Understanding Gel Electrophoresis: A Comprehensive Guide
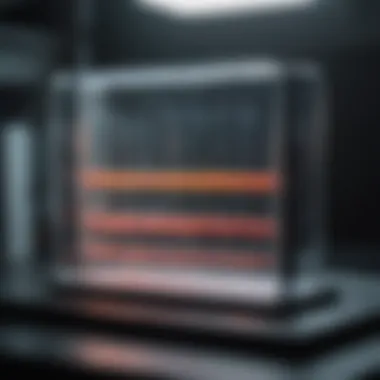
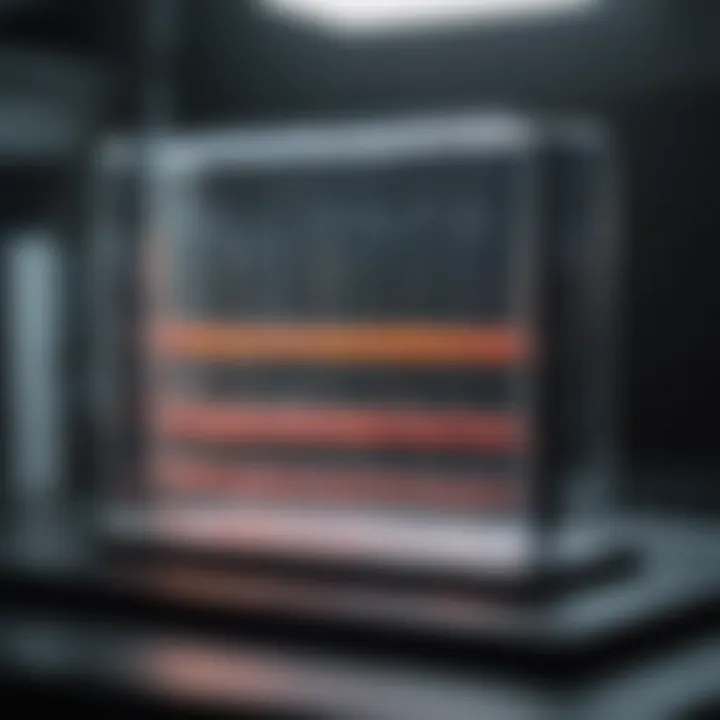
Intro
Gel electrophoresis is a fundamental technique in molecular biology. This method is important for the separation of macromolecules like DNA, RNA, and proteins. Its applications span across various fields such as genetic analysis, forensic science, and biotechnological research. As we explore this process, readers will gain insight into its significance and technical details. The following sections will provide a thorough explanation of how gel electrophoresis works, the types of gels employed, and the interpretation of results.
Research Overview
Summary of Key Findings
Gel electrophoresis allows scientists to separate biomolecules based on size and charge. The process relies on the movement of molecules through a gel matrix under an electric field. Key findings include:
- DNA fragments can be precisely analyzed.
- Protein separation is efficient for various applications.
- The technique has advanced with new technologies improving accuracy and speed.
Importance of the Research
Understanding gel electrophoresis is vital for researchers. It aids in the analysis of genetic material, which is crucial for disease research and diagnostics. Moreover, this technique supports forensic investigations by identifying genetic markers in criminal cases. The continuous advancements in gel electrophoresis technology enhance its role in innovative biotechnological solutions.
"Gel electrophoresis is a cornerstone technique that has transformed molecular biology and other scientific fields."
Methodology
Study Design
The methodological framework for studying gel electrophoresis involves thorough examination of its procedural steps and applications. Each experiment is designed to address specific research questions related to the separation of macromolecules. The design typically includes:
- Selection of the appropriate gel type based on the sample.
- Preparation of the gel matrix, often agarose or polyacrylamide.
- Loading samples into the gel wells.
- Running the gel under an electric field.
- Visualizing the separated bands.
Data Collection Techniques
Data is collected through imaging and quantification of bands after electrophoresis. Techniques include:
- Using UV light for DNA visualization with ethidium bromide.
- Coomassie Brilliant Blue staining for proteins.
- Software analysis for quantitative data.
Each of these techniques is crucial for achieving reliable results and ensuring accurate interpretation of gel electrophoresis outcomes.
Prelims to Gel Electrophoresis
Gel electrophoresis is an essential technique in molecular biology, integral for separating various macromolecules including DNA, RNA, and proteins. This section addresses why understanding gel electrophoresis is critical for researchers, educators, and students alike. The process is not only foundational for genetic analysis and biotechnology but also plays an important role in fields like forensic science and clinical diagnostics. By grasping the intricacies of this method, one can better appreciate its applications and underlying mechanisms.
The significance of gel electrophoresis lies in its versatility and reliability. Additionally, the ability to differentiate molecules based on size and charge enhances its utility in research labs. This technique helps in purifying samples, verifying the results of PCR amplifications, and conducting DNA fingerprinting. The benefits extend beyond practical applications; understanding gel electrophoresis also enriches one's knowledge of molecular biology methodologies.
Furthermore, advances in technology have expanded the capabilities of gel electrophoresis. Automation and integration with other experimental techniques make it a rapidly evolving field, presenting new opportunities for inquiry and discovery. Thus, a comprehensive overview of gel electrophoresis not only facilitates the mastery of a vital scientific skill but also encourages innovative thinking in research contexts.
Definition and Purpose
Gel electrophoresis is defined as a laboratory method that separates charged particles, primarily nucleic acids and proteins, across a gel matrix under an electric field. The primary purpose of this technique is to analyze and visualize these molecules, enabling researchers to determine their size, shape, and quantity. This process can isolate specific molecules from complex mixtures, which is crucial in molecular diagnostics, cloning, and sequencing projects.
The methodology relies on the principle that different molecules migrate at different rates when subjected to an electric current. For example, smaller DNA fragments will travel faster and farther than their larger counterparts. This fundamental behavior forms the crux of separating various macromolecules, providing clarity in analyses.
Historical Context
The development of gel electrophoresis dates back to the mid-20th century, with significant contributions from various scientists. The technique took shape in the early 1950s when scientists began to recognize the potential of electric fields to separate biological molecules. Notably, the work of Arne Tiselius in the 1940s laid critical groundwork for the understanding of electrophoresis as a separation technique.
In subsequent decades, there were key advancements in gel matrix compositions, such as agarose and polyacrylamide, which have become widely used. These materials allow for enhanced resolution and better separation of macromolecules based on size. The historical evolution of gel electrophoresis reflects continuous innovation that responds to the needs of molecular biology research. Now, it remains a staple in laboratories worldwide.
Fundamental Principles
The fundamental principles of gel electrophoresis serve as the backbone of the technique. Understanding these principles is paramount for students, researchers, and professionals working in molecular biology. It provides insights into how macromolecules are separated, the factors affecting their movement through the gel, and the interpretation of results. By grasping these concepts, one can facilitate experimentation and optimize conditions leading to improved efficiency and accuracy.
Electrophoresis Basics
Electrophoresis is the process that allows the separation of charged particles when placed in an electric field. The molecules move in response to the applied electric current, and their rate of migration is influenced by several factors. Critical among these are the size, charge, and shape of the molecules being analyzed. For instance, smaller molecules traverse the gel more quickly than larger ones. The overall effectiveness of electrophoresis depends not only on the characteristics of the samples but also on the properties of the gel itself.
In essence, electrophoresis relies on the interaction of charged molecules with the gel medium. Molecules with a net negative charge will move toward the positive electrode, while positively charged ones migrate to the negative electrode. Understanding this basic principle is essential for anyone seeking to manipulate electrophoretic techniques with precision.
Role of Electric Field
The electric field exerts a critical force on the charged molecules during electrophoresis. The strength of the electric field, typically measured in volts per centimeter (V/cm), determines the speed at which molecules migrate through the gel. A higher voltage can increase the separation rate, but it may also introduce issues such as overheating and distortion of bands. Therefore, optimizing the voltage is key for achieving reproducible results.
Additionally, the direction of the electric field must be considered. A consistent orientation allows for accurate tracking of molecular migration. Researchers should be careful to not exceed voltage limits defined for their gel type, as doing so might compromise separation integrity and resolution.
Interaction with Gel Matrix
The gel matrix, commonly made of agarose or polyacrylamide, plays a vital role in the electrophoresis process. The matrix provides a porous environment through which molecules travel. Different gel compositions and concentrations can affect the pore size and, consequently, the separation of molecules based on size and charge.
When a sample is loaded onto a gel, the molecules encounter resistance from the gel. This resistance is not uniform; smaller molecules navigate more freely through the pores than larger molecules, which may become impeded. Understanding how the gel matrix impacts movement is important for selecting the appropriate gel for specific applications.
Overall, the interaction between the gel matrix and the molecules being studied directly influences the resolution and clarity of the resulting bands. Proper selection of gel type and concentration based on the sample characteristics is mandatory for optimal results.
Understanding the fundamental principles of gel electrophoresis is foundational for effectively applying this technique in various scientific investigations.
Materials Required
The successful execution of gel electrophoresis hinges on the materials utilized in the process. Understanding these materials is essential for optimizing results and ensuring effectiveness in separating biomolecules. Each component plays a significant role in achieving precise outcomes during analysis.
Gel Composition
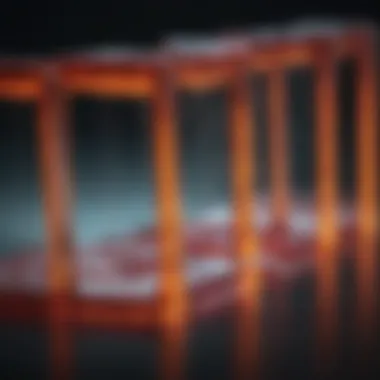
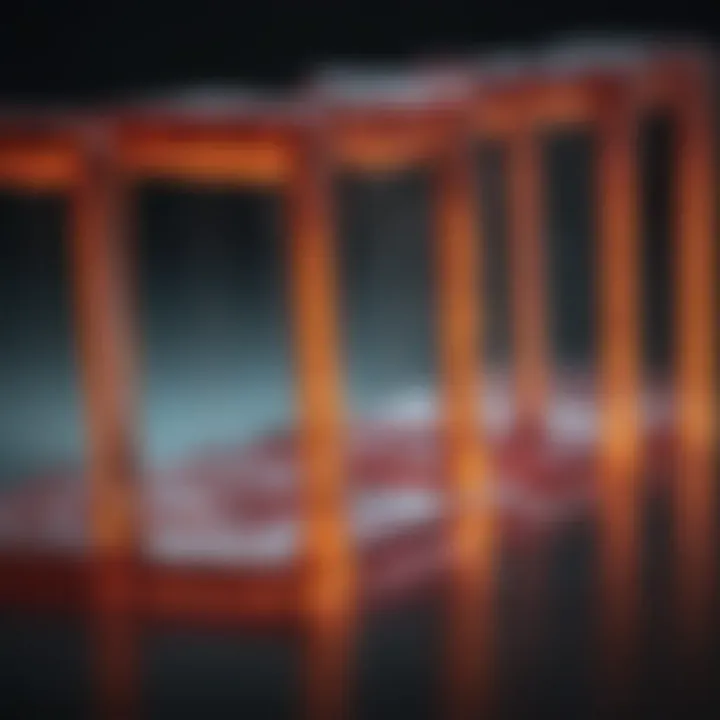
Agarose Gels
Agarose gels are widely recognized for their versatility in molecular biology applications. The primary component of these gels is agarose, a polysaccharide extracted from seaweed. This gel type is preferred for separating larger DNA fragments due to its porous nature, which allows for easy passage of larger molecules while providing adequate resolution. The key characteristic of agarose gels is their ability to create a uniform matrix, ensuring consistent results across samples. This quality makes them a dependable choice for routine gel electrophoresis experiments.
The unique feature that distinguishes agarose from other gels is its ease of preparation and the ability to alter the concentration based on the size of the target molecules. Higher concentrations yield a denser gel suitable for separating smaller fragments, whereas lower concentrations are ideal for larger pieces. One disadvantage, however, is that agarose gels typically have a lower resolution for smaller DNA fragments compared to others, like polyacrylamide gels.
Polyacrylamide Gels
Polyacrylamide gels serve as another critical component in gel electrophoresis, especially for separating proteins. These gels are formed through the polymerization of acrylamide, creating a mesh-like structure. Their significant advantage is the ability to finely tune pore size by adjusting acrylamide concentration, making them suitable for resolving small biomolecules effectively. The key characteristic of polyacrylamide gels is their superior resolution compared to agarose, which is particularly evident in applications such as SDS-PAGE for protein analysis.
A unique feature of polyacrylamide gels is their applicability in both denaturing and non-denaturing conditions. This versatility allows researchers to explore various interactions and characteristics of proteins. However, polyacrylamide gels require more careful handling and preparation due to potential health risks associated with acrylamide, a neurotoxin.
Buffer Solutions
Buffer solutions are pivotal in maintaining the pH and ion concentration during gel electrophoresis. These solutions provide the necessary ionic environment, crucial for effective separation and migration of molecules within the gel matrix.
Common Buffers
The choice of common buffer solutions, such as Tris-Glycine or TAE (Tris-Acetate-EDTA), influences the overall effectiveness of the electrophoresis. These buffers maintain stable pH levels during the process and serve to carry ions throughout the gel. The key characteristic of common buffers is their ability to affect the conductivity of the gel and facilitate molecule movement. They are favored for their compatibility with a broad range of applications in molecular biology.
A unique feature of these buffers is their ability to prevent excessive heat buildup during electrophoresis, which can affect results. However, one disadvantage is that some buffers may react with the samples, potentially leading to artifacts during analysis.
pH Considerations
The pH of the buffer is a critical factor that requires careful attention. It affects the charge of the molecules, which in turn influences their migration during electrophoresis. The key characteristic of pH considerations is their impact on the ionic strength of the buffer. Maintaining an optimal pH ensures consistency and precision in results. This makes pH considerations crucial for accurate analysis of biomolecules.
Another aspect to highlight is that different molecules require specific pH conditions for optimal separation. For instance, proteins may need varied pH levels depending on their isoelectric points. A disadvantage is that maintaining the correct pH can be challenging if not monitored closely, resulting in altered outcomes.
Loading Dye and Sample Preparation
Loading dye assists in the visualization of samples as they are loaded into the gel. It contains a tracking dye, typically bromophenol blue, which helps to monitor the progress of electrophoresis. Proper sample preparation is also essential. Sample concentration, volume, and the presence of contaminants can affect the resolution and clarity of the bands.
In summary, the materials required for gel electrophoresis are fundamental in achieving reliable and reproducible results. Each component, from gel type to buffer choice, contributes to the efficiency of this important technique.
The Gel Electrophoresis Process
The gel electrophoresis process is crucial for understanding how macromolecules, such as DNA, RNA, and proteins, are separated and analyzed in a laboratory setting. This technique forms the backbone of many molecular biology experiments and provides invaluable insight into various applications, such as genetic analysis and forensic science. By following the steps of this process, researchers can obtain specific results which can lead to significant conclusions in their studies. Each step of gel electrophoresis must be executed with precision, as their cumulative effects determine the quality and accuracy of the results obtained.
Preparation of Gel
Preparing the gel is one of the foremost tasks in gel electrophoresis. The gel serves as a matrix that allows molecules to move when an electric field is applied. The most common types of gels used are agarose and polyacrylamide, each chosen based on the size of the molecules being studied. The concentration of the gel can be adjusted to control the size of pores, affecting the separation of macromolecules. For example, lower concentrations of agarose are typically used for larger DNA fragments, while higher concentrations are appropriate for smaller pieces.
The preparation of the gel involves dissolving the chosen powder in a buffer solution and heating it until it forms a homogeneous mixture. This mixture is then poured into a casting tray, allowing it to cool and solidify. Care must be taken during this step to minimize bubbles to ensure even flow of samples during electrophoresis.
Sample Loading
Once the gel is prepared, the next step is sample loading. This is a vital stage where the researcher carefully adds the samples mixed with loading dye into the wells created in the gel. The loading dye provides both color and density, allowing for easy visualization during the loading process. It is important to ensure that the samples do not overflow and mix in the wells as it may cause cross-contamination. Precision and care during this step are critical because any error can compromise the entire experiment.
Running the Electrophoresis
After the samples are loaded, the gel is placed into an electrophoresis chamber. An electric current is applied, and the negatively charged macromolecules move towards the positively charged electrode. Factors such as the gel composition, voltage, and buffer system directly affect how quickly molecules migrate. Generally, smaller molecules traverse the gel more quickly than larger ones, leading to separation. This separation allows the researcher to analyze the banding patterns that form as the molecules migrate through the gel matrix.
Staining and Visualization
After electrophoresis is complete, staining the gel is critical for visualization of the migrated bands. Stains bind to nucleic acids or proteins, making them observable under UV light or other imaging systems.
Ethidium Bromide
Ethidium bromide is a commonly used stain due to its ability to intercalate between DNA bases. When exposed to UV light, it fluoresces, allowing researchers to see the DNA bands clearly. This property makes it a beneficial choice for many laboratories. However, ethidium bromide is a potent mutagen, requiring careful handling and disposal practices. Its advantages include high sensitivity and ease of use, but the safety concerns mean that alternatives are sometimes preferable for specific applications.
Coomassie Blue
Coomassie Blue is another staining option, primarily used for proteins. This stain binds to proteins in a gel to produce visible blue bands. It is particularly useful for polyacrylamide gels. Coomassie provides relatively high sensitivity and can stain proteins in non-denaturing conditions, preserving their structure. Despite its advantages, it is less sensitive compared to ethidium bromide for nucleic acids, which limits its application in pure DNA analysis. Nonetheless, it remains a popular choice in protein studies due to its robust performance across a variety of sample conditions.
Types of Gel Electrophoresis
The various types of gel electrophoresis play a crucial role in molecular biology. They provide different approaches for separating macromolecules based on specific characteristics. Understanding each type allows researchers to select the most appropriate method for their particular needs. The distinction between agarose and polyacrylamide gel techniques, along with advances like capillary electrophoresis, shapes how scientists approach analysis and interpretation of biological samples.
Agarose Gel Electrophoresis
Applications
Agarose gel electrophoresis is widely used for the separation of nucleic acids. This method is simple and effective, making it a common choice for DNA and RNA analysis. The primary advantage of agarose gels lies in their ability to resolve large fragments of DNA. This is essential for tasks such as restriction fragment length polymorphism (RFLP) analysis and DNA fingerprinting.
A key characteristic of agarose gel applications is the range of concentrations used. Gels can be prepared with varying percentages of agarose, which influences the pore sizes. This flexibility allows for the separation of a broad spectrum of sizes while ensuring resolution. However, the technique does not perform as well with smaller fragments, which can limit its use.
Advantages and Limitations
Agarose gel electrophoresis offers some significant advantages. It is relatively easy to prepare and requires minimal equipment. Furthermore, it is considered safe when compared to some of the reagents used in other techniques. This method also allows for quantification by using standard samples.
However, there are limitations. One notable limitation is the resolution capability for smaller nucleic acid fragments. For fragments smaller than 100 base pairs, other methods like polyacrylamide gels may be necessay. Additionally, the gels can be more fragile, requiring careful handling.
Polyacrylamide Gel Electrophoresis
Types: SDS-PAGE, Native PAGE
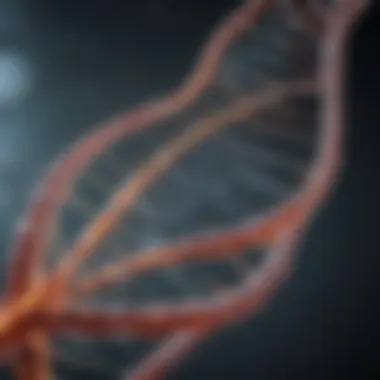
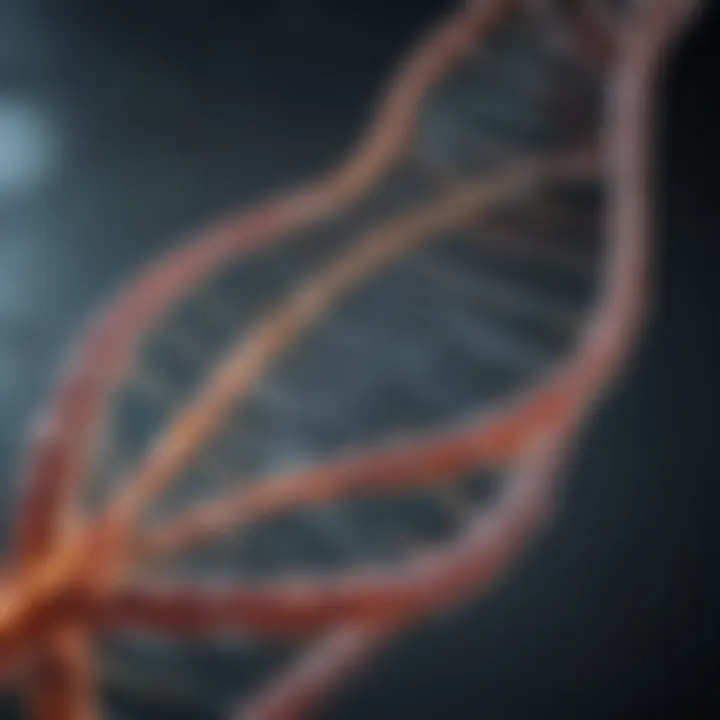
Polyacrylamide gel electrophoresis (PAGE) is another fundamental technique used in protein analysis. The two main types include SDS-PAGE and native PAGE. SDS-PAGE is specifically used for denaturing proteins, which separates them based on their molecular weight. It is widely applied in protein characterization and quantification.
On the other hand, native PAGE maintains the proteins in their natural state. This allows for the assessment of the protein's natural charge, conformation, and size. The versatility of these two types provides researchers with essential tools for detailed insights into protein functionality and interactions.
Specific Uses
Polyacrylamide gel electrophoresis is especially pertinent in analyzing complex protein mixtures. The ability to separate proteins with minimal sample preparation enhances its value in biochemical research. Specific applications include enzyme assays, determining protein-protein interactions, and post-translational modifications analysis.
A significant factor that characterizes the use of PAGE is its precision. Resolution is much higher than that of agarose gels, especially for small proteins. This capability makes it a vital technique in both research and clinical labs.
Capillary Electrophoresis
Capillary electrophoresis is an advanced method that allows for high-resolution separation of various biomolecules. It utilizes thin capillaries filled with a gel or polymer to facilitate the movement of analytes under the influence of an electric field. The major advantage of this method is its ability to provide extremely fast analysis times while requiring minimal sample volumes.
Capillary electrophoresis can separate not only nucleic acids but also proteins and peptides. This adaptability makes it an attractive option for comprehensive analysis in the fields of biotechnology and diagnostics.
In summary, understanding the various types of gel electrophoresis helps refine experimental conditions and improve the quality of results. Each method has its unique applications, benefits, and limitations, making them suitable for different scenarios in research.
Data Analysis and Interpretation
Data analysis and interpretation are crucial components of gel electrophoresis. The results acquired from this technique need thoughtful scrutiny to derive meaningful conclusions. An accurate analysis helps in the identification and characterization of biomolecules. This section delves into three key elements: band patterns, standardization, and quantitative analysis.
Band Patterns and Sizes
The visualization of band patterns on a gel serves as an essential foundation for analysis. Each band represents distinct molecules separated based on size and charge during electrophoresis. To determine the size of these molecules, a standard DNA ladder or protein marker is often used for reference. The migration distance of the bands correlates with molecular weight, providing valuable information regarding sample composition.
An important element is understanding how various factors influence band resolution. For example, choosing the right concentration of agarose or polyacrylamide gel affects the separation quality. It’s also imperative to consider loading volumes and sample purity to avoid overlapping bands, which may complicate the analysis. Accurate interpretation of band sizes aids in identifying molecular entities, understanding genetic variations, and assessing protein efficacy.
Standardization and Controls
Standardization in gel electrophoresis ensures reproducibility and reliability of results. Implementing controls is crucial for validating the process. Positive controls, negative controls, and technical replicates help in understanding the reaction dynamics and overall system performance. For instance, including known samples alongside test samples establishes a benchmark, allowing researchers to assess the effectiveness of their experimentation.
Furthermore, consistency in gel preparation and running conditions should be emphasized. Variables such as voltage, buffer composition, and temperature must remain stable to minimize deviations in results. The use of universal standards for specific applications boosts confidence in the data obtained, making conclusions more robust and generalizable.
Quantitative Analysis
Quantitative analysis in gel electrophoresis involves measuring the intensity of bands, which correlates with the concentration of the target molecules. This aspect is significant for various applications, including molecular diagnostics and gene expression studies. Tools like densitometry software facilitate precise measurements of band intensities, supporting the determination of relative abundance.
However, it is crucial to analyze this data critically. One must consider potential errors, such as variations in staining intensity and background noise. Proper normalization using controls can compensate for these discrepancies, enhancing the validity of results. Quantitative results contribute to a deeper understanding of biological processes, enabling scientists to draw concrete conclusions and design further experiments.
"The rigor of data analysis transforms raw electrophoresis results into actionable insights."
In summary, synthesizing the information about band's patterns, standardization practices, and quantitative methodology provides a holistic view of the data analysis in gel electrophoresis. Such diligence is essential for achieving reliable and interpretable findings in molecular biology.
Applications of Gel Electrophoresis
The applications of gel electrophoresis have significantly advanced the fields of molecular biology, clinical diagnostics, and forensic science. This technique allows for the effective separation of various macromolecules, including DNA, RNA, and proteins, which is essential for understanding biological processes. Each application serves a unique purpose and comes with distinct benefits and considerations.
Molecular Biology Research
DNA Fingerprinting
DNA fingerprinting is a powerful method used to identify individuals based on their unique genetic makeup. This application of gel electrophoresis is particularly valuable in criminal investigations, paternity disputes, and biodiversity studies. The key characteristic of DNA fingerprinting is its ability to compare specific regions of DNA, known as STRs, which vary from person to person. This makes DNA fingerprinting a beneficial choice in areas requiring identification and genetic profiling.
One unique feature of DNA fingerprinting is its precision. By analyzing the number of repeats in specific DNA sequences, researchers can generate a unique profile for each individual. However, there are disadvantages. The process can be time-consuming and requires a comparison to a database for accuracy. Moreover, ethical considerations around privacy and consent are present.
Gene Sequencing
Gene sequencing involves determining the exact sequence of nucleotides in a DNA molecule. This application plays a crucial role in genomics, enabling scientists to understand genetic variations and their implications in diseases. The key characteristic of gene sequencing is its ability to provide comprehensive data about genetic composition, which is essential for research and therapeutic purposes.
A unique feature of gene sequencing is its capability to uncover mutations that may lead to genetic disorders, helping in developing targeted treatments. However, the technique requires sophisticated equipment and can be expensive. Additionally, interpreting vast amounts of data generated poses a significant challenge, making it a complex but invaluable tool.
Clinical Diagnostics
In clinical settings, gel electrophoresis is extensively used for diagnosing genetic diseases and analyzing proteins. This application is invaluable for identifying specific genetic markers associated with various conditions, thus aiding in personalized medicine. Gel electrophoresis can separate proteins in blood samples, helping clinicians to detect abnormalities in protein levels that may indicate diseases such as diabetes or kidney dysfunction.
One of the benefits of using gel electrophoresis in diagnostics is the ability to provide rapid results. Hospitals can utilize this technique for routine screening and diagnostic purposes. However, traditional gel methods may not always provide the sensitivity required for detecting low-abundance proteins.
Forensic Science
Gel electrophoresis plays a crucial role in forensic science, particularly in analyzing biological samples from crime scenes. This application allows forensic analysts to profile suspects based on DNA evidence left at the scene of a crime. By comparing the DNA profiles generated through gel electrophoresis, law enforcement can narrow down suspects and link individuals to specific cases.
The significant advantage of using gel electrophoresis in forensics is its reliability in generating conclusive evidence. It operates by separating DNA fragments for comparison, thus offering clarity in investigations. However, forensic applications must adhere to strict protocols to avoid contamination and ensure results are legally defensible.
The integration of gel electrophoresis in various fields not only highlights its versatility but also its critical role in advancing our understanding of genetics and biology.
Advancements in Gel Electrophoresis Technology
Gel electrophoresis technology has continually evolved, leading to enhanced efficiency and accuracy in the analysis of biomolecules. Keeping up with these advancements is crucial for both researchers and practitioners in molecular biology. Innovations in this field not only streamline processes but also improve the resolution and reproducibility of results. The integration of automation, miniaturization, and complementary techniques helps researchers achieve more sophisticated analyses. This section navigates through the most significant advancements in gel electrophoresis technology, highlighting their benefits while considering relevant practical aspects.
Automation of Processes
Automation in gel electrophoresis has significantly improved throughput in laboratories. Automated systems can perform multiple simultaneous experiments, reducing manual labor and minimizing human error. One of the primary advantages of automation is consistency. Automated systems produce uniform results, making it easier to draw reliable conclusions from experiments.
Moreover, these systems can optimize running times and running conditions, thereby increasing the efficiency of separation. For instance, using automated gel preparation units ensures that every gel is prepared under the same conditions, enhancing reproducibility across experiments. The reliability gained through automation is vital for routine testing in clinical and research settings.
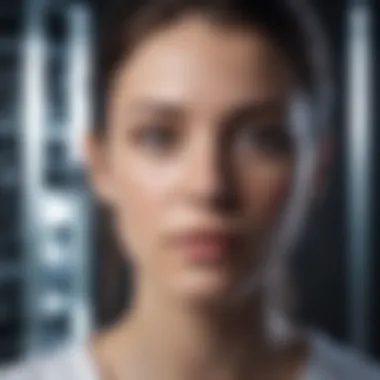
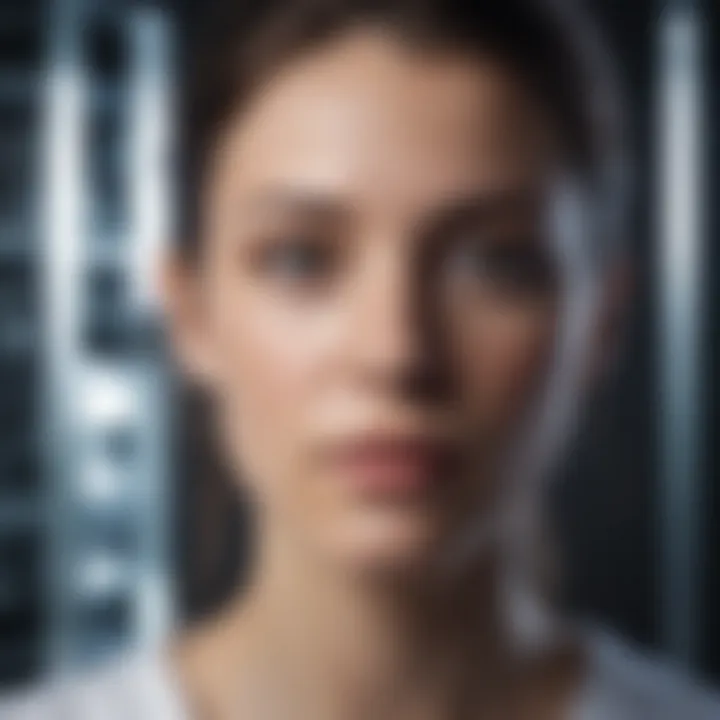
Miniaturization Techniques
Miniaturization in gel electrophoresis aims to reduce the volume of reagents and samples required for experiments. This trend has advantages that include cost savings and reduced waste. Smaller gel formats allow for using less material without sacrificing the quality of results. This method is particularly beneficial for labs with limited resources.
Additionally, miniaturization leads to faster runs, as shorter distances reduce migration time for the molecules. Devices like microfluidic systems represent a significant advancement in this area. They allow precise control over sample handling and separation, creating opportunities for high-throughput screening applications.
Integration with Other Techniques
The integration of gel electrophoresis with other advanced techniques has opened new avenues for biomolecular analysis. This synergy enhances both the analytical depth and efficiency of research endeavors.
Next-Generation Sequencing
Next-generation sequencing (NGS) is a groundbreaking approach that revolutionizes the field of genomics. It allows for the rapid sequencing of large segments of DNA. In conjunction with gel electrophoresis, NGS provides a powerful means of validating results obtained from electrophoretic analyses. The key characteristic of NGS is its ability to generate massive amounts of data in a short time, making it a cornerstone in genomic studies.
A unique feature of NGS is its scalability, as it can be applied to any size of sequencing project, from small targeted gene panels to entire genomes. The advantages of this integration are clear; researchers can achieve greater throughput and more comprehensive insights into genetic information. However, the complexity of data analysis presented by NGS can be a disadvantage, requiring advanced software and statistical expertise to interpret results effectively.
Mass Spectrometry
Mass spectrometry (MS) is another complementary technique that has gained prominence in molecular biology. It is particularly valued for its high sensitivity and ability to analyze complex mixtures accurately. When gel electrophoresis is combined with mass spectrometry, it allows for the clear identification and characterization of proteins and nucleic acids.
The key characteristic of mass spectrometry is its precision in measuring the mass-to-charge ratio of ions, enabling detailed structural analysis. This feature makes it an invaluable tool for confirming the identity of biomolecules separated by gel electrophoresis. Despite its benefits, mass spectrometry has limitations, such as the requirement for extensive sample preparation and the need for costly equipment.
Integrating gel electrophoresis with techniques like NGS and mass spectrometry enables a more comprehensive analysis of biomolecules. These advancements illustrate how collaborative approaches enhance the accuracy and depth of molecular investigations.
As gel electrophoresis continues to advance, it intertwines with developing technologies. Understanding these advancements ensures that professionals remain at the cutting edge of molecular biology research.
Challenges and Considerations
Understanding the challenges and considerations associated with gel electrophoresis is crucial for both practitioners and learners of molecular biology. This section explores the technical limitations that researchers may face and highlights the safety concerns that are vital to ensuring safe laboratory practices. Recognizing these elements contributes to better experimental planning and enhances the overall effectiveness of gel electrophoresis as a technique.
Technical Limitations
Gel electrophoresis, while a powerful method for molecular separation, comes with its share of technical limitations. Understanding these constraints is essential for accurate data interpretation and improved experimental designs.
- Resolution Issues: Different gel types yield varied resolutions. For example, agarose gels may struggle with very small DNA fragments, while polyacrylamide gels excel in resolving such samples.
- Sample Size Restrictions: Large samples can distort bands, leading to inaccurate results. Adhering to appropriate loading volumes is important to avoid compromising the integrity of electrophoresis.
- Staining Sensitivity: The choice of staining dye can affect the visibility and reliability of the results. Certain dyes may not bind to specific types of nucleic acids or proteins effectively, which can result in under-representation in the final output.
- Gel Preparation Variability: Differences in gel preparation, such as inconsistencies in concentration or polymerization, can lead to significant variations in outcomes. This inconsistency may affect reproducibility across experiments.
An awareness of these limitations allows researchers to select the most appropriate techniques and materials for their specific experimental conditions, thereby enhancing the quality of the results.
Safety Concerns
Safety considerations are of utmost importance in the context of gel electrophoresis. Many aspects of this process involve hazardous materials and procedures that require careful management.
- Chemical Hazards: Chemicals used in the preparation of gels and buffers, such as acrylamide and ethidium bromide, pose significant health risks. Ethidium bromide, for instance, is a mutagen and should be handled with care to avoid contamination.
- Electrical Hazards: The presence of electrical equipment raises concerns related to electrical safety. Equipment should be properly maintained, and safety gear should be worn to minimize risk during experiments.
- Disposal Protocols: Proper disposal of chemicals and gels is crucial. Many of these materials can be toxic to the environment, thus requiring adherence to specific disposal guidelines.
- Personal Protective Equipment (PPE): Laboratory workers must always wear appropriate PPE, including gloves, lab coats, and goggles, to mitigate exposure to hazardous substances.
Adhering to established safety protocols and being aware of potential hazards helps ensure a secure laboratory environment, promoting effective research while protecting personnel.
An informed approach to challenges and considerations in gel electrophoresis ensures accurate results and safety in the laboratory.
Understanding and addressing these challenges will not only optimize gel electrophoresis experiments but also foster a culture of safety and efficacy in laboratory settings.
Future Directions in Gel Electrophoresis
The landscape of gel electrophoresis is evolving, propelled by advancements in technology and ongoing research. Understanding these future directions is crucial for students, researchers, and professionals who depend on this technique. The continued refinement of gel electrophoresis can lead to enhanced efficiency and higher accuracy in molecular biology applications.
Emerging Technologies
Recent innovations are transforming the capabilities of gel electrophoresis. Technologies such as microfluidics are gaining traction. These approaches allow for miniaturization of processes, which can reduce sample volumes and increase throughput. Key examples include:
- Lab-on-a-chip systems that integrate multiple steps of molecular analysis into a single device.
- Next-generation sequencing and mass spectrometry integrated with gel electrophoresis can provide comprehensive data about DNA, RNA, and proteins, improving overall analysis accuracy.
Furthermore, improved imaging techniques enhance visualization of the separated molecules in real-time. Automated systems are also emerging, facilitating the process and reducing the risk of human error.
Potential Enhancements and Applications
As gel electrophoresis continues to evolve, several potential enhancements and applications are noteworthy:
- Increased Resolution: Future gel formulations may offer better resolution for complex samples, which is essential for detailed analysis in genomics and proteomics.
- Hybrid Methods: Developing hybrid techniques that combine traditional electrophoresis with new technologies could yield superior methodologies for separation.
- Environmental Sustainability: Advances that promote the use of biodegradable gels could lead to environmentally friendly practices in laboratories.
- Broadening Applications: The integration of gel electrophoresis with techniques used in diagnostics or therapeutic applications could enhance its role in clinical settings. The ability to analyze biomolecules rapidly could be vital in personalized medicine.
"The future of gel electrophoresis not only relies on technological advances but also on the creative application of these methods in varied scientific fields."
In summary, the future directions in gel electrophoresis underscore the importance of innovation in achieving greater precision and utility. As researchers experiment with new methodologies and technologies, the relevance of gel electrophoresis in scientific inquiry is likely to expand significantly.
Ending
The conclusion serves as the final synthesis of the major themes explored in this article. It provides a space to reflect on the importance of gel electrophoresis, particularly its critical role in molecular biology. This method, as detailed, is fundamental for the separation and analysis of various macromolecules. Understanding this process equips students, educators, researchers, and professionals with the knowledge to utilize gel electrophoresis effectively in their respective fields.
Summary of Key Points
In summarizing the key points, it is essential to revisit the pivotal aspects discussed:
- Gel electrophoresis is not just a laboratory technique; it is a cornerstone of molecular analysis.
- The principles behind the process highlight the interaction of molecules within a gel matrix under an electric field.
- Various types of gels, such as agarose and polyacrylamide, serve specific purposes depending on the macromolecules being analyzed.
- Applications extend across molecular biology, forensic science, and clinical diagnostics, demonstrating its broad relevance.
This comprehensive overview elucidates the significance of gel electrophoresis, emphasizing both theoretical concepts and practical implications.
Final Thoughts
As a concluding note, it is important to consider the future of gel electrophoresis. Advances in technology are continually augmenting its efficiency. Automation, miniaturization, and integration with other analytical techniques are transforming how this method is applied in research and diagnostics.
"The future of gel electrophoresis lies in its ability to adapt and innovate alongside advancements in technology and research methodologies."
This concluding section underscores not only the relevance of gel electrophoresis today but also its potential for future applications, providing a solid foundation for further exploration and understanding.