Advancements in 3D Culture Plates for Biological Research
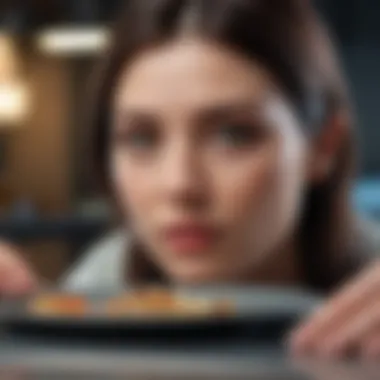
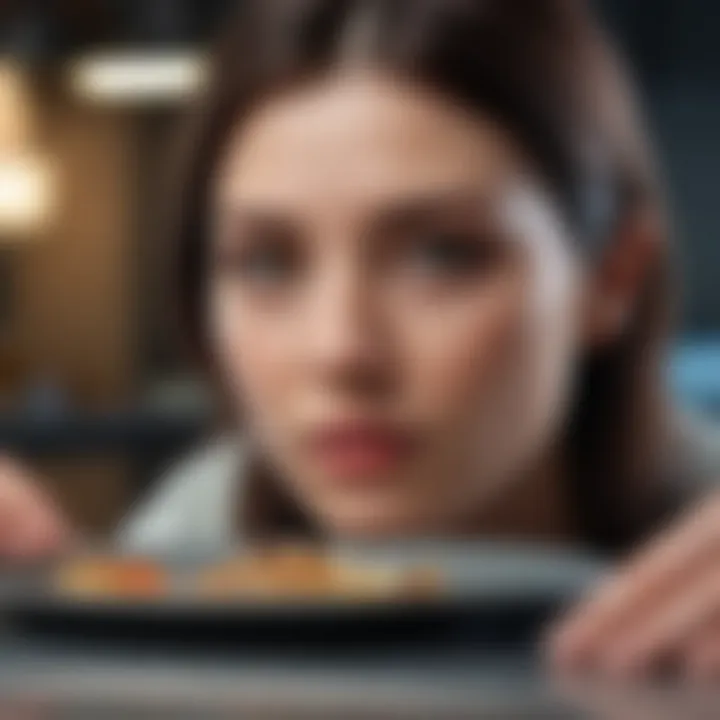
Intro
The landscape of biological research has always been on the move, adapting and evolving to meet the demands of scientific inquiry. At the forefront of this evolution is the emergence of 3D culture plates, shifting the paradigm from traditional 2D cell culture systems to more intricate and representative models of biological tissues. This article takes a closer look at the significance and advancements in the use of 3D culture plates, laying out how these systems have transformed research methodologies and enriched our understanding of cellular behavior.
Research Overview
Summary of Key Findings
The utilization of 3D culture plates offers numerous advantages over their 2D counterparts. Key findings reveal that:
- Enhanced Cell Viability: Cells in 3D environments tend to display increased viability, leading to more reliable results.
- Improved Cell Functionality: These systems promote cellular differentiation and mimic the physiological conditions found in living organisms.
- Better Drug Response: 3D cultures provide a more accurate predictive model for drug testing, allowing researchers to gauge treatment efficacy more effectively.
"Transitioning to 3D cultures can be likened to switching from a flat, simplified map of a city to a detailed three-dimensional model that showcases all the intricacies and nuances."
Importance of the Research
Understanding the role of 3D culture plates is crucial not only for basic research but also for translational applications in medicine. As biomedical science races to develop more personalized and effective therapies, these plates serve as vital tools in the vetting and evolution of treatments before they reach clinical trials. The integration of 3D culture technology can lead to significant breakthroughs in regenerative medicine, cancer research, and pharmacology, ultimately bridging the gap between laboratory discoveries and real-world applications.
By delving into the innovations surrounding 3D culture plates, the implications of their use extend far beyond simple experimentation. They pave the way for more informed clinical outcomes, necessitating ongoing investigation in this essential area of biological study.
Methodology
Study Design
To understand the capability of 3D culture systems fully, a systematic review of various studies published over the last decade is performed. By examining a range of 3D culture types, from hydrogels to scaffold-based systems, the review seeks to identify trends, challenges, and advancements in this ever-evolving field.
Data Collection Techniques
Data collection involves the interdisciplinary collaboration between biologists, bioengineers, and medical professionals to gather comprehensive insights. Techniques include:
- Literature Reviews: Sifting through scientific journals to comprehensively chart 3D culture advancements.
- Experimental Studies: Hands-on experimentation to assess outcomes in cellular behavior relative to traditional practices.
- Surveys: Engaging with researchers in the field to gauge perceptions and experiences regarding the transition to 3D cultures.
This detailed exploration provides a layered perspective to bring clarity around 3D culture plates, highlighting their transformative potential in biological research.
Prologue to 3D Culture Plates
In the pursuit of accurate biological research, 3D culture plates have emerged as a significant advancement, shifting the landscape away from the traditional 2D models that have long dominated laboratory practices. These innovative tools provide an improved environment that better simulates the complexity of living tissues, making them invaluable in both foundational research and applied sciences. As we delve into the nuances of 3D culture systems, understanding their definition, significance, and advantages becomes essential for anyone engaged in biological studies.
Defining 3D Culture Plates
3D culture plates, unlike their 2D counterparts, allow cells to grow in three dimensions. This method is not just a fancy term but represents a paradigm shift in cell biology. In these systems, cells are embedded in a matrix that mimics the extracellular environment, allowing them to grow, interact, and behave more like they would in a living organism. Think of it as a tiny ecosystem where each cell can play its role rather than merely adhering to a flat surface where numerous key interactions are often lost.
A good example of a 3D culture system is the use of hydrogels, which can be engineered to control various factors, such as stiffness and porosity, influencing cell behavior significantly. The capability of 3D culture plates to replicate tissue architecture is a key factor that allows scientists to examine phenomena like drug responses and disease progression more accurately.
Contrast with Traditional 2D Cultures
While 2D cultures have been a mainstay in biological research, they have some shortcomings that cannot be overlooked. Traditional flat cultures impose limitations on cell behavior, which can lead to skewed data. Cells grown on flat surfaces often lose their physiological relevance, showing altered gene expression, signaling pathways, and even metabolic processes compared to what occurs within actual tissue.
The contrast can be stark:
- Cell Viability: In 2D cultures, cell viability might be overestimated because cells often adhere flatly, which may not reflect their health in a living organism.
- Drug Testing: Drug screening in 2D systems may yield inaccurate responses, as the 3D structure of tissues can influence how drugs penetrate and affect cells.
- Tumor Studies: Tumors grown in 3D environments provide insights into how cancer cells behave and evade therapeutic measures, unlike the misleading results seen in 2D systems.
In summary, the move to 3D culture plates embodies a more nuanced and sophisticated approach to studying cellular behaviors and interactions. By providing a system that more closely mirrors real-life biology, researchers are better equipped to glean insights that can influence everything from basic biology to clinical applications.
Technological Advancements in 3D Culture Systems
The progression of 3D culture systems marks a turning point in biological research, reshaping how scientists approach cellular studies. These advancements offer not just incremental benefits but transformative changes in our understanding of biological behaviors, particularly in how cells grow, interact, and respond to external stimuli in a more lifelike environment. The key elements of technological strides in 3D culture include material innovations, improved fabrication techniques, and an overall enhancement of the physiological relevance of experimental conditions.
Materials Used in 3D Culture Plates
When it comes to the materials employed in 3D culture plates, there is quite a range to choose from, each bringing its unique set of properties to the forefront. Hydrogels are among the most widely utilized materials, offering a scaffold that mimics the extracellular matrix. Polyethylene glycol and collagen are common examples. Their biocompatibility allows for better cell survival and growth compared with traditional plastic plates. The incorporation of decellularized tissue also deserves attention. These are sources taken from organs that have had their cellular content removed, allowing a matrix that retains the structural integrity and bioactivity needed for effective cell behavior modeling.

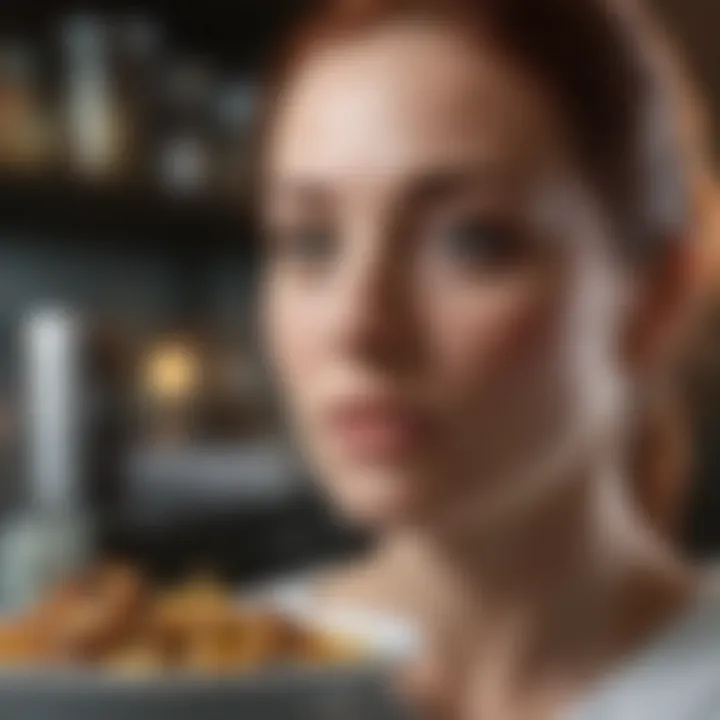
"The choice of material can deeply influence the outcome of experiments, as it dictates cell morphology, viability, and differentiation patterns."
Incorporating natural polymers, like chitosan or fibrin, is another exciting direction. These materials not only support cellular growth but can also be functionalized to present specific biochemical signals, enhancing cell adhesion and promoting intricate tissue formation. Therefore, the selection of suitable materials becomes a pivotal factor in the success of 3D cultures, influencing everything from cell attachment to the ultimate functionality of tissue constructs.
Innovations in Fabrication Techniques
Another significant area of advancement lies in the fabrication techniques for creating 3D culture plates. One notable method is 3D bioprinting, which allows researchers to layer cells alongside biomaterials with precision. This technology enables the creation of structures that closely mimic native tissue architecture, opening new doors for custom tissue design tailored to specific research needs. Moreover, innovations in microfabrication techniques, such as soft lithography, have improved the ability to pattern surfaces on a microscale, further enhancing cell organization and behavior studies.
The advancement of suspension cultures is also noteworthy. By utilizing bioreactor systems where cells are suspended in a nutrient-rich solution, these cultures allow for higher yields, particularly useful for scaling up production in drug testing scenarios. Automated systems for medium exchange and nutrient management have improved the consistency and reliability of these cultures.
In summary, the intersection of novel materials and innovative fabrication techniques in 3D culture systems not only enhances the experimental outcomes but also sets the stage for future exploration in various fields including regenerative medicine, cancer research, and pharmaceutical testing. Each technological leap not only provides researchers with better tools but also deepens our comprehension of biological principles underlying health and disease.
Biological Relevance of 3D Cultures
The significance of 3D cultures reaches far beyond mere technical advancements; it provides a framework for replicating biological scenarios more effectively than traditional 2D systems. As researchers delve deeper into the intricate dance of living cells, the insights derived from these three-dimensional environments have become vital. The reality is that biological systems are inherently three-dimensional, and as such, the methods we employ to study them must also reflect this complexity.
3D culture systems create microenvironments that mimic the natural architecture of tissues. By doing so, they enhance the accuracy and reliability of drug testing and disease modeling. For instance, tumor cells grown in 3D cultures exhibit growth and behavioral patterns that closely resemble those in living organisms, thus providing a better predictive model for cancer treatment. Not only does this relevance drive scientific investigation, but it also elevates the potential therapeutic exploration in clinical settings.
Mimicking In Vivo Environments
When considering the biological relevance of 3D cultures, one of the most impactful advantages lies in their ability to replicate in vivo environments. Utilizing scaffolds made from biomaterials, these systems allow cells to grow and interact in ways that are more similar to what they experience in living organisms. Such an approach is critical for understanding various biological processes such as cell differentiation, tissue development, and pathology.
Moreover, when cells are cultured in 3D, they form architectures resembling those found in tissues. This aspect contributes significantly to the nuances of cell behavior, altering signals and interactions which are often lost in flat cultures. For example, endothelial cells exhibit varying response patterns in 3D when compared to 2D cultures, showing a more reflective behavior in response to stimuli. These patterned interactions inform not just individual cell responses, but the collective behavior and health of tissues.
As researchers continue to explore the boundaries of our scientific understanding, the depth of insight provided by mimicking these environments becomes increasingly apparent. These models not only improve the relevance of laboratory findings but also pave the way for breakthroughs in regenerative medicine and pharmacology.
Cell-Cell Interactions in 3D Models
Cell-cell interactions are a crucial aspect of biological functions and are profoundly affected by the dimensionality of the culture system. In 3D cultures, cells can form more complex connections than in 2D settings. This shift is pivotal, as communication between cells dictates everything from growth and differentiation to apoptosis.
In contrast to their 2D counterparts, where cells engage in simplified communication, cells within 3D constructs establish a multitude of connections due to their spatial orientation. This interconnectedness facilitates a range of signaling pathways that are essential for maintaining homeostasis. For instance, cell types such as fibroblasts and endothelial cells, when used in a 3D model, interact in ways that encourage vascularization, a process critical for tissue regeneration.
Furthermore, the interactions among different cell types in a 3D setting often lead to emergent properties that cannot be predicted from 2D studies. The implications of these interactions are profound, especially in environmental responses under stress conditions such as hypoxia or drug exposure. With an understanding of these cellular dialogues, researchers can better predict how tissues respond to various treatments, thus enhancing the development of new therapeutic strategies.
"Understanding cell-cell interactions in a 3D environment is not just about observing behavior. It's about unlocking the mysteries of how cells work together to create functional tissues."
In summary, the biological relevance of 3D cultures is underscored by their capacity to faithfully replicate in vivo conditions, enhancing our understanding of cellular interactions and developing innovative approaches in medical research.
Applications of 3D Culture Plates
3D culture plates have ushered in a new era in biological research and medical applications. The significance of these plates goes beyond merely growing cells; they allow researchers to explore complex biological interactions, study disease mechanisms, and develop novel therapeutics. The applications of 3D culture systems are vast, yet they can be categorized into three primary fields: cancer research, drug development and testing, and tissue engineering. Each of these areas highlights unique benefits and considerations associated with the use of 3D cultures.
Cancer Research
In the realm of cancer research, 3D culture plates have offered a groundbreaking approach to studying tumor biology. Unlike traditional two-dimensional methods, which can oversimplify tumor growth and response, 3D cultures provide a more authentic environment that mirrors the natural architecture of tissues. This leads to more reliable data regarding cell behavior, metastasis, and treatment responses.
Using 3D cultures, researchers can observe how cancer cells interact with their microenvironment. Factors such as nutrient availability, oxygen gradients, and cellular communication are more accurately represented, allowing for a clearer understanding of tumor pathways. A study by Britannica illustrates that cells grown in 3D cultures tend to exhibit enhanced invasiveness compared to those in 2D layers, aligning closely with in vivo models. Ultimately, this information can help inform targeted therapies, improving outcomes for patients.
"The development of new therapies that effectively treat cancer hinges on the understanding that we gain from 3D models. They bridge the gap between simple lab studies and real-world patient scenarios."
Drug Development and Testing
When it comes to drug development and testing, 3D culture plates prove essential in evaluating drug efficacy and toxicity. Traditional methods often rely on cell lines or animal models that fail to provide an accurate reflection of human responses, leading to high failure rates in clinical trials. In contrast, the use of 3D culture systems enables the development of more predictive models that can simulate human physiology more effectively.
For instance, employing patient-derived tumor organoids in drug testing can offer insights into which therapies may be most effective for a specific cancer type. This patient-centered approach not only increases the likelihood of successful outcomes but also reduces reliance on animal testing, aligning with the ethical standards demanded by today’s scientific community. Researchers are already leveraging these techniques to evaluate the potential of new medications before entering the costly and time-consuming phases of clinical trials.
Tissue Engineering
Tissue engineering stands to gain immensely from the adoption of 3D culture plates. This field aims to develop viable tissue constructs that can replace or repair damaged organs. 3D cultures allow for the growth of interconnected cellular systems that can better replicate the functionality and integrity of human tissues.
Utilizing biomaterials and stem cells, scientists can create scaffolds that support the geometric arrangement and biological functions of native tissues. For example, in regenerative medicine, researchers have successfully engineered skin, cartilage, and even vascular tissues using these methods. This not only holds promise for organ transplantation and reducing donor shortages but also opens doors for studying disease mechanisms in a controlled yet realistic environment.
The integration of 3D culture technologies in tissue engineering poses challenges, however. Researchers must consider factors like angiogenesis, tissue integration, and long-term viability. Yet, as advancements continue to unfold, the potential for creating complex tissue constructs that could one day be used in transplantation is an exciting prospect.
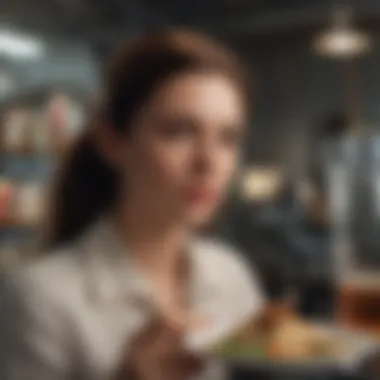
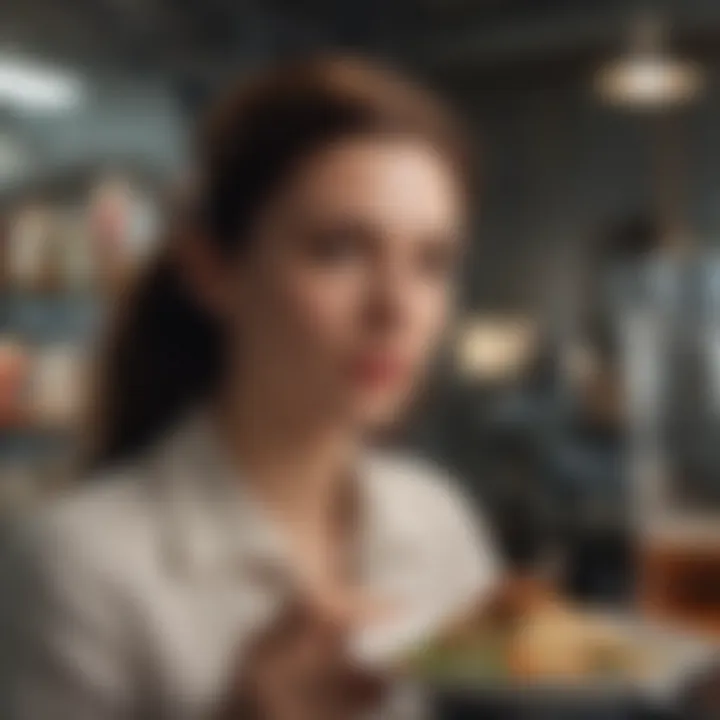
In summary, the applications of 3D culture plates are reshaping how biological research is conducted. From cancer therapy design to drug evaluation and tissue regeneration, the benefits stand tall against the traditional practices that once dominated the field. As the technology continues to evolve, so too does our understanding of biology, ultimately driving us toward more effective and personalized medical treatments.
Comparative Analysis of Efficacy
Understanding the efficacy of 3D culture plates compared to traditional 2D systems is key to appreciating the advancements in biological research. The differences in growth environments and the subsequent biological responses are significant. This part of the article dissects various elements related to efficacy, highlighting benefits and considerations that researchers must keep in mind while making the switch.
Assessing Viability and Growth Patterns
In this section, it's essential to evaluate how well cells thrive in 3D culture plates versus 2D cultures. In traditional 2D systems, cells often exhibit flattened morphology, which does not accurately represent their natural state. This results in altered functionality and impaired cell behavior.
3D culture plates allow for a more realistic environment. Cells can interact in a three-dimensional manner, promoting norms they would experience in vivo.
In a study published by Katt et al. (2020), it was observed that early-stage cancer cells exhibited improved proliferation rates and viability when grown in a 3D matrix. This suggests that the microenvironment induced by 3D cultures can influence growth patterns, allowing for more robust experimental data that better mimics physiological conditions.
- Cell Viability: Assessing how well cells can survive and thrive in their respective environments is crucial. In 3D systems, higher viability rates were noted due to more favorable interactions with surrounding cells.
- Growth Rate: The growth patterns display divergence in terms of cell density and morphology. In 3D cultures, a denser aggregate formation is common, resulting in complex cell dynamics.
Gene Expression Profiling
A major advantage of 3D cultures lies in their ability to affect gene expression profiles significantly, a crucial factor that can dictate experimental outcomes. Research has shown that cells in a 3D environment can express genes in a way that closely resembles their behavior in actual tissue.
For example, a study by Albini et al. (2014) indicated notable differences in gene expression profiles between 2D and 3D cultures. Genes related to cellular adhesion, migration, and apoptosis showed enhanced expression in 3D models, reinforcing the importance of using 3D culture plates for accurate biological analysis.
- Comparison of Profiles: By analyzing the RNA profiles, researchers can directly observe the discrepancies and identify key pathways that might not be evident in 2D setups.
- Applications in Research: Such profiling is crucial in fields like cancer research, where understanding tumor microenvironments can lead to more effective drug targets.
"The cell's behavior in a 3D environment often mirrors its behavior in vivo, leading to more applicable results for therapeutic research."
In summation, as we evaluate the comparative efficacy of 3D culture systems, it's clear that they offer substantial improvements in understanding viability, growth patterns, and gene expression. The organic interplay among cells leads to insights that are indispensable for researchers who aim to tackle the intricacies of human biology.
Challenges in Implementing 3D Culture Technologies
The rise of three-dimensional (3D) culture technologies has transformed biological research, offering intricate models that more accurately depict in vivo conditions. However, a deeper understanding of the challenges involved in this shift is essential for future progression. Addressing these obstacles not only highlights existing limitations but also paves the way for enhanced methodologies and better outcomes in research.
Technical Limitations and Obstacles
Despite the promising advantages of 3D culture plates, several technical limitations remain that can hamper their full adoption. One of the primary concerns is the complexity in the fabrication process. Unlike traditional 2D cultures, 3D systems often require specialized equipment and techniques that can be prohibitively expensive. Furthermore, variations in design can lead to inconsistencies which affect the reproducibility of experiments.
Another pressing issue is cell viability. Achieving optimal conditions for cell growth within 3D environments can be tricky. The interactions between cells and their surroundings, as well as nutrient diffusion, can drastically influence the overall cell health and function. This leads to variabilities that researchers cannot afford, especially in nascent fields such as drug testing and regenerative medicine. A further complication arises from the materials used; not all substrates support cell adhesion and growth uniformly, which can muddy result analysis.
- Key Technical Challenges
- Complexity of manufacturing processes
- High costs associated with specialized equipment
- Variability in experimental reproducibility
- Nutrient diffusion issues affecting cell viability
- Diverse material properties leading to inconsistent outcomes
Standardization of Methods
The standardization of methods in utilizing 3D culture technologies poses another layer of challenges, particularly in establishing consistent protocols for experimentation. As of now, the lack of widely accepted guidelines hinders collaboration and comparison across different studies. This non-standardization encourages variability, making it difficult for researchers to validate each other’s findings effectively.
Moreover, the nuances of different 3D culture systems aren’t all fully understood, leading to obstacles in methodology. For example, different scaffolding techniques might yield distinct cell behaviors, but without standardized protocols, drawing comprehensive conclusions across diverse datasets proves arduous.
To overcome these issues, it’s imperative for the scientific community to focus on:
- Developing consensus protocols that all researchers can utilize.
- Collaboratively working towards creating best practices that enhance reliability.
- Encouraging communication among labs to share data and findings, thereby enriching the overall research landscape.
"Standardization is the backbone of reliable scientific inquiry; without it, results remain mere whispers in the crowded hall of knowledge."
In summary, while 3D culture technologies hold great potential for advancing biological research, navigating the challenges of technical limitations and method standardization is crucial. Overcoming these hurdles will not only improve the reliability of research but also enhance the clinical applications of 3D cultures, ultimately benefiting the broader field of medicine.
Future Prospects of 3D Technology in Biological Research
As the field of biological research continues to evolve, the future prospects of 3D culture technology stand as a beacon of advancement. This section aims to shed light on how integrating innovative 3D culture plates with existing and emerging technologies can lead to groundbreaking applications in medicine and therapeutics. Researchers and practitioners alike are increasingly acknowledging the relevance of these advancements, as they promise to address various challenges faced in traditional models.
Integration with Other Technologies
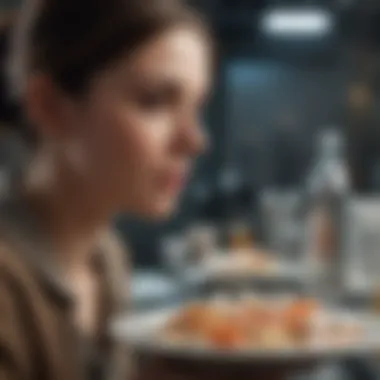
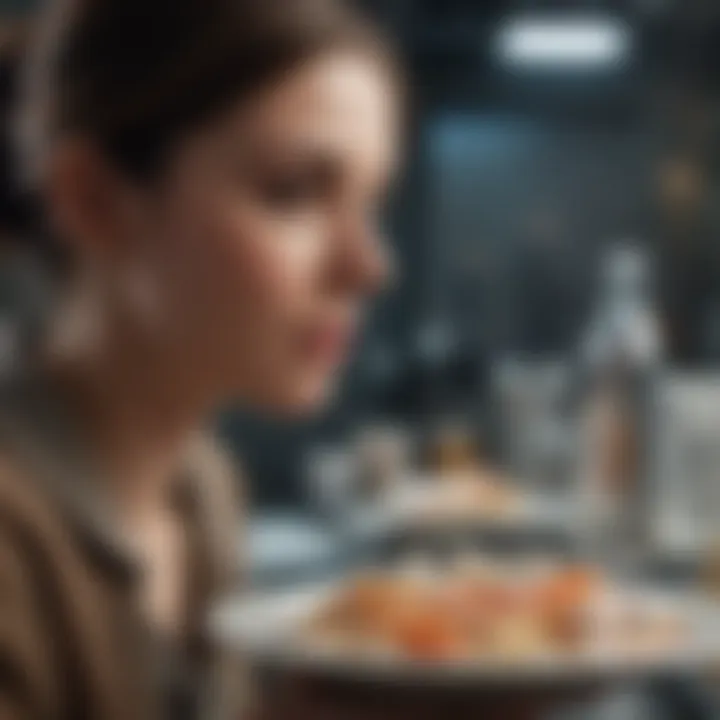
The integration of 3D culture plates with other technologies opens up a world of opportunities. By collaborating with advancements in fields such as bioinformatics, imaging techniques, and robotics, researchers can enhance not only the precision of their studies but also the efficiency of experiments.
- Bioinformatics: With improved data analytics, researchers can analyze vast amounts of biological data, offering insights that would have been impossible with standard techniques. This leads to a more nuanced understanding of cellular behavior in 3D environments.
- Imaging Technologies: Techniques such as micro-CT and advanced microscopy allow for real-time imaging of 3D cultures, providing invaluable data on the microenvironment's dynamics. This means researchers can observe how cells interact within a more realistic context, leading to better models of disease and therapy.
- Robotics: The use of robotic systems enables high-throughput screening of 3D cultures. Such automation minimizes human error and allows for the evaluation of hundreds of compounds in a fraction of the time.
These integrations not only streamline the research process but also enhance the accuracy of biological interpretations.
Potential for Personalized Medicine
Personalized medicine is a growing field aimed at tailoring medical treatment to the individual characteristics of each patient. With 3D culture technology at the forefront, the potential for advancements in personalized therapy becomes more tangible.
3D cultures facilitate the use of patient-derived cells; thus, they provide a platform to study how specific therapies affect unique cell models. By using these personalized models:
- Drug Testing: Researchers can evaluate the efficacy and safety of drugs tailored specifically to a patient’s own cellular environment before actual treatments.
- Predicting Treatment Outcomes: With detailed assessment, scientists can better predict how an individual might respond to a particular drug, ensuring that the patient receives the most effective treatment without unnecessary side effects.
- Disease Modeling: Patient-specific 3D cultures also enable better modeling of diseases, offering insights into disease progression and potential therapeutic targets that are unique to each patient.
This promises not just a theoretical shift in practice but real-world implications for improving patient outcomes.
Ethical Considerations in Using 3D Cultures
The ethical implications surrounding the use of 3D culture plates are as significant as the technical advancements they offer. As research continues to evolve, so too do the considerations surrounding the implementation and application of these novel systems. Understanding these ethical aspects is critical not only in guiding current best practices but also in laying the groundwork for responsible future developments in biological research.
Animal Models and Regulatory Concerns
In biological research, animal models have long been considered a necessary evil for understanding complex human conditions. However, the advent of 3D culture systems offers an alternative that could potentially reduce reliance on live animals for experimentation. While 3D models can’t fully replicate the intricate interactions found within a living organism, they do provide more relevant data than traditional 2D cultures, thus offering a pathway to better align research practices with ethical standards.
The regulatory landscape surrounding animal testing is stringent, designed to ensure humane treatment and minimize suffering. Transitioning to 3D cultures could alleviate some of these regulatory burdens, leading to fewer animals used in studies. However, it remains crucial for researchers to maintain transparency about their methodologies and outcomes, especially as regulatory bodies scrutinize the efficacy of these new systems.
"Embracing a reduction in animal use not only advances ethical concerns but also enhances the credibility of research outcomes."
Human Tissue Usage
The use of human tissue in 3D culture systems presents its own set of ethical dilemmas. Ethical sourcing of tissues is paramount—donors must provide informed consent, understanding how their biological materials will be utilized in research. The guidelines governing human tissue use are stringent and rightly so; they aim to respect individual rights while promoting scientific advancement.
Moreover, when using human tissues, complications arise regarding the fair distribution of research benefits. Equitable access must be considered to avoid exploitation of vulnerable populations. Researchers need to navigate these moral waters carefully, focusing on transparency and respect for individuals contributing to the scientific landscape.
Closure
The exploration of 3D culture plates presents a remarkable advancement in biological research, highlighting their essential role in enhancing the accuracy of various scientific practices. These novel systems allow researchers to create more life-like environments for cell studies, thus bridging the gap between traditional methods and the complexities observed in real biological systems. The differences in outcomes when using 3D cultures versus 2D cultures are monumental; cells within 3D environments behave more like they would in vivo, showing improved viability and more authentic interactions.
Summarizing the Insights Gained
In summary, the insights gained from this discourse shed light on the multifaceted advantages and evolving technologies associated with 3D culture plates. Key takeaways include:
- Enhanced Mimicry of Natural Conditions: 3D cultures closely replicate the extracellular matrix, leading to more accurate cell responses.
- Improved Drug Testing Accuracy: Through a more representative environment, 3D cultures facilitate effective drug development by providing reliable data on how medications affect cells.
- Potential for Customized Treatments: The use of personalized 3D cultures could revolutionize treatment plans tailored to individual patient needs.
Overall, shifts from 2D to 3D systems mark a significant highlight in the methodological evolution of biological research, paving the way for more relevant findings and applications.
The Way Forward for 3D Culture Technologies
Looking ahead, the trajectory for 3D culture technologies appears promising yet sprinkled with challenges. As we aim to integrate these systems thoroughly into mainstream practice, the following aspects deserve close consideration:
- Standardization Proposals: There's a clear need for establishing standardized protocols that will enhance the reproducibility of experiments across different laboratories.
- Technological Merging: Future prospects shine upon the potential fusion of 3D culture technology with other emerging fields, such as bioinformatics and machine learning, to boost data analysis and outcome predictions.
- Regulatory Framework Development: With the increasing application of 3D models, establishing a robust regulatory framework will play a critical role in guiding their ethical and efficient use, ensuring that these technologies are used responsibly in research and therapeutic applications.
In essence, while 3D culture plates have begun reshaping the landscape of biological research, their journey is still in its infancy. Addressing the challenges ahead can ensure these systems fulfill their promise in delivering better, safer healthcare solutions.
Citing Relevant Studies
A variety of studies have documented the advancements and implications of 3D culture plates. For instance, research published in journals like Nature Biotechnology and Tissue Engineering provides necessary insights into how these innovative systems mimic in vivo environments more accurately than traditional 2D cultures. One notable study by Zhang et al. illustrates differences in cellular behavior when cultured in a three-dimensional context, emphasizing greater relevance in drug testing scenarios. It’s a stark reminder of how research findings guide the trajectory of academic and clinical practices.
"The ability to create conditions that closely resemble natural physiological environments enhances the reliability of preclinical data."
By referring to specific studies, researchers not only reinforce their arguments but also lay down a pathway for future inquiries. Some additional key articles include:
- Advanced Drug Delivery Reviews: Discusses the use of 3D cell culture models in drug delivery systems.
- Biomaterials: Examines the engineering aspect of materials used in 3D cultures. This landscape of literature paints a fuller picture of how 3D culture plates are reshaping biological research.
Further Reading Recommendations
For readers seeking to expand their understanding of 3D culture technology, several resources can serve as essential companions:
- Books: "3D Cell Culture: Methods and Protocols" offers practical insights and procedures for implementing 3D cultures in various research environments.
- Articles: The article "Emerging trends in 3D cell culture technologies" available on platforms like ScienceDirect, provides an overview of recent innovations and methodologies.
- Web Resources: Websites such as Britannica and educational portals often compile comprehensive overviews about the applications and advancements of 3D cultures. These can offer broader contexts impossible to achieve through single studies alone.