Exploring Tungsten and Rhenium: Properties and Advances
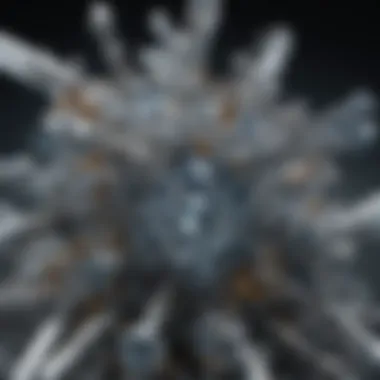
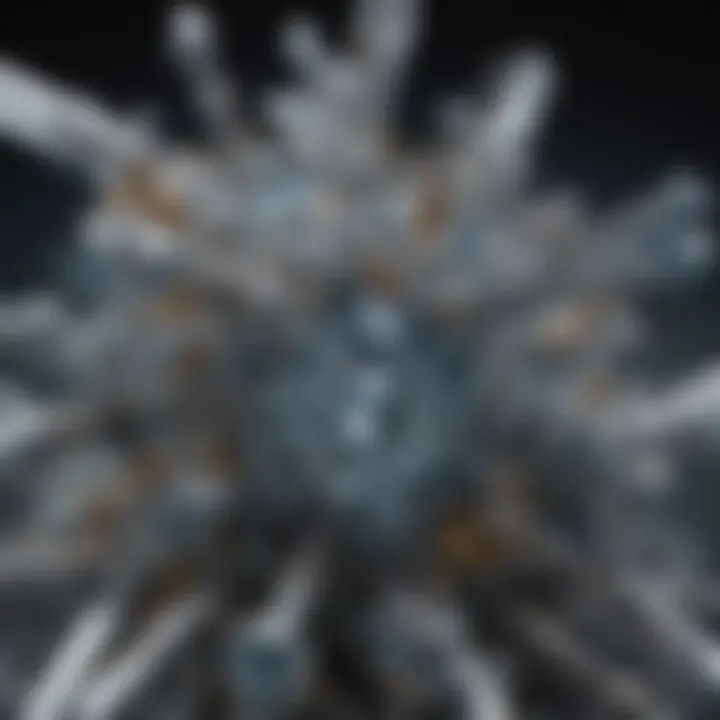
Intro
Tungsten and rhenium are transition metals that belong to the d-block of the periodic table. These elements exhibit unique properties that make them useful in a variety of scientific and industrial applications. Tungsten is known for its high melting point and density, while rhenium stands out for its remarkable catalytic properties and resistance to thermal degradation. The intersection of these two elements invites exploration into how their characteristics can be harnessed effectively.
In various applications, the synergistic effects of tungsten and rhenium can open doors to new technologies. From aerospace engineering to electronics, understanding their properties and interactions can lead to significant advancements.
This article will present an overview of research on tungsten and rhenium, including their individual and collaborative properties. Specific attention will be given to their significance in current industry practices and future technological innovations.
Intro to Tungsten and Rhenium
Tungsten and rhenium are two significant elements in modern science and technology. Understanding their properties and applications is crucial for both academic and industrial fields. Tungsten, known for its exceptional hardness and high melting point, has become essential in various applications ranging from electronics to aerospace. Rhenium, on the other hand, is less common but is equally valuable due to its unique chemical properties and high-temperature capabilities.
The interaction between tungsten and rhenium creates a synergy that enhances material performance, making them valuable in alloy forms. This article examines the importance of these elements, focusing on their shared characteristics, applications, and future research opportunities.
Historical Context
Tungsten was first discovered in the late 18th century by the chemist as Juan José de Elhuyar and his brother, while rhenium was discovered in 1925 by Walter Noddack, Ida Tacke, and Otto Berg. These discoveries opened new gateways in metallurgy and material science. Tungsten quickly found applications in the production of strong and durable materials. It became vital in military and industrial contexts, especially during the world wars. Additionally, rhenium’s unique properties such as its high melting point and ability to form superalloys contributed significantly to its use in aerospace and catalytic converters in the automotive industry. Overall, the historical development of these elements illustrates their growing importance and relevance in various technological advancements.
Occurrence in Nature
Both tungsten and rhenium are not abundant in the Earth's crust. Tungsten typically occurs in minerals such as wolframite and scheelite. Rhenium is much rarer and is often found in the ores of molybdenum and copper. Their rarity influences their industrial applications and market dynamics. Often, the recovery process for these elements can be expensive and complicated, which raises considerations regarding resource management and sustainability. As demand for advanced materials grows, more exploration into their natural occurrence and extraction processes becomes essential.
Chemical Properties of Tungsten
The chemical properties of tungsten define its behavior and interactions in various environments. Understanding these properties is essential for scientists and engineers alike. Tungsten's unique atomic structure contributes to its remarkable characteristics, including its high melting point and density. These elements make tungsten an ideal candidate for numerous industrial applications.
Atomic Structure
Tungsten’s atomic structure is paramount to its chemical properties. It has an atomic number of 74 and an atomic mass of approximately 183.84 g/mol. Its electron configuration is [Xe] 4f¹⁴ 5d⁴ 6s², which indicates a complex arrangement of electrons that contribute to its stability. The strong bonding forces within its atomic lattice explain tungsten's exceptional hardness and toughness. In the periodic table, tungsten belongs to group 6, indicating that it has similar traits to other transition metals, like molybdenum. This resemblance means tungsten also shares certain reactivity properties, which are crucial for its applications in various fields.
Reactivity and Compounds
Tungsten displays notable resistance to corrosion and oxidation at room temperature, making it a stable element in many environments. However, when subjected to high temperatures, it may react with halogens or acids. For example, tungsten can form compounds with halogens such as tungsten hexafluoride (WF₆) and tungsten dichloride (WCl₂). Its ability to form these compounds underlines its importance in chemical processes. Additionally, tungsten can create alloys that enhance its reactivity and usability in applications ranging from electronics to aerospace. The conjunction of tungsten with other elements can yield compounds with significant mechanical and thermal properties, further enhancing its applicability in technology and industry.
Tungsten's unique reactivity and the resultant compounds are critical in various technological advancements.
In summary, the chemical properties of tungsten, particularly its atomic structure and reactivity, play a vital role in its applications. These characteristics make tungsten not only important but essential in many technological processes.
Chemical Properties of Rhenium
Rhenium, a transition metal in group 7 of the periodic table, possesses unique chemical properties that distinguish it from many other elements. These properties are central to its applications and significance in various industries. Understanding the atomic features of rhenium, along with its behavior in alloys and compounds, is crucial for researchers and professionals working with this element.
Atomic Features
Rhenium has an atomic number of 75 and is characterized by its dense and heavy nature. Its atomic structure consists of a relatively high atomic mass, a notable trait that impacts its physical and chemical stability. Rhenium exhibits a unique electron configuration, which is [Xe] 4f14 5d5 6s2. This configuration contributes to its ability to form complex compounds and participate in various reactions.
The presence of five unpaired electrons in its d-orbitals allows rhenium to engage in numerous oxidation states ranging from -1 to +7. This versatility in oxidation state is important. For example, its higher oxidation states, such as +7, enable it to act as a strong oxidizing agent, while its lower states contribute to its stability in various compounds, notably those used in catalytic reactions.
Moreover, rhenium’s ability to form stable complexes with various ligands is significant in industrial processes. This includes its use in catalytic converters and other chemical reactions where precision is crucial. The understanding of rhenium’s atomic features informs innovations in chemical manufacturing, metallurgy, and technology.
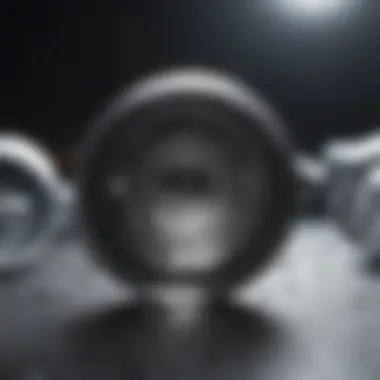
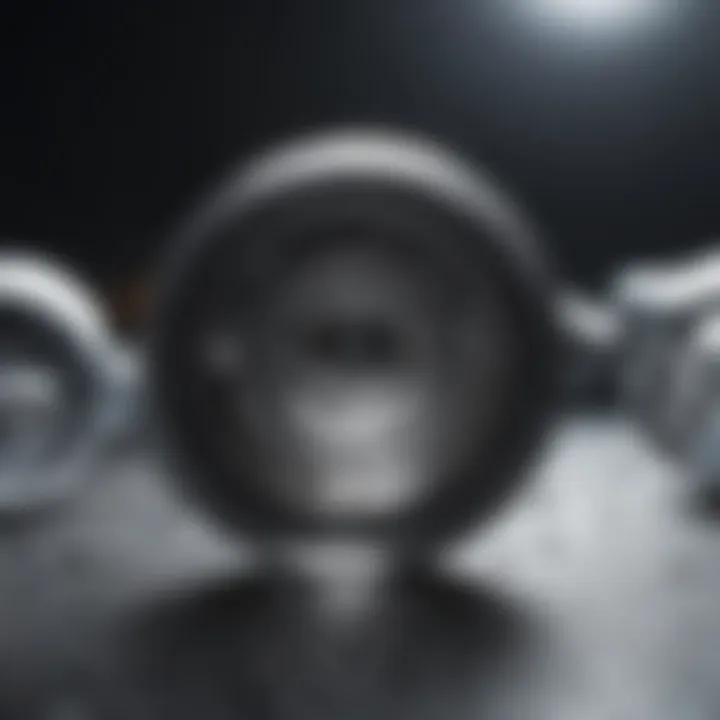
Alloying and Compound Behavior
Rhenium’s properties extend to its behavior when alloyed with other metals, most notably in high-performance applications. When combined with other elements like tungsten, it significantly improves hardness and heat resistance of the resulting alloys. These alloys find applications in aerospace and mechanical industries where durability and strength under extreme conditions are paramount.
Rhenium is also utilized in superalloys. These are designed to endure extreme temperatures and mechanical stress. The inherent properties of rhenium enhance the resistive qualities of such materials, making them invaluable in gas turbines and rocket engines.
Furthermore, rhenium forms a variety of compounds, notably rhenium disulfide (ReS2) and rhenium oxides. Rhenium disulfide, in particular, has garnered attention for its potential applications in electronics and nanotechnology due to its semiconducting properties.
In addition to these strong industrial applications, environmental considerations are crucial when discussing rhenium. The recovery of rhenium from mining processes raises concerns, and its substance can impact ecosystems. Therefore, understanding both its alloying and compound behaviors facilitates informed decision-making regarding its use and environmental implications.
"Rhenium's remarkable reaction versatility and its ability to form stable alloys make it critical in modern material science and technology."
Overall, the chemical properties of rhenium are pivotal not only for its role in existing technologies but also for future advancements in research and industrial practices.
Shared Characteristics of Tungsten and Rhenium
The exploration of shared characteristics between tungsten and rhenium reveals fundamental aspects that contribute to their significance in various applications. Both elements exhibit remarkable features that cater to demanding industrial needs. Understanding these characteristics helps in appreciating their roles in technological innovations and their potential for future applications.
High Melting Points
Tungsten and rhenium are distinguished by their exceptionally high melting points. Tungsten has a melting point of approximately 3,422 °C (6,192 °F), while rhenium follows closely with a melting point of 3,186 °C (5,767 °F). This unique trait allows both metals to maintain structural integrity under extreme thermal conditions. For instance, in aerospace engineering and high-temperature applications, materials must endure severe thermal stresses without deformation.
Due to their high melting points, tungsten and rhenium also serve critical functions in electrical contacts and filaments. They can operate effectively in high-temperature environments, making them ideal for specialized applications in electronics, such as cathodes in electron microscopes and high-intensity lamps.
Furthermore, this high thermal resistance enables advancements in fields such as nuclear applications and materials science. Components made with tungsten and rhenium can endure greater thermal energy, reducing the need for frequent replacements and boosting efficiency in processes that demand durability and reliability.
Mechanical Strength
Mechanical strength is another significant shared characteristic of tungsten and rhenium. Tungsten features one of the highest tensile strengths among metals, a property that is complemented by rhenium's impressive capabilities. Rhenium offers excellent ductility, which allows it to be shaped and formed without loss of strength.
This duality of strength and ductility means that alloys combining these two metals are exceptionally resilient. Such tungsten-rhenium alloys are often utilized in applications where both stress resistance and thermal stability are essential. The aerospace and defense sectors benefit greatly, as components in this context require materials capable of withstanding high stress and fatigue.
Moreover, these mechanical properties pave the way for innovative uses in medical devices and tools that require precision and reliability. The robustness of tungsten and rhenium ensures that they can operate in critical applications, such as surgical instruments, where both strength and precision are paramount.
"The combination of high melting points and mechanical strength makes tungsten and rhenium essential in fields requiring exceptional materials."
In summary, the exploration of high melting points and mechanical strength highlights how tungsten and rhenium share essential attributes. These characteristics not only enhance their individual applications but also promote the development of advanced materials leading to technological improvements across multiple sectors.
Applications of Tungsten in Industry
Tungsten is a remarkably versatile metal, with applications that span various industries. Its unique properties, such as high melting point, density, and strength, make it a key material in numerous technological advancements. In this article section, we will delve into two major areas where tungsten plays an essential role: electronics and semiconductors, as well as aerospace engineering.
Electronics and Semiconductors
In the realm of electronics and semiconductors, tungsten is revered for its extraordinary conductivity and resistance to heat. These properties are critical for ensuring stability in devices that operate under high temperatures. Tungsten is often used in making the contacts of integrated circuits and transistors, due to its ability to withstand thermal and electrical stress.
- Wolfram Process: One popular method of integrating tungsten into electronics is through the Wolfram process. In this process, tungsten is deposited onto silicon substrates to form conductive paths essential for circuit functionality.
- High Performance: Tungsten's resistance to electromigration, a process that can degrade metal connections in semiconductors over time, enhances the reliability of electronic products. As devices become smaller and more complex, having a material that performs under these demanding conditions is increasingly crucial.
Moreover, tungsten’s role in the manufacturing of cathodes for gas discharge lamps and LEDs highlights its importance in energy-efficient lighting. The thermal stability combined with excellent electron emission properties makes tungsten an ideal choice for applications requiring lasting performance. These features contrast starkly with materials that may not hold up as effectively under similar conditions.
Aerospace Engineering
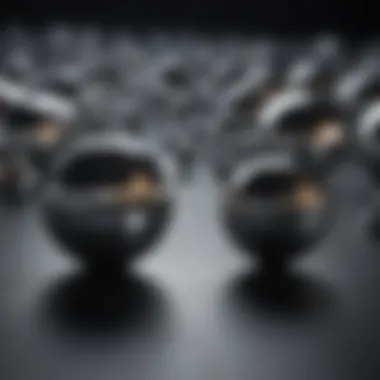
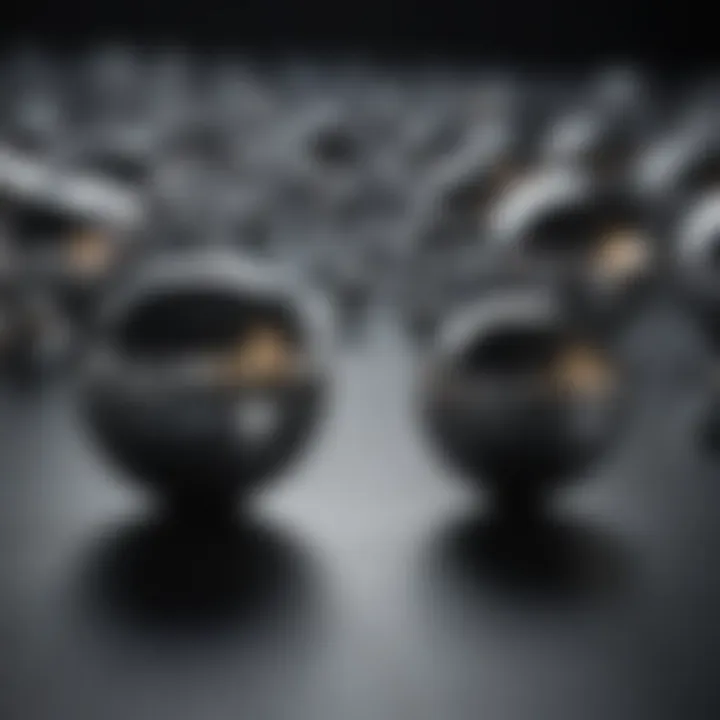
Tungsten's strength and high melting point substantially contribute to its use in aerospace engineering. The aerospace sector requires materials that can withstand extreme conditions, including high temperatures and pressures, where failure can lead to catastrophic results.
- Engine Components: In this context, tungsten is commonly found in engine components such as turbine blades and nozzle guide vanes. Its high mechanical strength allows these parts to endure thermal shock and intense aerodynamic forces, which are prevalent during flight.
- Radiation Shielding: Tungsten also finds applications in radiation shielding for space exploration. The metal's density offers exceptional protection against cosmic rays and other forms of radiation that can pose risks to both equipment and human life in space.
The ongoing advancements in aerospace technology continue to call for more efficient and durable materials, and tungsten stands at the forefront of meeting these demands. The integration of tungsten into various aerospace applications illustrates its importance in enhancing safety and performance in an industry that is critical to modern transportation and exploration.
Tungsten's unique properties are essential for maintaining functionality and reliability in both electronic devices and aerospace applications.
Applications of Rhenium in Industry
Rhenium is an increasingly valuable metal due to its unique properties and diverse applications across several industries. In this section, we will explore the critical roles that rhenium plays, focusing on catalytic processes and high-temperature superconductors. The importance of understanding rhenium's applications cannot be overstated, especially as the demand for sustainable and efficient technologies continues to grow.
Catalytic Processes
Rhenium is known for its significant role as a catalyst in various chemical processes. It is especially prominent in the oil refining industry, where it is utilized to improve the efficiency of catalytic reforming. This process converts crude oil into high-octane gasoline, which is vital for the automotive industry. Rhenium-based catalysts enhance the quality of refined products while reducing the energy required during processing.
Moreover, rhenium is also employed in the production of chemicals such as hydrogen sulfide and ammonia. Rhenium's catalytically active form facilitates these reactions, promoting more accessible pathways that result in higher yields and lower energy consumption. The effectiveness of rhenium in these reactions can be attributed to:
- High thermal stability which allows it to perform at elevated temperatures
- Exceptional selectivity that minimizes unwanted by-products
- Resistance to poisoning from impurities found in feedstock
In addition, recent advancements in nanotechnology have led to the development of rhenium-based nanocatalysts. These catalysts provide enhanced surface area and reactivity, further optimizing chemical processes. As industries seek more efficient ways to handle raw materials, the importance of rhenium in catalysis will only continue to rise.
High-Temperature Superconductors
Rhenium is also gaining recognition in the field of superconductors. It has unique electrical properties which make it a suitable candidate for high-temperature superconductors. These materials can conduct electricity with zero resistance at elevated temperatures, which is essential for various applications including magnetic resonance imaging (MRI) and particle accelerators.
The addition of rhenium helps improve the stability and performance of these superconductors. In specific superconductor compounds, rhenium contributes to:
- Increased critical temperatures, enhancing operational efficiency
- Better mechanical properties, allowing devices to withstand greater stress
- Doped structures that enhance electrical transport properties
As research advances, the demand for high-temperature superconductors in industries like healthcare and energy generation continues to grow. Rhenium's role in this sector implies future enhancements in both the performance and capability of these materials.
In summary, rhenium's applications in catalytic processes and high-temperature superconductors underline its importance in modern industry. The understanding of these applications not only sheds light on rhenium's unique properties but also highlights its potential in addressing future engineering challenges.
Tungsten-Rhenium Alloys
Tungsten-rhenium alloys occupy a critical niche in materials science due to their excellent combination of physical and chemical properties. The synergy between tungsten and rhenium results in an alloy that exhibits high strength, durability, and resistance to extreme conditions. These attributes make tungsten-rhenium alloys particularly valuable in applications where performance cannot be compromised.
Research on these alloys indicates that their unique composition allows for significant enhancement in mechanical properties, including tensile strength and hardness. The addition of rhenium to tungsten not only improves the alloy’s ability to withstand high temperatures but also enhances its machinability. This makes the alloy suitable for applications in both aerospace and medical fields.
Composition and Structure
The composition of tungsten-rhenium alloys generally consists of varying percentages of tungsten and rhenium, commonly in the range of 1% to 50% rhenium by weight. Each alloy variation offers distinct characteristics suited for specific applications. The structure of these alloys predominantly retains the face-centered cubic lattice of rhenium while integrating the body-centered cubic structure of tungsten. This complex microstructure significantly contributes to its high melting point and stability under stress.
The alloy's microstructure is further optimized through heat treatment processes. This can refine the grain size, enhancing strength and ductility. Specific ratios can be tailored to achieve desired properties for particular applications, providing flexibility in design without sacrificing performance.
Mechanical Properties
Mechanical properties of tungsten-rhenium alloys are exceptional. These alloys exhibit superior tensile strength when compared to pure tungsten or rhenium individually. Their ability to maintain integrity at elevated temperatures is also noteworthy, thus expanding their utility in extreme environments. Among the noteworthy mechanical properties are:
- Tensile Strength: Tungsten-rhenium alloys demonstrate high tensile strength, making them ideal for load-bearing applications.
- Hardness: The hardness of these alloys is considerably enhanced, allowing them to resist wear and deformation under mechanical stress.
- Ductility: While being strong, they also maintain a level of ductility, which allows for processing without cracking.
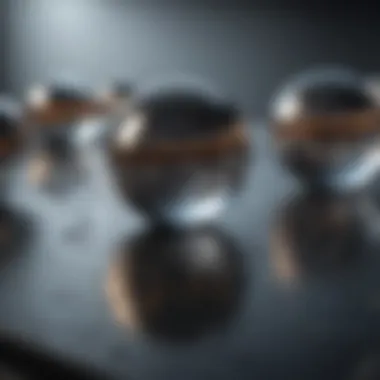
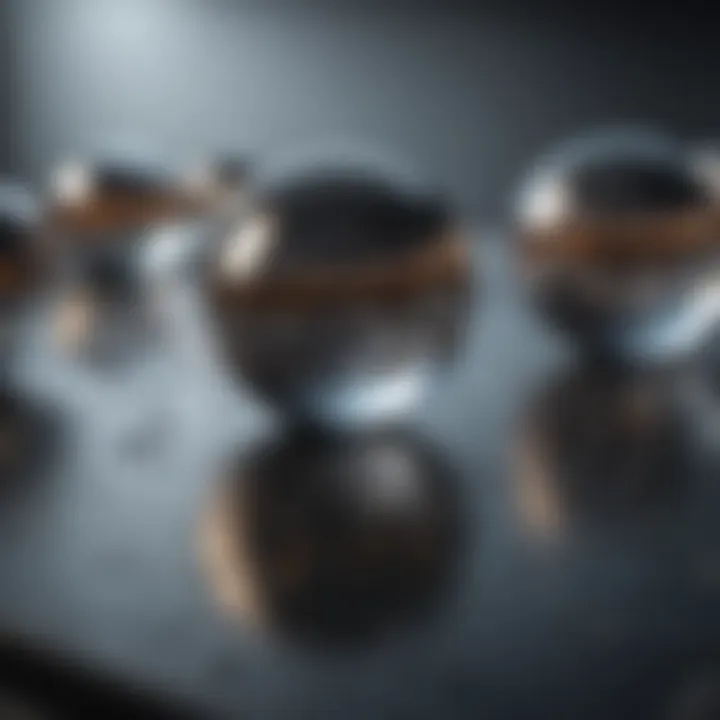
These mechanical properties make tungsten-rhenium alloys suitable candidates for various applications, including electron microscope filaments, specialized aerospace components, and high-temperature thermocouples. The versatility of tungsten-rhenium alloys indeed supports their growing use across industries.
"The remarkable properties of tungsten-rhenium alloys make them essential in both high-performance and specialized applications, continuing to drive advancements in materials science."
Challenges in the Use of Tungsten and Rhenium
The utilization of tungsten and rhenium, while significant in numerous industrial applications, is not without challenges. Understanding these obstacles is critical for researchers and professionals working with these materials. The two primary challenges that emerge in the discussion of tungsten and rhenium are resource scarcity and environmental concerns.
Resource Scarcity
Resource scarcity is one of the main challenges in using tungsten and rhenium. Both elements are not abundantly found in nature. Tungsten is primarily obtained from the mineral scheelite, and its availability is often tied to geopolitical factors and mining operations. Rhenium, on the other hand, is typically derived as a by-product of molybdenum and copper processing. The demand for both materials has increased substantially due to their unique properties.
However, this rising demand contrasts sharply with the limited supply. The mining and extraction processes can be hindered by various factors such as economic viability, environmental regulations, and technological limitations. Consequently, some industries may face difficulties in procuring the amounts required for their applications, which can lead to increased costs and supply chain instability. To alleviate this issue, ongoing research emphasizes the importance of recycling and effective resource management. Enhancing extraction methods and developing alternative materials could also help mitigate the effects of scarcity.
Environmental Concerns
Another challenge related to tungsten and rhenium is the environmental impact associated with their extraction and use. The mining processes for both elements can result in significant ecological disruption. For tungsten mining, land degradation and water pollution are particularly concerning. The concentration of heavy metals and other pollutants during extraction can adversely affect local ecosystems and water sources.
Rhenium production, while less frequent, still raises environmental issues. The refining processes often generate hazardous waste, affecting surrounding communities. The emissions from various processes can also contribute to air pollution.
Given these environmental implications, there is a pressing need for industries to adopt sustainable practices. Implementing modern technologies that minimize waste, enhance process efficiency, and promote responsible mining can be essential steps toward reducing the environmental footprint of tungsten and rhenium utilization. Addressing these environmental concerns is a crucial step toward fostering a more sustainable future in the technological landscape.
As industries increasingly rely on tungsten and rhenium, a balance must be struck between extraction, use, and environmental responsibility.
Future Research Directions
The exploration of tungsten and rhenium is not merely an academic exercise; it possesses significant implications for industries and technologies. Understanding future research directions is crucial for unlocking the full potential of these elements. This section examines innovative applications and sustainability practices that can arise from continued study. The importance of this topic lies in its ability to address both current technological challenges and future needs in an ever-evolving landscape.
Innovative Applications
As industries continue to push the boundaries of what is possible, the innovative applications of tungsten and rhenium emerge as a focal point for research. These elements have unique properties that lend themselves well to cutting-edge technologies.
- Aerospace Engineering: Tungsten-rhenium alloys can withstand harsh temperatures and pressures. As aerospace design advances, these materials may become integral to engine components and thermal protection systems, enhancing performance and safety.
- Catalysis: Rhenium's role as a catalyst in refining processes is well established. However, ongoing research could uncover new catalytic applications, perhaps in the synthesis of valuable chemicals or fuels, thereby improving efficiency and reducing environmental impact.
- Electronic devices: The electronics industry is also a promising area. Research may lead to the development of more efficient semiconductors using tungsten and rhenium, which can support faster and more efficient computing technologies.
The combination of tungsten and rhenium offers opportunities to create materials with superior properties, which could open doors to entirely new applications.
Sustainability Practices
Sustainability has emerged as a vital area of focus across all industries. The research exploring tungsten and rhenium should integrate sustainability at its core. This aspect can manifest in several ways, helping to mitigate the environmental impacts of extraction and manufacturing:
- Recycling and Reuse: Utilizing these metals effectively by focusing on recycling methods can reduce dependency on mined resources, which are often environmentally damaging. Research into advanced recycling techniques could provide innovative ways to reclaim tungsten and rhenium from old devices.
- Green Extraction Methods: Exploring less invasive extraction techniques could minimize the ecological footprint. Research aimed at developing biotechnological methods may reduce waste and pollution associated with traditional mining processes.
- Lifecycle Analysis: A thorough assessment of the lifecycle impacts of tungsten and rhenium products can guide sustainable practices. Understanding impacts from extraction, manufacturing, usage, and disposal will inform better practices in industry.
In summary, future research directions for tungsten and rhenium are pivotal. The focus on innovative applications and sustainable practices can provide significant benefits, addressing both technological advancement and environmental responsibility. Integrating these concerns can lead to a more holistic approach to using these valuable elements.
End
Understanding the intersection of tungsten and rhenium highlights their integral role in modern technology and materials science. This conclusion aims to synthesize the key insights from the previous sections while considering the broader implications of these elements in various applications.
Summarizing Key Insights
The article has traversed the unique properties of tungsten and rhenium, their applications across multiple industries, and the advancements made possible through their alloying. Key insights include:
- Chemical Properties: Both elements exhibit distinct chemical behaviors that are essential in various reactions, enabling enhanced performance in materials.
- Applications: Tungsten finds substantial use in electronics, aerospace, and cutting tools, while rhenium is pivotal in catalytic processes and superconductors. Their collaborative efforts in alloys enhance mechanical properties as well.
- Challenges: Resource scarcity and environmental concerns loom over the continued exploitation of these materials, necessitating better practices.
- Future Directions: Research into innovative applications and sustainable sourcing practices will dictate the future landscape of tungsten and rhenium usage.
Final Thoughts on Tungsten and Rhenium's Future
The future of tungsten and rhenium seems promising yet challenging. Ongoing research is directed towards refining their applications to meet both technological demands and environmental standards. Their durable and high-performance characteristics make them vital for the development of advanced materials. However, as industry shifts towards sustainability, innovation will be critical. Finding methods to recycle and economically source these metals will influence their role significantly in the coming years. The interplay between scientific advancement and ecological stewardship will define how tungsten and rhenium contribute to future technological landscapes.