Understanding FTIR Technology: Principles and Applications
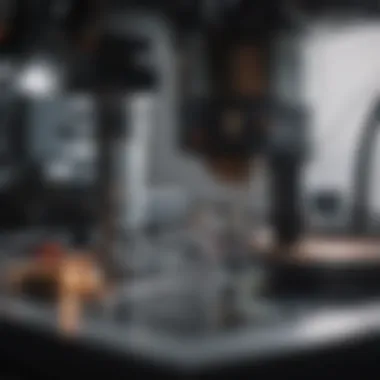
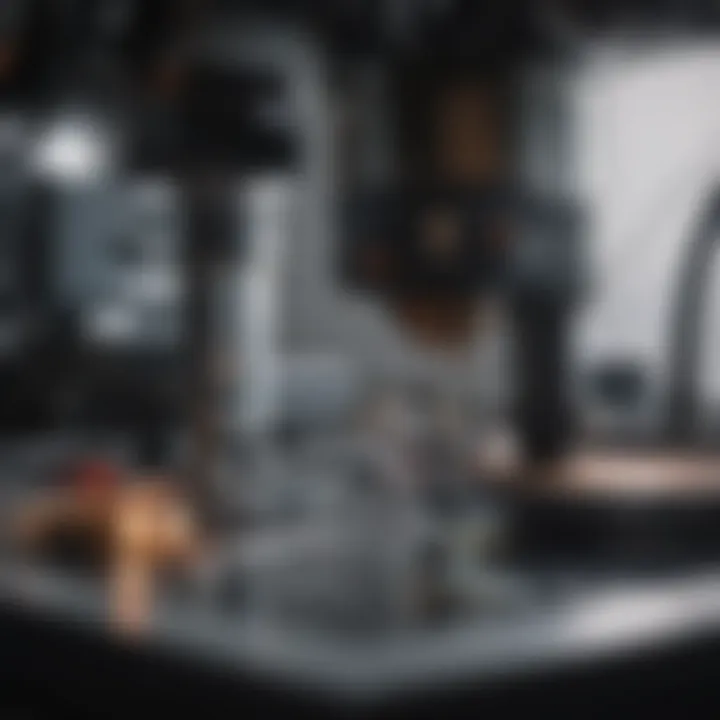
Intro
Fourier Transform Infrared (FTIR) technology has cemented its place as a cornerstone in analytical chemistry. From academic research labs to industrial applications, its role in elucidating molecular characteristics is invaluable. Understanding FTIR involves more than just knowing how it works; it necessitates a grasp of its principles, the breadth of its applications, and the remarkable advancements that continue to evolve the technology.
As we delve into the world of FTIR, it is essential to recognize how this technology bridges gaps in our comprehension of material interactions. Whether employed in identifying unknown substances or monitoring chemical reactions in real-time, FTIR technology is as diverse as it is critical.
Research Overview
Summary of Key Findings
Recent studies underscore the versatility of FTIR, highlighting its application across fields such as materials science, biology, and environmental monitoring. Not only is FTIR pivotal in chemical analysis, but it also serves as a non-destructive technique, allowing researchers to explore samples without altering their structure. Key findings in these areas include:
- Enhanced Sensitivity: Advanced FTIR spectrometers are now capable of detecting minute quantities of analytes, which broadens the scope of potential applications.
- Integration with Other Techniques: There is a growing trend to combine FTIR with other methodologies like mass spectrometry or Raman spectroscopy for a more robust analytical approach.
- Automation and Miniaturization: Developments in instrumentation allow FTIR to be performed on portable devices, making it accessible for on-site analysis, particularly in field studies.
Importance of the Research
Understanding FTIR technology extends far beyond mere technical know-how. Its implications seep into various scientific realms, allowing for deeper insights into:
- The molecular fingerprinting of substances, which aids in forensic investigations and quality control.
- The real-time observation of chemical reactions, leading to improved product formulations in pharmaceuticals and cosmetics.
- Environmental assessments, where the monitoring of pollutant levels has become critical in sustaining ecological integrity.
"The versatility of FTIR technology reflects its significance in advancing scientific methods across a multitude of domains. It's not just a tool; it’s a facilitator of innovation."
Methodology
In unraveling the complexities of FTIR, it is crucial to outline the methodologies employed by researchers in this field. While FTIR itself is a methodology, its application may vary depending on the study's objectives.
Study Design
Studies involving FTIR typically follow a structured design that includes:
- Defining specific research questions that FTIR can address.
- Selecting appropriate FTIR equipment tailored to experimental requirements.
- Considering sample preparation techniques, which can greatly influence the accuracy of results.
Data Collection Techniques
The data collection process in FTIR studies usually involves:
- Spectral Acquisition: Capturing the infrared spectrum by illuminating a sample with infrared light and measuring the resulting absorbance.
- Baseline Corrections: Adjusting for any background noise to enhance the interpretability of the spectra.
- Data Analysis: Employing software tools to interpret the spectral data, which may include peak identification and quantification methodologies.
Through this structured methodology, researchers can consistently yield valuable data that underpins the scientific advancements made possible via FTIR technology.
By investigating the underlying principles, diverse applications, and cutting-edge advancements in FTIR, we equip ourselves with the tools to navigate its vast potential.
Prologue to FTIR Technology
Understanding FTIR technology is pivotal, given its wide-ranging influence in various scientific fields. This section sets the stage, peeling back layers of complexity to reveal why FTIR is a fundamental asset in analytical chemistry, materials science, and beyond. By grasping the principles behind FTIR, readers can appreciate its significance in research and industry.
Definition and Overview
Fourier Transform Infrared (FTIR) spectroscopy is a technique that leverages the interaction of infrared light with matter to derive molecular information about a sample. Essentially, FTIR allows scientists to detect and analyze the bonds and functional groups present in a substance. When infrared radiation passes through a material, some wavelengths are absorbed while others are transmitted. The resulting spectrum functions as a molecular fingerprint, unique to each compound.
What's really interesting is how FTIR brings an array of benefits. It's rapid, non-destructive, and can analyze solid, liquid, and gaseous samples alike. Additionally, FTIR instrumentation is not prohibitively expensive, making it accessible for both academic institutions and industrial laboratories. With advancements in technology, FTIR has matured from bulky setups into compact and more accessible platforms, broadening its application across diverse sectors.
Historical Background
The concept of FTIR arose in the 20th century, yet the foundations of infrared spectroscopy have deeper roots, tracing back to early spectral techniques of the 1800s. Initially, spectroscopy was a labor-intensive and time-consuming process. Then came the Fourier Transform, an innovation attributed to mathematician Jean-Baptiste Joseph Fourier in the early 1800s. His work provided a means to decompose complex signals into simpler components, laying crucial groundwork for FTIR technology.
In the 1950s, the first commercial FTIR spectrometers became available, marking a turning point in the field of spectroscopy. This technology allowed researchers to obtain full spectra in mere seconds, a stark contrast to the lengthy processes of earlier methods.
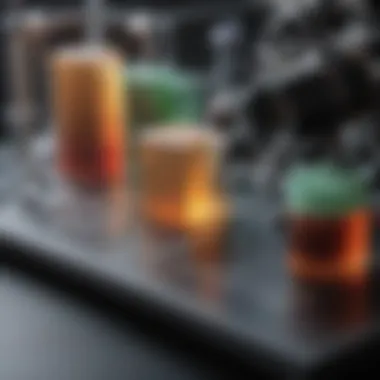
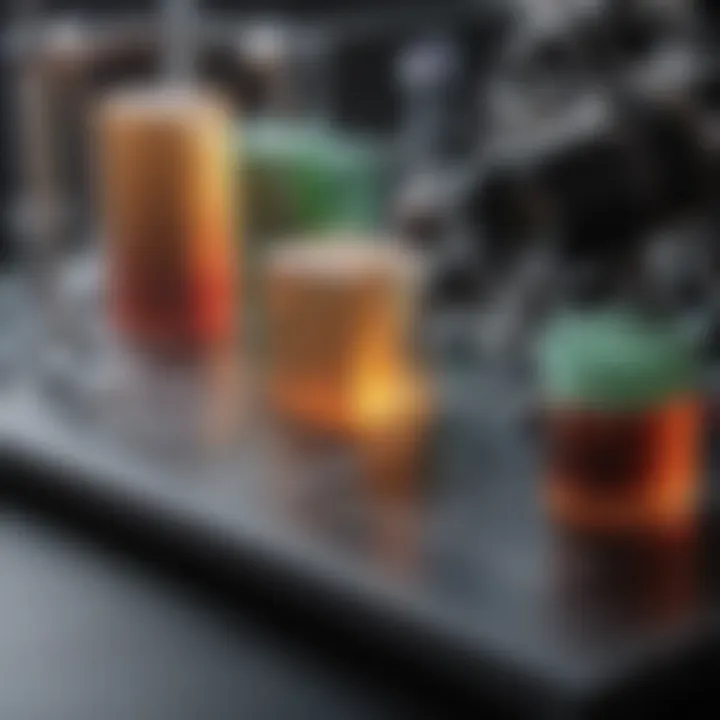
As FTIR evolved, it became recognized for its versatility. Whether in materials science, environmental testing, or pharmaceuticals, FTIR instruments began to dot laboratories worldwide. This transition demonstrated not just the emergence of new technology but also the growing realization of optics and signal processing's roles in understanding molecular structures. With continuous advancements, FTIR has transformed from a niche analytical tool to a cornerstone in modern scientific research.
Fundamental Principles of FTIR
The realm of Fourier Transform Infrared (FTIR) technology is intrinsically linked to its fundamental principles. Understanding these principles is pivotal for grasping how FTIR serves as a lens to illuminate the molecular world around us. At its core, FTIR is not just an analytical tool; it’s a bridge connecting theoretical physics with practical applications in chemistry, material science, and beyond. This section meticulously explores the crucial concepts underpinning FTIR technology, establishing a framework that is essential for both students and seasoned professionals alike.
The Infrared Spectrum
The infrared spectrum, which occupies the wavelength range between 0.7 and 1,000 micrometers, is the foundation upon which FTIR operates. It is further divided into three segments: near-infrared, mid-infrared, and far-infrared. Each range corresponds to different molecular vibrations that the technology aims to interpret. The mid-infrared region, in particular, is vital because it contains the most informative absorption bands related to the fundamental vibrations of chemical bonds.
By analyzing how various substances absorb infrared light, FTIR spectrometers provide insight into molecular composition, functional groups, and structural features of compounds. For instance, consider a sample containing organic compounds. The unique pattern of absorbance at specific wavelengths will behave like a fingerprint. This detection allows researchers to ascertain molecular interactions and elucidate complex chemical phenomena.
Fourier Transform Methodology
Fourier Transform is the mathematical backbone of FTIR technology. It transforms the time-domain signal captured during the interference of infrared light into a frequency-domain spectrum. This process is influential in improving the quality and speed of data interpretation.
In practice, Fourier Transform methodology enhances signal-to-noise ratios, which is particularly beneficial when analyzing low-concentration samples. Here’s a simple overview of how it functions:
- Interference Patterns: An interferometer collects data in the time domain.
- Transformation: The captured interferogram is subjected to a mathematical Fourier Transform.
- Frequency Spectrum: This data is converted into a usable frequency spectrum, making it simpler to identify absorption features.
The efficiency and speed gained through this methodology have revolutionized spectroscopic analysis, making FTIR an invaluable tool in both research settings and industry applications.
Spectroscopic Techniques
FTIR encompasses various spectroscopic techniques that cater to specific needs, ensuring versatility in application. One predominant method is Attenuated Total Reflectance (ATR), which is commonly used for solid and liquid samples. ATR allows for easy sample preparation and minimal interaction with the sample itself, reducing potential contamination or alteration.
Additionally, baseline correction and spectral subtraction techniques help refine the obtained data, isolating relevant signals from noise. The combination of these techniques results in a powerful analytical approach that delivers high-quality results, providing clarity in what can often be a noisy dataset.
The pivotal role of spectroscopic techniques in FTIR not only enhances the accuracy of analyses but also broadens the spectrum of feasible applications, from environmental monitoring to pharmaceutical quality control.
Components of FTIR Instruments
Understanding the components of FTIR instruments is like unraveling the mechanism of a well-oiled machine. Each part plays a crucial role, contributing to the overall functionality and efficacy of Fourier Transform Infrared (FTIR) spectroscopy. When discussing FTIR technology, it's imperative to recognize how these components interact and the impact they have on data accuracy and analysis.
Interferometer Design
At the heart of FTIR technology lies the interferometer, specifically the Michelson design. This device is pivotal for measuring the phase differences of light waves, resulting in the interference patterns essential for generating spectra. The design consists of beamsplitters and mirrors, allowing the infrared (IR) light to travel different paths before recombining.
The beamsplitter, typically made from potassium bromide or germanium, divides incoming IR light into two beams. One beam reflects off a stationary mirror while the other reflects off a moving mirror. The movement of the mirror creates an optical path change that is invaluable for spectral data collection. As the moving mirror lengthens or shortens, the interference patterns vary, leading to the distinctive spectral patterns we analyze.
Moreover, the precision of the optical components directly influences the spectral resolution of the analysis. If the beamsplitter isn’t calibrated correctly or if there are misalignments, data can become skewed, leading to ambiguous or misleading results. To sum it up, a well-designed interferometer is fundamental for generating high-quality spectra, and its intricate design choices directly affect performance.
Detector Systems
Next in line is the detector system, which captures the IR light after it passes through the sample. Different detector types serve diverse analytical needs, ranging from the common deuterated triglycine sulfate (DTGS) detectors to the more sensitive mercury cadmium telluride (MCT) detectors. The choice of detector can significantly influence sensitivity and detection limits.
For instance, DTGS detectors are widely used due to their robustness and capacity to function without liquid nitrogen cooling; however, they may lack some sensitivity compared to MCT detectors. In contrast, MCT offers heightened sensitivity but requires cooling for optimal performance. The flexibility of FTIR instruments is partly due to this variety in detector systems, accommodating different project requirements.
The proper functioning of these detectors is also crucial for accurate data interpretation. If a detector has a low signal-to-noise ratio, it becomes difficult to discern relevant data from background noise. Thus, the detector selection has implications not just for performance but also for the viability of concluding results drawn from the spectral data.
Sample Presentation Techniques
The final piece of the puzzle, sample presentation techniques, fundamentally impacts how the FTIR readings are conducted. Presenting samples in the appropriate manner ensures the fidelity of the results. Several methods, including transmission, reflection, and attenuated total reflection (ATR), have distinct pros and cons.
- Transmission technique involves placing thin samples directly in the path of the IR beam. This method works well for solids and liquids, provided the sample is thin enough.
- Reflection is often employed for surfaces or layers that are challenging to analyze via traditional transmission methods. This division transforms how researchers analyze coatings or thin films.
- The attenuated total reflection (ATR) method allows for quick and reliable measurements without extensive sample preparation. This is often favored in scenarios where time and simplicity are critical.
To make things even more intriguing, the choice of technique can result in variations in spectral quality. Sample thickness, uniformity, and even orientation can subtly alter the readings, leading to a critical evaluation of the sample handling process.
In short, the interplay between the interferometer, detector systems, and sample presentation techniques is central to the effectiveness of FTIR instruments. All these components come together to form a sophisticated and nuanced analysis tool that can yield precise and detailed information about a sample's molecular structure. Understanding their operational intricacies ultimately leads to better research outcomes.
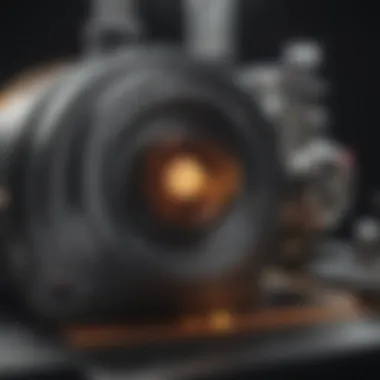
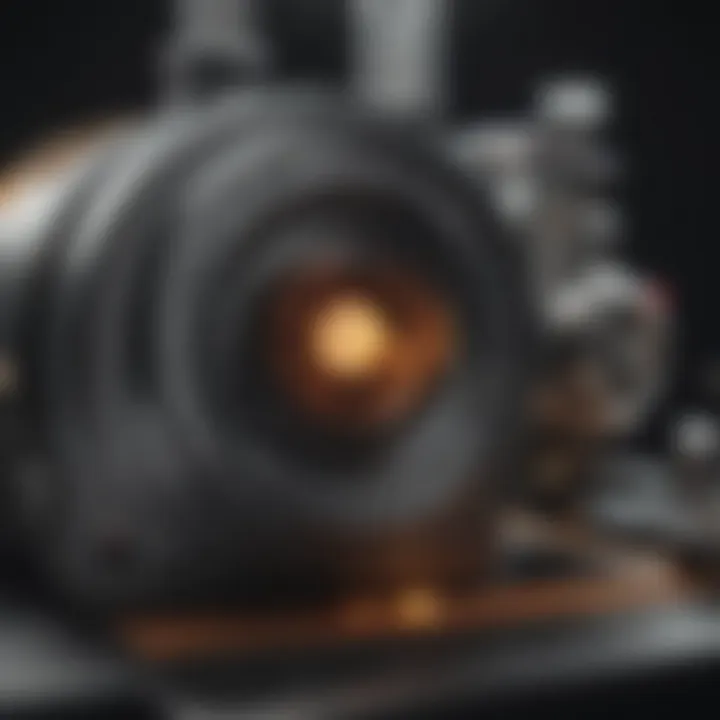
Applications of FTIR Technology
The versatility of FTIR technology is impressive, allowing its applications to span multiple scientific disciplines. Its significance cannot be understated as it provides crucial insights into molecular structure and composition. By applying FTIR, researchers and professionals can achieve accurate analyses in fields ranging from chemistry to food safety. This section covers the primary areas where FTIR shines by dissecting these applications and their respective influences on each field.
Chemistry and Materials Science
In the realm of chemistry and materials science, FTIR acts as a cornerstone for qualitative and quantitative analysis of compounds. Whether it’s identifying functional groups in organic compounds or characterizing polymers, FTIR provides rapid results that are reproducible and reliable. For instance, analysts might employ FTIR spectroscopy to investigate the binding interactions in polymer blends. Elevated precision in characterizing materials leads to innovations in product formulation.
- Sunday Best: FTIR is often used for analyzing molecular changes during polymer processing, helping scientists optimize formulations.
- Beyond the Surface: The technique aids in studying surface coatings, an essential aspect for providing durability and performance in materials.
- Material Realizations: FTIR's ability to monitor reactions in real-time allows for efficient development in nanomaterials and composites.
These capabilities demonstrate that FTIR is more than just a tool—it enriches our comprehension of material interactions at a molecular level.
Pharmaceutical Analysis
Pharmaceutical analysis benefits immensely from FTIR technology. In this sector, ensuring the quality and efficacy of drugs is non-negotiable. FTIR is routinely used in characterizing raw materials, verifying the identity of active pharmaceutical ingredients (APIs), and monitoring changes over time. For pharmaceutical formulators, this means peace of mind when developing new therapies.
- Regulatory Compliance: FTIR can help demonstrate compliance with pharmacopeial standards, making it essential in quality control processes.
- Stability Studies: The ability to identify degradation products ensures that medications meet safety requirements throughout their shelf life.
In this manner, FTIR serves as both a gatekeeper and a facilitator in the journey from formula to final product.
Environmental Studies
Environmental scientists are increasingly harnessing FTIR for monitoring and analyzing pollutants in air, soil, and water. This technology provides an efficient method for detecting organic compounds, particularly volatile organic compounds (VOCs), which can have lasting effects on human health and ecosystems. Given the pressing need for environmental conservation, FTIR plays a pivotal role.
- Air Quality Assessments: Regular FTIR checks can trace hazardous gases in urban environments, providing data critical for public health policies.
- Soil and Water Testing: By identifying contaminants, FTIR aids in remediation strategies, ensuring ecosystems can recover from human impact.
Ultimately, FTIR helps illuminate the hidden elements of our environment, fostering a deeper understanding that informs smarter, more strategic conservation efforts.
Food Safety and Quality Control
In today’s world, consumers demand transparency regarding food quality, emphasizing the importance of safety and compliance. FTIR serves as an essential tool in the food industry by facilitating rapid analysis of ingredients and end products. This application is not just limited to the detection of adulterants but extends to analyzing moisture content, fats, and oils.
- Ingredient Verification: FTIR assists in checking authenticity in products, such as confirming that an olive oil is indeed extra virgin.
- Nutritional Profiling: By analyzing food samples, manufacturers can control the quality and consistency of their products, aligning with regulatory standards.
Through the lens of FTIR, food safety gains a layer of assurance, allowing stakeholders to ensure that what reaches consumers is not only safe but also of the highest quality.
Recent Advances in FTIR Technology
Recent advancements in FTIR technology have spearheaded a transformation across analytical fields, providing researchers with tools that push the boundaries of what is possible in molecular analysis. As we stand on the brink of new discoveries, it's crucial to comprehend how these innovations not only enhance traditional methodologies but also create entirely new avenues for exploration. The enhancements mainly focus on improving accessibility, increasing integration with other analytical processes, and leveraging state-of-the-art data processing techniques. These developments are particularly advantageous for industrial applications, research laboratories, and academic institutions, fostering a deeper understanding of materials and their interaction with the environment.
Miniaturization and Portability
The miniaturization of FTIR instruments marks a significant leap forward. Conventional FTIR setups were often bulky, making them cumbersome for fieldwork or applications in tight spaces. Advances now allow scientists to carry compact versions, such as portable FTIR spectrometers. This mobility provides researchers the flexibility to conduct on-site analyses, whether in environmental monitoring or food safety validation.
Some notable benefits include:
- Enhanced Field Applications: Testing can be done right where samples are collected, minimizing transport time and potential contamination.
- User-Friendly Interfaces: Modern portable devices often incorporate user-friendly designs that enable even non-specialists to utilize FTIR technology effectively.
- Cost Efficiency: Smaller instruments generally require fewer resources to operate and maintain, making them a cost-effective choice for many laboratories.
Integration with Other Techniques
Integrating FTIR with other analytical methods creates a more comprehensive toolkit for researchers. This combination can clarify complex interrelations within samples. For instance, coupling FTIR with techniques like gas chromatography (GC) or liquid chromatography (LC) allows for a deeper molecular characterization that might otherwise be missed.
The advantages of such integrations include:
- Comprehensive Analysis: Multi-faceted approaches can examine samples for a broader range of substances, improving the robustness of the data.
- Improved Sensitivity: Coupling can enhance detection limits, particularly in trace analysis.
- Streamlined Workflows: Researchers can obtain simultaneous data, reducing the time needed for comprehensive analyses.
Machine Learning and Data Processing
Machine learning is emerging as a game-changer in data analysis related to FTIR spectroscopy. The vast amount of data generated from FTIR spectra can be overwhelming. By employing machine learning algorithms, researchers can efficiently interpret complex spectral data with greater accuracy and speed.
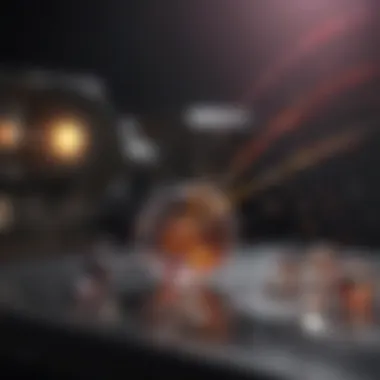
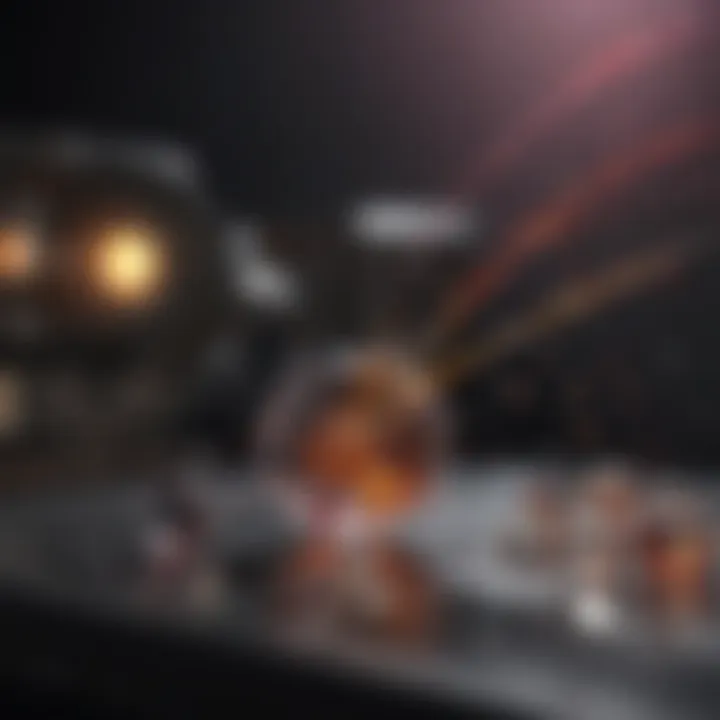
Key benefits include:
- Pattern Recognition: Advanced algorithms can identify subtle spectral patterns that human analysts might overlook.
- Predictive Modeling: Machine learning can predict outcomes based on historical data, making it invaluable for quality control in various industries.
- Automated Workflows: Automation supported by machine learning enhances the efficacy of data processing, freeing up time for researchers to focus on interpretation rather than raw data sifting.
"Integrating machine learning with FTIR technology represents a convergence of traditional science and modern computational power, setting the stage for unprecedented innovations in moleculer analysis."
Challenges and Limitations of FTIR
The utilization of FTIR technology is far from straightforward. While it offers a host of benefits such as speed and precision, it also presents significant challenges that warrant a closer examination. Acknowledging these hurdles is crucial; it allows users to prepare adequately and to mitigate potential complications when utilizing FTIR in various applications. This section will dive into two primary challenges: spectral overlap and sample preparation compatibility. Both aspects can critically affect the reliability of the results obtained from FTIR analysis.
Spectral Overlap and Interpretation
One of the most pressing issues in FTIR spectroscopy is spectral overlap. This phenomenon occurs when different compounds present in a sample yield infrared signals that coincide within the same wavelength range. This can make it particularly tricky to discern the characteristics of individual substances.
For instance, consider a scenario where a sample contains both water and ethanol. The IR spectrum of water has strong absorption bands in the same region where ethanol also absorbs. When plotted together, it becomes nearly impossible to separate the contributions of each compound. Misinterpretation in such cases can lead to skewed conclusions, particularly in sensitive analytical applications like pharmaceutical development or environmental monitoring.
To mitigate this, chemists often employ deconvolution algorithms to analyze overlapping spectra. However, this technique also requires a solid grasp of both the software used and the underlying chemistry of the sample.
Sample Preparation and Compatibility
Another significant consideration is sample preparation. The inherent nature of FTIR demands that samples be prepared in a way that they can be effectively analyzed. Whether it's solid, liquid, or gas, each state presents unique challenges that need addressing. For instance, solid samples often need to be ground into a fine powder or mixed with a non-absorbing matrix to produce a clear spectrum.
Limited compatibility can arise too when samples interact with insulating material used in preparation. A classic case is using potassium bromide pellets for solid samples; certain organic compounds might react with the pellet to produce unwanted peaks, confounding the results.
Moreover, the quality of the sample also plays a substantial role. Impure or poorly prepared samples might yield misleading spectra that do not accurately represent the composition of the analytes in question. Thus, avoiding these pitfalls requires meticulous preparation and sometimes even additional training or proficiency in handling FTIR instruments.
Summary
Future Perspectives in FTIR Research
As Fourier Transform Infrared (FTIR) technology continues to evolve, its potential applications and enhancements pave the way for exciting future developments. The importance of examining these perspectives lies not only in understanding what has been accomplished, but also in envisioning how this analytical tool can further revolutionize various fields of science and industry. The ongoing improvements in FTIR methodologies promise to yield more precise, faster, and broader applications.
Emerging Technologies
Emerging technologies are reshaping the landscape of FTIR research. Some of these innovations focus on enhancing the sensitivity and specificity of FTIR measurements, thereby enabling the detection of trace amounts of compounds that were previously challenging to identify. Here are some notable trends:
- Micro and Nano-FTIR: Advancements in micro-FTIR spectrometers allow for analyses that require minimal sample sizes. These compact devices can analyze microscopic samples, opening new avenues in biomolecular research.
- Optical Coherence Tomography (OCT): This method integrates FTIR with OCT to provide both structural and chemical information in real-time. It serves well in fields such as medical diagnostics and materials science.
- Remote Sensing: The integration of FTIR systems into drones and satellites presents the potential for environmental monitoring on a global scale. This could lead to significant improvements in how we track emissions or assess environmental changes.
"The ability to deploy FTIR technology in diverse and remote locations can vastly enhance our capabilities in research and industry alike."
These emerging technologies not only add versatility to FTIR but also broaden its scope significantly, making it applicable to new and unforeseen challenges.
Applications in Nanotechnology
Nanotechnology is another exciting frontier for FTIR research. The manipulation of matter on an atomic or molecular scale presents unique challenges that FTIR is well-suited to tackle. Here are some areas where FTIR applications are becoming increasingly prominent:
- Characterization of Nanomaterials: FTIR aids in analyzing the chemical composition and structural characteristics of nanomaterials. This information is crucial for researchers who are developing new materials with tailored functions.
- Drug Delivery Systems: With the rise of nanoparticles in drug delivery, FTIR allows scientists to study how drugs interact with these carriers at the molecular level. Understanding these interactions is vital for increasing the efficacy of treatments.
- Surface Plasmon Resonance (SPR): Combining FTIR with SPR can enhance detection capabilities, particularly in the field of biosensors where real-time monitoring of molecular interactions is required.
The application of FTIR technology in nanotechnology not only enhances our understanding of nanoscale materials but also challenges us to find innovative solutions to the complex issues posed within this evolving discipline.
Thus, the future of FTIR technology seems bright, offering a promising path toward addressing contemporary scientific challenges and unlocking potentials that can transform fields of research and application.
The End
The conclusion serves as the linchpin of the article, encapsulating the essence and breadth of Fourier Transform Infrared (FTIR) technology. By reflecting on the key insights discussed and examining the ramifications within various fields of study, it reinforces the significance of this analytical technique.
Summary of Key Insights
- Understanding Molecular Composition: FTIR technology stands out in its ability to provide detailed information about the molecular structure and interactions of substances. By interpreting infrared spectra, one can discern functional groups and chemical bonds, which is crucial in numerous scientific inquiries.
- Diverse Applications: From chemistry to environmental science, FTIR finds its place in a myriad of applications. Scientists utilize FTIR for material identification, pharmaceutical analysis, food safety, and even assessing environmental pollutants. Its versatility ensures that it remains relevant across multiple disciplines.
- Recent Developments: Ongoing advancements in FTIR, such as miniaturization and integration with machine learning processes, exemplify how technology evolves to meet modern needs. These improvements not only enhance measurement precision but also broaden the scope of potential applications.
- Challenges to Overcome: Despite its advantages, FTIR has limitations like spectral overlaps and sample compatibility issues. Understanding these challenges is essential for effective application and interpretation of results.
The Role of FTIR in Advancing Science
FTIR has carved out a vital niche in contemporary scientific research, forever changing the landscape of material analysis. By enabling researchers to gather real-time data on molecular compositions, it empowers innovations and breakthroughs in various sectors.
- In Chemistry: Researchers are continually exploring new compounds, and FTIR acts as a trusted ally in confirming compound identities and purity levels.
- In Medicine: This technology plays a pivotal role in drug formulation and testing, providing data that supports regulatory standards and patient safety.
- In Environmental Monitoring: FTIR is crucial for detecting hazardous substances in air and water, driving efforts to maintain environmental health.