invaluable in genetic engineering, allowing for advancements in our understanding and manipulation of genetic materials.
Enzyme Engineering Approaches
Enzyme engineering approaches have been enhanced through the study of trypsin. Researchers focus on optimizing its activity and stability to improve its efficiency for various applications. One method involves the modification of trypsin's catalytic properties through site-directed mutagenesis, enabling the creation of variants with tailored functionalities. Such engineered variants can exhibit improved substrate specificity, thermal stability, and resistance to inhibitors.
In addition, trypsin can be utilized as a model in enzyme engineering to design other proteases. By understanding its structure and function, biochemists can develop enzymes with enhanced attributes suitable for specific industrial processes. This plays a critical role in sectors such as pharmaceuticals, food processing, and biofuels.
Moreover, immobilization techniques are applied to trypsin, enabling it to be reused in batch processes while maintaining its activity. This not only reduces costs but also enhances enzymatic efficiency in various applications, such as biocatalysis and biosensors.
Understanding trypsin's role in biotechnology opens doors to innovative applications that can effectively serve various industries.
These discussions illustrate that trypsin is not merely an enzyme of biological interest; its versatility and functional proficiencies make it a cornerstone for developments in biotechnology.
Trypsin Isolation and Purification
Isolating and purifying trypsin is a crucial step in the study and application of this enzyme. Pure trypsin is essential for many experimental and clinical uses. In research, it serves as a model protease to understand enzymatic mechanisms and protein interactions. In biotechnology, trypsin has applications in both the food industry and pharmaceuticals. The benefits of isolation include enhanced specificity, activity, and reduced interference from other proteins.
When discussing isolation and purification, several factors must be considered, including yield, purity, and the method's efficiency. The process must also ensure that the enzymatic activity of trypsin is retained. Any loss of activity can diminish the usefulness of the enzyme in subsequent applications.
Techniques for Purification
The purification of trypsin involves various techniques. Here are some of the most commonly used methods:
- Affinity Chromatography: This technique uses a specific ligand to selectively bind trypsin. Once bound, impurities can be washed away, and trypsin can be eluted in a pure form.
- Ion-Exchange Chromatography: In this method, proteins are separated based on their charge. Trypsin is eluted from the column by changing the ionic strength of the buffer, allowing for selective purification.
- Gel Filtration Chromatography: This separation technique is based on the size of molecules. Smaller molecules pass through the column, while larger ones are retained. Since trypsin is relatively small, it can be successfully isolated by this method.
- Precipitation: Ammonium sulfate precipitation is commonly used. By adding ammonium sulfate, proteins can be selectively precipitated out of the solution. After this, further purification techniques can be applied.
Successful isolation of trypsin not only enhances its applicability but also provides essential data on its biochemical characteristics.
Each method has its advantages and disadvantages. The choice of technique often depends on the specific requirements of the study or application. Balancing efficiency with the preservation of enzymatic activity is key to achieving a successful purification process.
Concluding Remarks
The concluding section of this article emphasizes the significance of trypsin in both biological mechanisms and broader applications. It is crucial to synthesis the interconnected aspects of trypsin's structure, function, and regulation. By understanding these facets, we can appreciate trypsin’s role not just as a digestive enzyme but also as an essential tool within various scientific realms.
A central benefit of studying trypsin lies in its ability to facilitate protein digestion. This property is especially important in fields such as nutrition and medicine. Additionally, the insights gained through the analysis of trypsin can direct future research endeavors, guiding scientists towards innovations in proteomics and biotechnology.
Key considerations include:
- The diverse roles of trypsin in metabolic processes.
- The implications of trypsin regulation on health and disease.
- Its utility in diagnostic applications and therapeutic research.
Understanding these elements fosters an acknowledgment of how trypsin's characteristics relate to both biochemical pathways and practical applications. This integrated perspective enhances our knowledge and appreciation for the complexity of proteolytic enzymes.
Summary of Key Points
In this article, we discussed the following key points regarding trypsin:
- Definition and Historical Context: We provided a thorough definition of trypsin and traced its historical significance in enzyme research.
- Molecular Composition: Insights into trypsin’s amino acid structure and active site offered a clearer view of its enzymatic functions.
- Biological Functions: Discussion included trypsin’s role in digestion and maintaining protein homeostasis.
- Mechanistic Insights: We examined substrate specificity and the mechanisms of activation and regulation, showcasing how trypsin operates effectively in biological settings.
- Clinical and Research Relevance: The article highlighted trypsin’s involvement in disease mechanisms and its applications in medical diagnostics and research fields.
- Biotechnological Applications: Trypsin’s versatility in the food industry and biopharmaceuticals showcased its relevance beyond basic biology.
- Experimental Approaches: Techniques in enzyme assays and protein digestion provided practical perspectives on working with trypsin.
- Future Directions: We outlined areas for future research, particularly in understanding trypsin variants and innovations in applications.
- Ethical Considerations: Finally, attention to biosafety and regulations emphasized the importance of responsible trypsin use in research and industry.
The comprehensive nature of this analysis underscores trypsin’s indispensable role across various scientific disciplines and its continuing relevance in research and therapeutic contexts.