Understanding Wavelength Measurement in Science
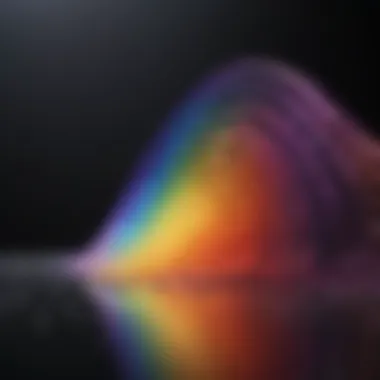
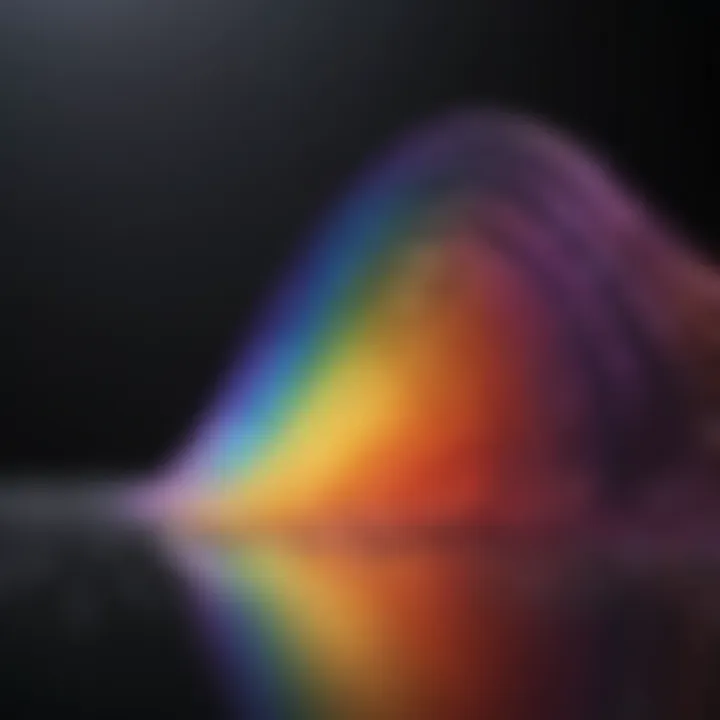
Intro
Wavelength is a fundamental concept in physics and other scientific disciplines. It expresses the distance between successive crests or troughs of a wave. Understanding how to measure wavelength is crucial in various applications, from telecommunications to medical technology. This section will explore the importance of accurate wavelength measurement and its effects in real-world scenarios.
Research Overview
Summary of Key Findings
Measuring wavelength accurately has significant implications in multiple fields. For example, in optics, the precision of wavelength measurements impacts lens design and the effectiveness of lasers used in surgeries. In telecommunications, accurate measurements enhance signal transmission and reception capabilities.
In this article, we highlight methods utilized for measuring wavelength, including the use of spectrometers and diffraction gratings. Each method offers unique insights and varying degrees of precision.
Importance of the Research
Accurate measurements can influence the development of new technologies, enhance scientific research, and improve educational approaches. Whether it is a student grasping the concept for the first time or a researcher conducting advanced experiments, mastering the principles of wavelength measurement is indispensable.
"The accuracy of wavelength measurement can significantly affect the outcome of experiments and practical applications across several scientific domains."
Methodology
Study Design
The methodologies for measuring wavelength can vary based on the desired precision and the specific application. This ranges from simple techniques suitable for educational environments to complex and specialized methods used in scientific research.
Data Collection Techniques
Common techniques for measuring wavelength include:
- Spectroscopy: Analyzing the spectrum of light emitted or absorbed by substances to determine their wavelengths.
- Diffraction Grating: Using a surface with a regular pattern to measure the angles at which light is diffracted to calculate wavelength.
- Interferometry: Utilizing the interference of light waves to measure changes in path length, which correlates to wavelength.
These techniques allow researchers to obtain precise wavelength measurements, contributing to advancements in technology and scientific discovery.
Prelims to Wavelength
Wavelength is a fundamental aspect of wave physics that underlies many scientific principles and applications. Understanding wavelength helps in various fields such as physics, engineering, and environmental science.
This section lays the foundation for the entire discussion on wavelength measurement by presenting its definition and exploring its significance in the scientific community.
Definition of Wavelength
Wavelength refers to the distance between successive peaks or troughs of a wave. This concept applies to different wave types, including sound waves, electromagnetic waves, and water waves. The measuring unit for wavelength often varies depending on the context, with meters, nanometers, or micrometers being commonly used. For instance, in visible light, the wavelength ranges from about 400 to 700 nanometers.
Mathematically, wavelength is expressed with the symbol ( \lambda ) (lambda) and is commonly associated with the wave's frequency and speed. The relationship between these factors can be described by the equation:
[ \lambda = \fracvf ]
where ( v ) is the wave speed and ( f ) is the frequency. Understanding this relationship is significant as it links wavelength to wave behavior and properties, providing a deeper appreciation of how waves function in various mediums.
Importance of Wavelength in Science
Wavelength is a crucial element across many scientific disciplines. Its measurement impacts areas like telecommunications, medical imaging, and environmental monitoring. The following points outline the importance of wavelength:
- Wave Behavior: Wavelength affects how waves propagate through different materials. Changes in wavelength can lead to phenomena such as refraction or diffraction.
- Energy Relationships: The energy of a wave is inversely related to wavelength. Shorter wavelengths usually equate to higher energy, which is vital in understanding concepts in quantum mechanics.
- Technological Applications: Numerous technologies, including lasers, fiber optics, and radio communication, depend on precise wavelength measurement for optimal performance.
- Scientific Research: Accurate wavelength measurements allow researchers to analyze substances via spectroscopy. This analysis helps in identifying chemical compositions and understanding complex biological processes.
Recognizing the significance of wavelength in science is essential for both theoretical exploration and practical applications. By comprehending how wavelength influences different phenomena, scientists and engineers can innovate and solve complex challenges across numerous fields.
Units of Wavelength Measurement
The measurement of wavelength is central to various scientific disciplines, including physics, telecommunications, and biology. Understanding the units of wavelength is essential to grasp the implications of this measurement in practical applications. Different fields often prefer specific units that best suit their measurement needs and the phenomenon they study. This section explores the primary units of wavelength measurement, their relevance, and specific considerations.
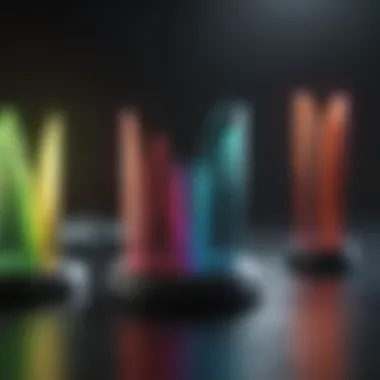
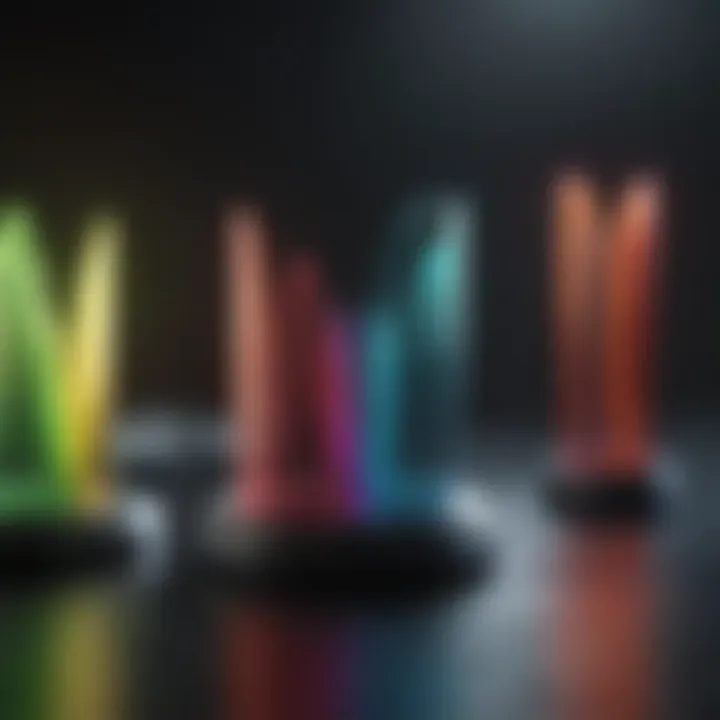
Meters as a Standard Unit
The meter is the internationally accepted standard unit for measuring wavelength. It serves as the foundational measurement in the Metric system. This unit is particularly useful for longer wavelengths, such as those associated with radio waves. When measuring a wavelength in meters, scientists can easily convey distances on a practical scale, making it suitable for a wide range of applications in engineering and technology.
Using meters simplifies calculations and conversions, and it establishes a common framework for scientists across different fields. For instance, in telecommunications, a wavelength of approximately 1 meter is typical for radio frequencies. The versatility of this unit makes it an essential part of wavelength measurement.
Nanometers in Electromagnetic Radiation
Nanometers are another crucial unit of measurement, especially in the context of electromagnetic radiation. One nanometer equals one billionth of a meter (10^-9 meters). This unit is particularly significant in optics and the study of light waves. The visible spectrum, the portion of electromagnetic radiation perceptible to the human eye, spans approximately 380 to 750 nanometers.
In more technical fields, such as quantum mechanics and photonics, understanding wavelengths in nanometers allows researchers to engage with phenomena at atomic and subatomic levels. For instance, ultraviolet light has a wavelength around 10 to 400 nanometers, while infrared radiation ranges from 700 nanometers to 1 millimeter. These distinctions are vital for applications like spectroscopy, where precise wavelength measurements directly correlate with energy levels and transitions in atoms.
Other Units: Micrometers and Picometers
Apart from meters and nanometers, other units like micrometers and picometers have specific applications in scientific measurements. A micrometer represents one millionth of a meter (10^-6 meters) and is often used in fields such as biology and material science. For instance, the wavelength of some infrared radiation can be measured in micrometers, aiding in understanding thermal imaging and other applications.
Picometers, on the other hand, measure one trillionth of a meter (10^-12 meters). This is more common in high-energy physics and fields that involve atomic particles. For instance, the wavelength of gamma rays can fall within this range. By using these smaller units, researchers can accurately describe interactions that occur at minute scales.
"Different units of wavelength not only help define the scale but also allow scientists to navigate the complex relationships between wavelength, energy, and physical phenomena."
Correctly selecting the appropriate unit of measurement is crucial for effective communication among scientists. Ultimately, understanding these diverse units enhances the practical application of wavelength measurement across various scientific disciplines.
Methods of Measuring Wavelength
Understanding the methods of measuring wavelength is crucial in the field of physics and other sciences. Accurate measurement techniques ensure that wavelengths, which influence a variety of phenomena, are documented correctly. This lays the foundation for applications in telecommunications, medical imaging, and environmental monitoring. Each method offers distinct advantages, whether in precision, accessibility, or versatility.
Direct Measurement Techniques
Direct measurement techniques are essential for obtaining wavelength values through hands-on methods. These techniques can be broadly classified into two significant categories: optical methods and mechanical methods.
Optical Methods
Optical methods utilize light to measure wavelength. This approach focuses on the interaction of light waves with various materials and instruments. A key characteristic of optical methods is their high precision. Light's fundamental properties make optical techniques a popular choice for measuring wavelength.
Unique Characteristics of Optical Methods:
Optical methods often utilize lasers due to their coherence and monochromaticity. This allows for sharp and defined measurements, reducing uncertainties that may arise from using broader light sources.
- Advantages:
- Disadvantages:
- High resolution and accuracy
- Easy to use with small instruments
- Non-invasive measurements
- Sensitivity to environmental conditions like temperature and pressure
- Requires calibration against known standards
Mechanical Methods
Mechanical methods employ tangible instruments to gauge wavelength. These techniques are notable for their straightforwardness and reliability. A primary benefit of mechanical methods is their capacity to yield exact results in settings where optical methods may be inadequate.
Unique Characteristics of Mechanical Methods:
Mechanical methods commonly involve devices such as rulers or calipers, especially in scenarios like measuring sound waves or vibrations. The hands-on approach often allows for quicker adjustments.
- Advantages:
- Disadvantages:
- Generally less expensive than optical methods
- Can be used in a broader range of environments
- Straightforward setup and measurements
- Potentially less precise compared to optical methods
- Can be limited by physical constraints of measurement tools
Utilizing Spectroscopy
Spectroscopy plays a pivotal role in measuring wavelength by analyzing the spectrum of light emitted or absorbed by materials. This technique enables scientists to discern the wavelength from the spectral lines, offering invaluable insights into the properties of substances.
Advanced Techniques in Wavelength Measurement
Advanced approaches, such as interferometry and grating techniques, provide enhanced accuracy and detail in wavelength measurement.
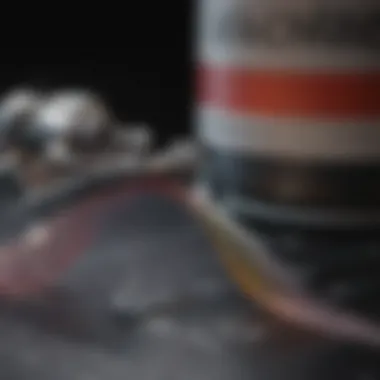
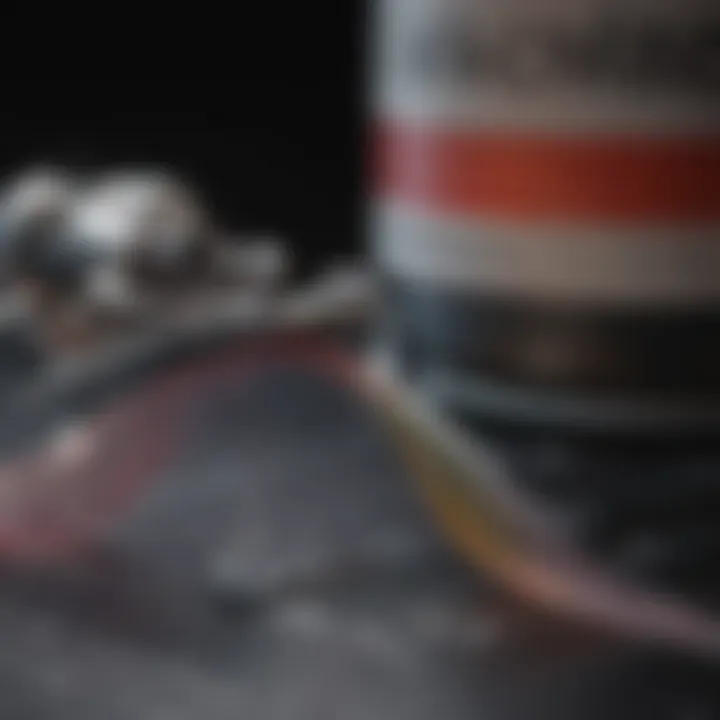
Interferometry
Interferometry stands out for its ability to measure wavelengths with exceptional accuracy. By combining two or more light beams, this method allows for the observation of interference patterns. A notable feature of interferometry is its sensitivity, making it suitable for detecting minute changes in wavelength.
- Advantages:
- Disadvantages:
- Extremely high precision
- Capable of achieving measurements at atomic levels
- Complexity in setup can deter usage
- Requires stable environments to prevent errors
Grating Techniques
Grating techniques involve the use of optical gratings, which diffract light into several beams. This allows for the determination of wavelengths based on the angles at which the light beams are dispersed. A key characteristic of grating techniques is their versatility in applications across various fields.
- Advantages:
- Disadvantages:
- Allows for simultaneous measurement of multiple wavelengths
- Effective over a broad wavelength range
- May require intricate calibration for accurate results
- Sensitive to surface imperfections on the grating
Wavelength and Energy Relationship
The connection between wavelength and energy is fundamental in physics and has wide implications across various scientific disciplines. Understanding this relationship allows scientists to explore and manipulate the properties of waves, be they light, sound, or other forms of electromagnetic radiation. Wavelength is inversely related to energy: shorter wavelengths correspond to higher energy levels, while longer wavelengths have lower energy. This principle plays a crucial role in fields such as quantum mechanics, telecommunications, and medical imaging, as it helps explain how energy is transferred and absorbed in different settings.
Wave-Particle Duality
Wave-particle duality represents a cornerstone of modern physics, illustrating that light and other particles exhibit properties of both waves and particles. This concept is pivotal for explaining how energy interacts with matter. For example, photons—the particles of light—exhibit both wave-like and particle-like behavior, depending on the context of their observation. This duality complicates the measurement and interpretation of wavelengths, as the behavior can shift when energy is applied or observed. Understanding wave-particle duality offers insights into the fundamental principles of nature. It illustrates why precise measurements of wavelength are critical in high-energy physics and quantum experiments where energy states are tightly bound to the wavelength measurements.
Planck's Law and Energy Calculation
Planck's Law describes the energy emitted by black bodies at various wavelengths. This law establishes a direct relationship between the energy of a photon and its wavelength. Specifically, the energy of a photon can be calculated using the formula:
[ E = \frachc\lambda ]
where:
- (E) is the energy of the photon,
- (h) is Planck's constant (6.62607015 × 10^-34 m² kg / s),
- (c) is the speed of light (approximately 3.00 × 10^8 m/s), and
- (\lambda) is the wavelength.
Understanding Planck's Constant
Planck's constant is a unique aspect of quantum mechanics. It quantifies the relationship between the energy of a photon and its frequency. This concept allows calculations that connect wavelength to energy, which is vital for understanding different physical phenomena. The significance of Planck's constant lies in its role as a bridge between macroscopic and microscopic understandings of energy. It facilitates elucidation of processes such as black-body radiation and the photoelectric effect. Without this constant, the bridge connecting classical and quantum physics would be incomplete.
Implications for Quantum Mechanics
The implications of Planck's Law and its connection to wavelength extend into the realm of quantum mechanics. As wavelength affects energy, it sets the stage for numerous quantum mechanical principles such as quantization and the energy levels of electrons within atoms. The absorption and emission of energy at specific wavelengths lead to spectral lines that provide deep insights into atomic structure and behaviors. This understanding allows for advancements in technologies such as lasers and semiconductors, which are crucial in telecommunications and computation. The quantized nature of energy linked to wavelength is a beneficial aspect that drives innovation and exploration in modern physics.
Applications of Wavelength Measurement
Wavelength measurement is not just an academic exercise; it has broad applications across several fields of science and technology. Understanding wavelength and its implications can lead to advancements in communication, medical diagnostics, and environmental assessments. Recognizing these applications helps emphasize why accurate measurement is vital in both everyday and specialized contexts.
In Telecommunications
In the realm of telecommunications, wavelength plays a crucial role. Fiber optic technology, which powers high-speed internet, relies on the manipulation of light signals. The specific wavelengths chosen for transmission can affect the bandwidth and quality of signal. Different wavelengths can also minimize interference, allowing multiple signals to coexist within the same medium. The precision in measurement ensures that networks maintain optimal performance and reliability, which is essential in today’s data-driven society.
In Medical Imaging
Medical imaging techniques utilize wavelengths to visualize and diagnose conditions inside the body.
Uses in MRI and Ultrasound
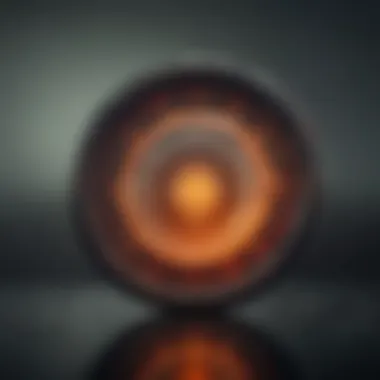
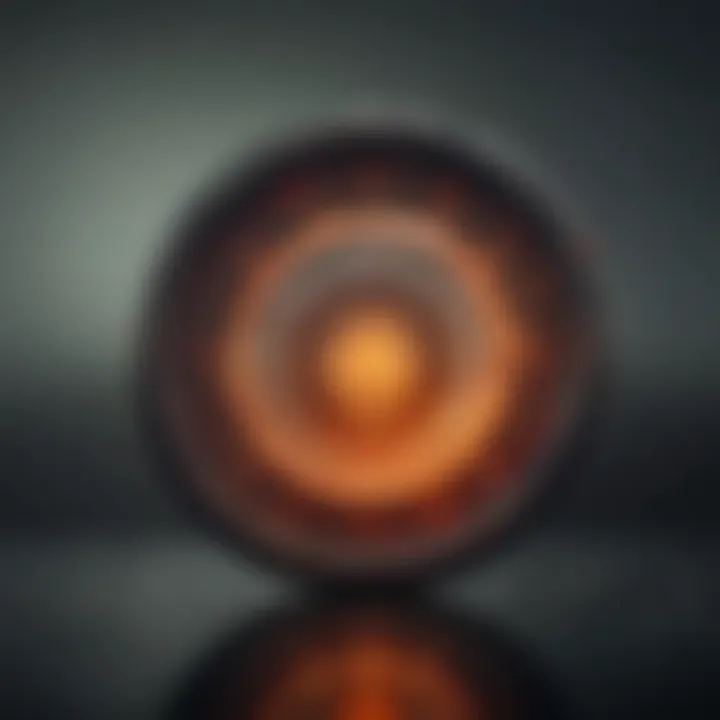
Magnetic Resonance Imaging (MRI) and ultrasound are two prominent examples highlighting the significance of wavelength measurement. MRI uses radio waves emitted by hydrogen atoms in the body when subjected to a magnetic field. The precise measurement of these wavelengths contributes to the clarity of the images produced, allowing for accurate diagnoses. Ultrasound employs high-frequency sound waves; the wavelengths are critical for distinguishing between various tissues and structures within the body. This method is particularly beneficial en in obstetrics and cardiology, where real-time imaging is paramount.
Photon Emission and Detection
Photon emission and detection are critical in various diagnostic tools. Techniques like Positron Emission Tomography (PET) require accurate wavelength measurement to capture the emissions from radioactive tracers in the body. This process helps identify issues at cellular levels. The ability to measure wavelengths accurately ensures that the data gathered from these emissions are reliable and can lead to timely interventions. The precision in measurement enhances the effectiveness of these imaging techniques, thus playing a vital role in patient care and treatment outcomes.
In Environmental Monitoring
Environmental monitoring uses wavelength measurement to analyze pollutants and other elements in ecosystems. Sensors that measure light absorption at specific wavelengths can detect changes in air and water quality. This information is pivotal for environmental scientists and policymakers to address issues like climate change and pollution. By accurately measuring the wavelength of light absorbed by different substances, researchers can draw significant correlations regarding ecosystem health, making it a critical tool for sustainable development.
Challenges in Measuring Wavelength
Measuring wavelength is a fundamental aspect of many scientific disciplines, but it also presents various challenges that can impact the results obtained. These challenges are critical in ensuring reliable and reproducible measurements, especially in precise fields such as physics and engineering. Understanding these difficulties can help scientists and researchers refine their methodologies and improve accuracy in their findings.
Precision and Accuracy Issues
The concepts of precision and accuracy are central to the challenges faced in wavelength measurement. Precision refers to the consistency of measurements, while accuracy denotes how close those measurements are to the true value.
- Instrument Calibration: Instruments must be properly calibrated to yield accurate results. If an instrument is not calibrated correctly, it can produce systematic errors, leading to misleading conclusions. For example, a spectrometer used to measure the wavelength of light needs to be calibrated against known standards to ensure reliability.
- Measurement Techniques: Different techniques for measuring wavelength can yield varying results. For instance, an optical method may deliver different outcomes compared to a mechanical method due to differences in experimental conditions or inherent limitations in tools. This variability requires careful consideration when comparing results from different studies or applications.
- Environmental Factors: Temperature fluctuations and pressure changes can also influence measurement results. Scientists must control these factors in experiments to maintain a stable environment, ensuring that results are both precise and accurate. In some cases, real-time adjustments in measurement techniques are required to compensate for these changes.
"Precision and accuracy are not mere terms; they are fundamental aspects that define the quality of scientific measurement."
Influence of Medium on Wavelength
The medium through which a wave travels significantly affects its wavelength. This influence can cause alterations in measurements and must be well understood.
- Refraction and Diffraction: When waves pass through different media, they bend and change speed, known as refraction. This bending leads to alterations in wavelength, which can result in discrepancies if not accounted for accurately. Diffraction also occurs when waves traverse edges or openings, complicating the measurement further.
- Medium Properties: The optical properties of the medium — such as refractive index — directly impact wavelength. For instance, light travels slower in glass than in air, resulting in a shorter wavelength when measured in the glass. Understanding these transitions is vital for obtaining accurate measurements.
- Wave Interaction: Variations in medium can also influence how waves interact with each other. Constructive and destructive interference can alter amplitude, thereby affecting how wavelength is perceived and measured in specific environments.
Understanding these challenges equips researchers and practitioners to approach wavelength measurement with greater proficiency. Addressing precision and accuracy issues, along with recognizing the influence of different media, can lead to enhanced methodologies and improved outcomes across various fields of science.
Future Developments in Wavelength Measurement
The field of wavelength measurement is rapidly evolving. This evolution is driven by advancements in technology and an increasing understanding of the fundamental principles that govern the behavior of light and other waves. Recognizing the future potential in this area is essential, not only for scientific inquiry but also for practical applications in multiple industries.
Emerging Technologies
New technologies are on the horizon that promise to enhance wavelength measurement. Techniques such as quantum sensing are being explored to improve precision and accuracy. Quantum sensors exploit the principles of quantum mechanics to detect tiny changes in wavelength, which could revolutionize fields like telecommunications and medical imaging.
Another promising development is the integration of artificial intelligence (AI) in data analysis. AI can process vast amounts of data from wavelength measurements, identifying patterns that may not be visible to human researchers. This can lead to breakthroughs in material science and environmental monitoring.
Moreover, advancements in fiber optics technology are expanding the ways wavelengths can be measured and manipulated. Enhanced fiber optic sensors can provide real-time monitoring of environmental conditions, helping in applications from pollution control to climate change studies.
Potential Impacts on Various Fields
The advancements in wavelength measurement technology could have far-reaching consequences across multiple disciplines. In telecommunications, improved wavelength measuring tools can lead to more efficient data transfer methods, boosting internet speeds and reliability. This is particularly significant as the demand for high-speed internet continues to grow.
In the area of medical imaging, precise wavelength measurements can enhance techniques such as MRI and ultrasound. The ability to measure specific wavelengths can allow for better tissue characterization, leading to early disease detection.
In environmental science, enhanced measurement techniques can be key in monitoring pollution levels and health of ecosystems. By employing sophisticated wavelength measurement tools, scientists can gather precise data on atmospheric conditions and chemical compositions more accurately than ever before.
"Understanding and measuring wavelength opens new doors in innovation, impacting fields from healthcare to environmental protection."
In summary, the future developments in wavelength measurement are poised to transform various domains, facilitating innovations that enhance our understanding of natural phenomena and improve our quality of life. Researchers and professionals in these fields must remain adaptive and informed to capitalize on these advancements.
Ending
Recap of Key Points
- Definition: Wavelength is defined as the distance between successive peaks of a wave. Its measurement is crucial for understanding wave dynamics in light and sound.
- Units of Measurement: Common units for measuring wavelength include meters, nanometers, micrometers, and picometers, which provide different scales of precision.
- Methods of Measurement: Various techniques, including direct measurement, spectroscopy, and advanced methods like interferometry and grating techniques, are used to determine wavelength accurately.
- Relation to Energy: The relationship between wavelength and energy, described by principles such as Planck's Law, establishes the basis for understanding wave-particle duality and quantum mechanics.
- Applications: Measurement of wavelength is applicable in numerous fields, influencing technological advancements in telecommunications, medical diagnostics, and environmental assessments.
- Challenges: Issues related to precision and accuracy, and how the medium affects wavelength must be addressed to ensure reliable measurements.
- Future Developments: As emerging technologies evolve, they promise to enhance wavelength measurement capabilities, impacting various scientific fields.
The Significance of Wavelength Measurement
The significance of wavelength measurement cannot be overstated. It serves as a cornerstone in both fundamental research and applied science. Accurate wavelength measurements lead to improved communication technologies, deeper insights into biological processes through medical imaging, and enhanced environmental monitoring techniques.
"Understanding wavelength not only illuminates the physical world but also drives technological innovation."
As we continue to explore the potential of wavelength measurement, our society will likely see advancements that contribute to better scientific understanding and more effective applications in everyday life. The ongoing research and development in this area promise to unlock new possibilities, thereby underscoring the relevance of wavelength measurement in our increasingly technology-driven world.