Understanding the Role of gRNA in Genetic Engineering
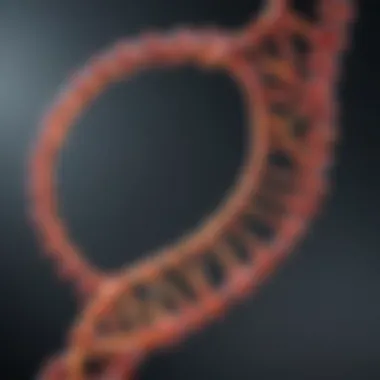
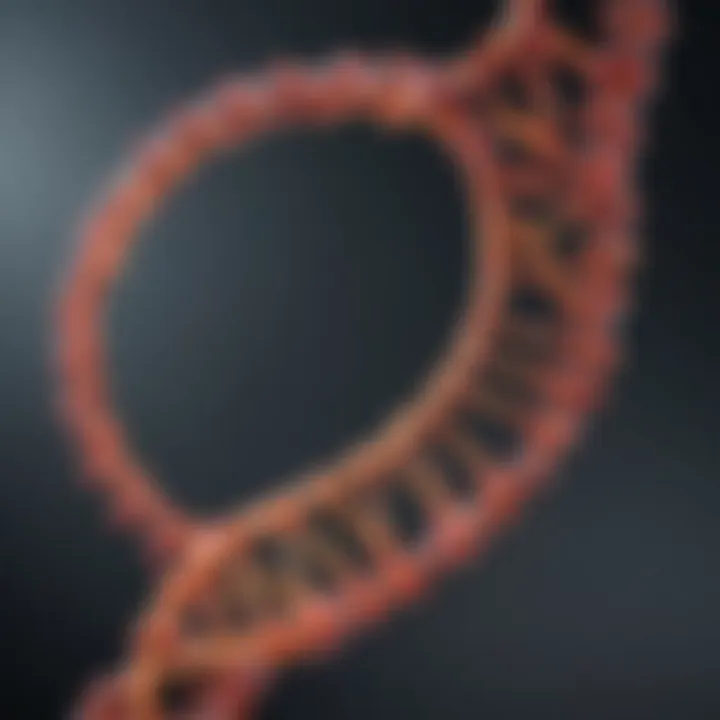
Intro
Guide RNA (gRNA) represents a pivotal component within the landscape of genetic engineering, particularly when discussing the functional capabilities of CRISPR technology. As researchers and biotechnologists continue to explore the intricacies of gene editing, understanding gRNA's role becomes crucial. It is the guiding force that directs Cas proteins to specific genomic locations, thereby facilitating accurate and targeted edits within the DNA sequence.
The relevance of gRNA extends beyond mere mechanics; it intersects with various applications in research and therapeutics. With the rise of CRISPR systems, researchers now possess tools that not only enhance our understanding of genomic structures but also unlock potential advancements in medicine, agriculture, and beyond. This article delves deeply into the foundation, applications, and ethical implications of gRNA, offering insights that aim to empower students, educators, and professionals alike in grasping the transformative potential of gRNA in the realm of genetic engineering.
Prelude to gRNA
The topic of guide RNA (gRNA) is of paramount importance in the field of genetic engineering. It serves as a foundational component of the CRISPR technology, which has revolutionized how scientists approach gene editing. gRNA functions by directing the Cas proteins to specific DNA sequences, enabling targeted modifications within an organism's genome. Understanding gRNA is crucial for grasping the implications of genetic editing, its applications in various sectors, and the ethical questions it raises.
Defining Guide RNA
gRNA can be defined as a short synthetic RNA molecule that plays a significant role in the CRISPR-Cas9 system. It is specifically designed to complement a target DNA sequence, ensuring precision during editing. gRNA consists of two key parts: a sequence that is complementary to the target DNA and a scaffold region that binds to the Cas protein. This structure allows the gRNA to guide the protein to the precise location within a genome where changes are desired.
The advent of gRNA has made the editing process not only more efficient but also more accessible. With advancements in design techniques, researchers can craft gRNAs tailored to specific sequences, enhancing the accuracy and efficiency of genome editing efforts.
Historical Context of Gene Editing
The roots of gene editing extend back to the early studies of genetics and molecular biology, but the introduction of CRISPR technology marked a significant turning point. Prior to CRISPR, traditional techniques of gene editing were often labor-intensive and required considerable time and resources. Methods such as zinc-finger nucleases and transcription activator-like effector nucleases showed promise but were limited in their applicability and ease of use.
CRISPR first drew attention in the early 2010s, when scientists discovered that a particular bacterial immune system could be repurposed for gene editing. The identification of gRNA's role within this system allowed for a new era of precise genetic modifications. This cutting-edge technology has since sparked a global interest in genetic research and applications, paving the way for various innovations in medicine, agriculture, and more. Understanding the historical developments that led to the emergence of gRNA is essential for recognizing its current applications and future potential.
The Mechanism of gRNA Function
The mechanism of gRNA function is central to the efficiency and precision of gene editing technologies. gRNA acts as the molecular guide that directs Cas proteins, such as Cas9, to specific locations on the DNA strand. Understanding this mechanism is critical for researchers and practitioners aiming to harness the full potential of CRISPR systems. The ability to target DNA with high accuracy minimizes undesired effects and enhances the reliability of genetic modifications.
Interaction with Cas Proteins
The interaction between gRNA and Cas proteins is fundamental within the CRISPR technology framework. Once the gRNA is synthesized, it forms a complex with the Cas protein, typically Cas9. This complex is guided by the complementary sequence of the gRNA to locate the matching DNA sequence in the genome. The affinity and specificity of this binding determine the efficiency of gene editing.
An efficient interaction relies on precise sequence homology between the gRNA and target DNA. If the gRNA binds inaccurately, the editing process can be compromised, leading to off-target effects. Thus, enhancing the understanding of this interaction can lead to more effective design strategies, improving the outcomes of genetic engineering efforts.
Target Sequence Recognition
Target sequence recognition is a crucial step in the gene editing process. The gRNA includes a complementary sequence that matches the DNA target, typically 20 nucleotides long. Efficiency in this recognition is vital, as the Cas protein will cut the DNA strand only when the gRNA finds its target.
In the presence of a protospacer adjacent motif (PAM), a short sequence adjacent to the target DNA that Cas proteins require for binding, the gRNA can accurately guide Cas proteins to the correct location. Understanding the specifics of PAM sequences necessary for the Cas protein's activity is essential.
Improving the specificity of target sequence recognition remains a key concern for scientists. Developing more refined gRNAs allows for targeted edits with reduced likelihood of unintended consequences.
Formation of the Ribonucleoprotein Complex
The formation of the ribonucleoprotein complex, where the gRNA and Cas protein come together, is pivotal for successful gene editing. This assembly is not merely a physical interaction; it involves intricate biochemistry that enhances the stability and functionality of the complex. Once formed, this complex is ready to engage with the target DNA, signifying the beginning of the editing process.
The transition from simple gRNA-Cas protein assembly to a functional ribonucleoprotein complex involves conformational changes. These changes ensure that the complex is optimally prepared to execute the cleavage of DNA at the targeted site. Understanding the dynamics of this formation can lead to innovations in gRNA and Cas protein design, ultimately improving the targeted editing potential of genetic tools.
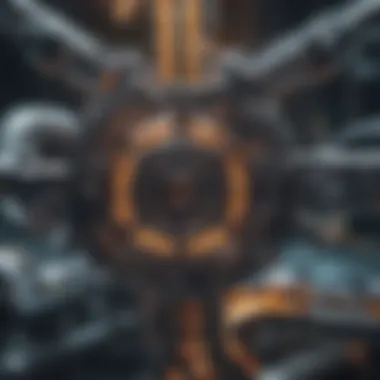
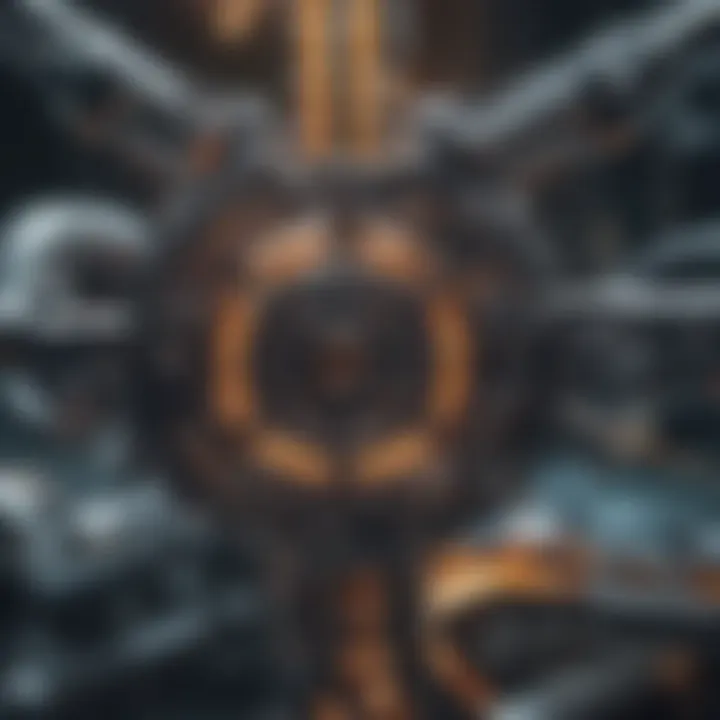
Key Takeaway: The proper understanding of gRNA mechanisms enhances precision in genomic editing. Addressing interactions with Cas proteins, sequence recognition, and complex formation is fundamental for advancing gene editing technologies.
Designing gRNA for Gene Editing
Designing effective guide RNA (gRNA) is pivotal in the realm of genetic engineering, particularly within CRISPR technologies. A thoughtfully designed gRNA enhances the precision and efficiency of genome editing efforts. This section delves into the elements that govern effective gRNA design, including its criteria for functionality and various construction techniques employed in research and application.
Criteria for Effective gRNA
Effective gRNA must meet several criteria to ensure optimal performance in gene editing. Key elements include:
- Specificity: gRNA must target its intended genomic location without impacting other areas. This specificity is vital to avoid off-target effects that may lead to unintended consequences.
- Length: An ideal gRNA typically ranges from 20 to 24 nucleotides. This length is crucial as it provides sufficient binding strength to the target DNA while still allowing for some flexibility in recognition.
- Secondary Structure: The gRNA should maintain a stable configuration. Misfolding can hinder its ability to bind effectively with Cas proteins.
- Custom Adaptations: Depending on the gene editing goals, adjustments may be necessary. For instance, the inclusion of specific sequences such as the protospacer adjacent motif (PAM) can enhance target identification.
"The effectiveness of gRNA can significantly dictate the success rate of gene editing endeavors."
gRNA Construction Techniques
Constructing gRNA involves various technical approaches that are pivotal for ensuring quality design. Common methods include:
- In Vitro Transcription: This technique allows researchers to design the sequence electronically and then synthesize gRNA in the lab. It is a widely used method for generating large quantities of gRNA.
- Synthetic gRNA Production: Companies provide custom gRNA synthesis based on specific design criteria. This option can save time and labor, delivering ready-to-use gRNA.
- Genome-Wide Libraries: Constructing gRNA libraries enables high-throughput screening. Researchers can explore numerous gRNA sequences simultaneously, identifying the most effective candidates for further studies.
- Cloning into Vectors: gRNA can also be cloned into plasmid vectors. This method allows for stable expression in cells, facilitating long-term studies of gene function.
By focusing on these criteria and construction techniques, researchers can maximize the effectiveness of gRNA, thereby enhancing the potential of gene editing technologies.
Applications of gRNA in Biotechnology
The applications of gRNA in biotechnology represent a critical area of innovation and development. As guide RNA serves to bind to target DNA through base pairing, it allows for precise modifications in genetic sequences, thereby enhancing the overall versatility of biotechnological tools. This is particularly evident in areas such as gene editing, which has gained mainstream attention due to its wide array of potential applications.
The role of gRNA in CRISPR technology should be emphasized, as this system has transformed genetic engineering. The ability of gRNA to direct the Cas9 protein to specific sites in the genome has facilitated targeted editing with remarkable precision. This not only streamlines the process of gene modification but also reduces the chance of unintended genetic changes, ushering in a new era of genetic research.
The benefits of gRNA applications are numerous. It contributes to advances in agriculture, medicine, and beyond. For instance, in crop improvement, gRNA strategies can enhance traits such as drought resistance or pest tolerance. In medicine, gRNA is pivotal in developing gene therapies for genetic disorders, underscoring its broad applicability across disciplines.
However, there are considerations that researchers must account for when using gRNA. The stability and efficiency of gRNA during gene editing can vary. Furthermore, off-target effects present a challenge that requires careful evaluation. Researchers are continuously working on optimizing gRNA designs and delivery mechanisms to ensure precision while minimizing unwanted alterations. Overall, the applications of gRNA in biotechnology hold the potential to revolutionize multiple fields while also demanding rigorous ethical scrutiny and technical diligence.
Utilization in CRISPR-Cas9 Systems
Guide RNA is essential in CRISPR-Cas9 systems for precise gene editing. When designed correctly, gRNA leads the Cas9 nuclease to specific genomic locations, enabling targeted cuts. This targeted action is vital for any subsequent gene insertion or deletion, bringing about desired genetic changes with minimal collateral effects. Studies have shown that the specificity of gRNA significantly influences the success rate of CRISPR applications.
Moreover, the adaptability of gRNA facilitates its application to various organisms. By changing the gRNA sequence, researchers can modify genes across species, from plants to animals and even in human cells. This flexibility has driven advancements in therapeutic approaches aimed at combating genetic disorders.
Potential in Gene Therapy
In the context of gene therapy, gRNA plays a transformative role. By guiding the editing machinery to faulty genes, it facilitates the repair or replacement of defective sequences. For diseases caused by single-gene mutations, such as cystic fibrosis or sickle cell anemia, gRNA holds particular promise. Successful edits can restore normal function, paving the way for potential cures.
Gene therapy using gRNA also presents challenges. Off-target effects can result in unintended mutations, raising safety concerns in clinical applications. Striking the right balance between efficacy and safety is paramount for further development in this area. As research progresses, optimizing gRNA design will likely enhance its applicability in patient therapies, presenting exciting possibilities for the future of medicine.
gRNA in Agricultural Biotechnology
The application of gRNA in agricultural biotechnology is another area where its impact is significant. Modification of crops through gRNA technology can produce plants with enhanced resilience to environmental stresses or improved nutritional profiles. Gene editing can be employed to introduce traits that are beneficial for crop yield or resistance to diseases.
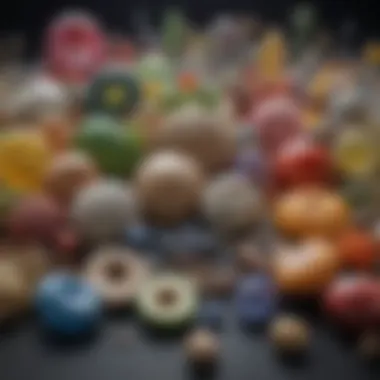
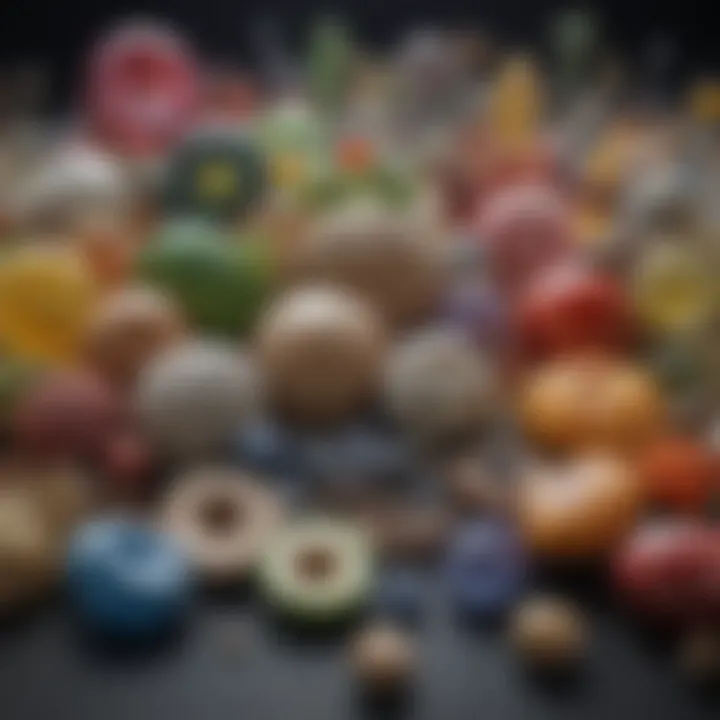
As agricultural challenges evolve due to climate change and global demands, gRNA offers innovative solutions. Through targeted breeding techniques, farmers can cultivate crops that better withstand changing conditions. Furthermore, such technologies may reduce the reliance on chemical pesticides, promoting more sustainable farming practices. The potential to address food security issues through gRNA is profound and highlights the importance of ongoing research in this field.
The integration of gRNA into biotechnology exemplifies the crossroads of science and practical application. Each use case underscores its pivotal role in advancing our technological capabilities while necessitating ongoing ethical and practical considerations.
Ethical Considerations of gRNA Usage
As guide RNA plays an important role in genetic engineering, it raises profound ethical considerations that must be addressed. The advent of CRISPR technology enhances our ability to manipulate genetic material, yet this comes with responsibilities. Discussions around the ethical implications of gRNA usage are essential to ensure the safety and efficacy of these innovations. This section will focus on the impacts on biodiversity and concerns in human gene editing.
Impacts on Biodiversity
The application of gRNA technology has effects on biodiversity that require careful contemplation. The ability to edit genes in organisms can lead to unintended consequences. For instance, altering specific traits in crops could enhance yields but might also disrupt local ecosystems.
Potential repercussions include:
- Loss of genetic diversity: Focusing on a select number of edited species may decrease the overall genetic pool.
- Invasive species: Genetically modified organisms might outcompete native species, altering habitats.
- Unintended gene flow: The transfer of modified genes to other species can have unforeseen outcomes.
It is crucial to establish regulatory frameworks that assess these risks before deploying gRNA technologies in environmental contexts. Addressing the balance between innovation and preserving biodiversity is a pressing ethical obligation for researchers.
Concerns in Human Gene Editing
The use of gRNA in human gene editing elicits significant ethical questions. Not only does it target potential genetic diseases, but it also opens discussions about the implications of editing the human germline.
Key considerations include:
- Informed consent: Patients must understand the risks and benefits of gene editing before proceeding with treatment.
- Equity in access: There is a need to ensure equitable access to gene editing technologies across different populations.
- Potential for designer genes: There is a risk of creating socioeconomic divides based on who can access these technologies for enhancements.
"While gRNA offers pathways to rectify genetic disorders, it is vital to critically think about the long-term effects on future generations and social structures."
Each of these points reflects the importance of operating within an ethical framework when conducting research involving gRNA. Only through careful consideration can we navigate the complexities associated with these technologies while maintaining societal trust and safety.
In summary, understanding the ethical considerations surrounding gRNA usage not only informs responsible research but also encourages a more conscientious approach to genetic engineering as a whole.
Challenges in gRNA Research and Application
Understanding the challenges in gRNA research and application is critical for advancing genetic engineering technologies. As gRNA plays a pivotal role in CRISPR systems, addressing these challenges can maximize both the effectiveness and safety of gene editing initiatives. The implications of these difficulties are far-reaching, affecting both research outcomes and therapeutic advancements.
Off-Target Effects
Off-target effects represent a significant concern in the field of genetic engineering. These unintended modifications can occur when gRNA binds to genomic sequences that are similar, but not identical, to the intended target. A variety of factors influence off-target activity, including the sequence specificity of the gRNA and the activity of the associated Cas protein. Research has shown that off-target effects could lead to unintended consequences, such as unwanted mutations or disruptions in gene function.
Addressing off-target effects requires careful design of gRNA. Applying computational tools can help predict potential off-target sites. Additionally, experimental validation of gRNA specificity is necessary to confirm that edits are localized to intended regions of the genome. Researchers are exploring more advanced gRNA design strategies to enhance precision, including the use of truncated gRNAs that retain targeting capability while minimizing off-target binding.
Off-target effects pose a significant challenge in ensuring the safety and efficiency of CRISPR systems. Addressing these effects is crucial for therapeutic applications.
Delivery Mechanisms in Target Cells
The delivery of gRNA into target cells is another major challenge in the application of genetic engineering. Various delivery mechanisms have been developed, but each presents its own limitations regarding efficiency, safety, and efficacy. Common methods include viral vectors, electroporation, and lipid nanoparticles. While viral delivery is efficient, it raises concerns about immunogenicity and insertional mutagenesis.
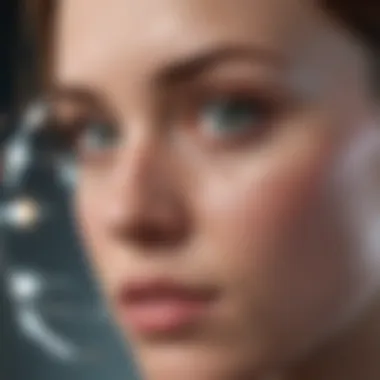
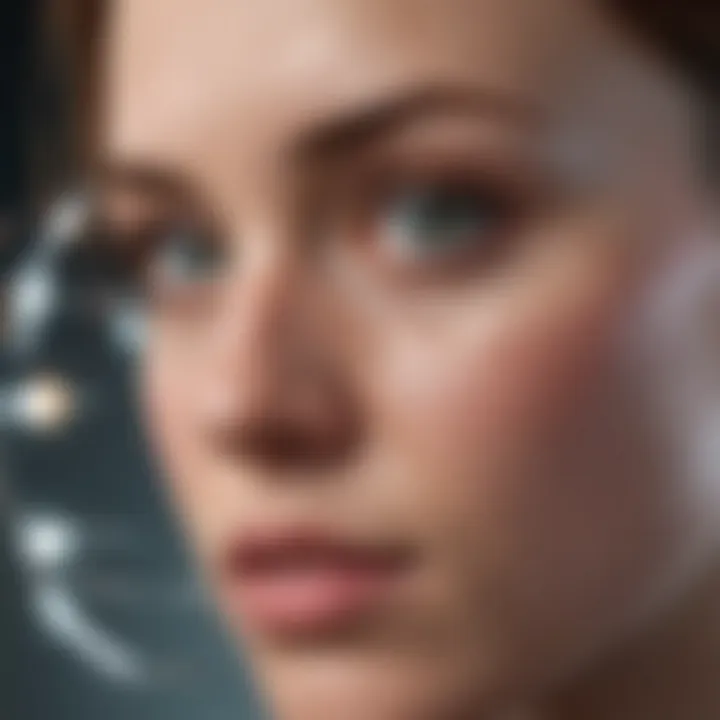
Lipid nanoparticles, on the other hand, offer a less invasive option for delivering RNA. However, achieving the right balance between effectiveness and cellular uptake remains challenging. Selecting the appropriate delivery mechanism becomes essential based on the cell type and the desired outcomes of the gene editing process.
Innovations in delivery methods are ongoing. Researchers are investigating nanoparticles that can navigate cellular barriers or techniques that could enhance the stability of gRNA in biological environments. Ultimately, developing reliable delivery methods will greatly improve the integration of gRNA into gene therapy applications.
Optimization of gRNA Efficiency
Optimizing gRNA efficiency is vital for improving the overall success rate of CRISPR technologies. Factors such as the length of the gRNA, the presence of secondary structures, and its thermodynamic properties play key roles in the efficiency of target recognition and binding. Optimal design can significantly reduce edit variations, thereby increasing consistency in gene modifications.
There are several strategies for enhancing gRNA efficiency. Systems biology approaches can be employed to comprehensively analyze the transcriptional landscape in cells. Furthermore, conducting systematic evaluations of gRNA variants can reveal which sequences perform best under certain conditions.
In addition, measuring the binding dynamics between gRNA and its target can offer insights into the mechanisms that dictate efficiency. By focusing on optimizing these elements, researchers can enhance gRNA performance, ultimately driving forward developments in gene editing.
Future Directions in gRNA Research
The exploration of future directions in gRNA research has become paramount in the realm of genetic engineering. As the scientific community continues to unlock the potential of gRNA, various factors underscore its significance. Innovations in technology and methodologies can enhance the precision and efficiency of genetic modifications. Understanding the long-term implications of gRNA use is critical for its integration into therapeutic applications, agriculture, and basic research.
Additionally, public perception and ethical considerations are becoming more crucial as the techniques become widespread. Addressing these concerns directly impacts funding and regulatory approvals. Thus, it is also about creating a framework for safe and responsible integration into modern science. The need for continual dialogue with stakeholders about the transformative potential of gRNA cannot be overstated.
Innovations in gRNA Technology
Innovations in gRNA technology are redefining gene editing capabilities. One of the notable advancements includes the development of next-generation sequencing techniques that enhance the design and validation of gRNA sequences. Researchers now possess tools that improve the specificity of gRNA, significantly reducing off-target effects. Moreover, modifications in the structural elements of gRNA, such as optimizing the scaffold region, have been shown to enhance the performance of CRISPR-Cas systems.
For example, high-throughput screening methods enable the examination of multiple gRNA candidates simultaneously, thus facilitating the identification of optimal sequences for a desired gene target. Enhancements in computational design tools also play a crucial role in predicting how gRNA will interact with target DNA sequences. These technologies provide a foundation for advancing not only the feasibility of uses in existing applications but also in new emerging fields.
Broadening Application Horizons
Broadening application horizons for gRNA is an exciting frontier in the study of genetic modifications. Beyond the well-known applications in gene editing and agricultural biotechnology, gRNA shows potential in other pivotal areas. This includes the possibility of using gRNA in antifungal and antibacterial treatments. Researchers explore ways to leverage these capabilities to combat resistant strains of pathogens in clinical and agricultural settings.
Positive developments in gene therapy are also noteworthy. Using gRNA to target specific genes can potentially correct genetic disorders by restoring proper gene function. Additionally, its application in synthetic biology for the engineering of microbial systems is under keen investigation. This could lead to bioengineering enzymes for bioremediation or the production of biofuels.
In summary, the future of gRNA research is vibrant and essential. Continuous improvements in technology guarantee that the advantages of gRNA will touch various facets of genetics, therapeutics, and the environment, paving the way for novel solutions to complex scientific challenges.
Finale
The significance of guide RNA (gRNA) in the realm of genetic engineering cannot be overstated. As a crucial component in CRISPR technology, gRNA serves to direct Cas proteins with precision to specific genomic locations, allowing for targeted modifications. This precision fosters innovation in multiple fields ranging from medicine to agriculture, reinforcing the potential for gRNA to incite transformative change.
Summarizing the Importance of gRNA
gRNA is not just a tool; it represents a paradigm shift in our approach to genetic modification. Key factors illustrating its importance include:
- Target Specificity: gRNA enhances the precision with which gene editing occurs, reducing the risk of off-target effects. This is vital for therapeutic applications where unintended edits can lead to complications.
- Customization: The ability to design gRNA for specific genes allows researchers to tailor their interventions. Customization paves the way for new therapies for genetic disorders.
- Diversity of Applications: Beyond human health, gRNA is instrumental in agriculture, enabling the development of crops that are more resilient to diseases and environmental stressors.
In summary, gRNA is foundational to advancements in gene editing techniques, solidifying its role in future biotechnological innovations.
Final Insights on Ethical Research Practices
As the applications of gRNA continue to expand, considerations around ethical practices are paramount. Here are some considerations:
- Informed Consent: Researchers must ensure that participants in gene editing studies are fully informed, as decisions can have far-reaching implications.
- Biodiversity: Gene editing can affect ecological balance, making it essential to proceed cautiously with applications in agriculture and wildlife.
- Regulatory Frameworks: Effective regulations are necessary to govern the use of gRNA, especially in human gene editing, to prevent misuse.
The ethical considerations surrounding gRNA usage require a complex balance of scientific advancement and societal responsibility.
Thus, ongoing discussions about the ethical implications in gRNA research are essential. Balancing innovation with ethical integrity will help ensure that the benefits of gRNA are realized while minimizing potential risks.