Exploring Cas9 Proteins and Their Nuclear Signals
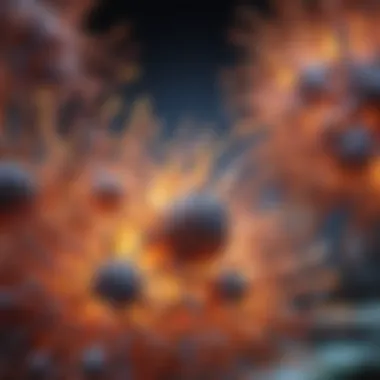
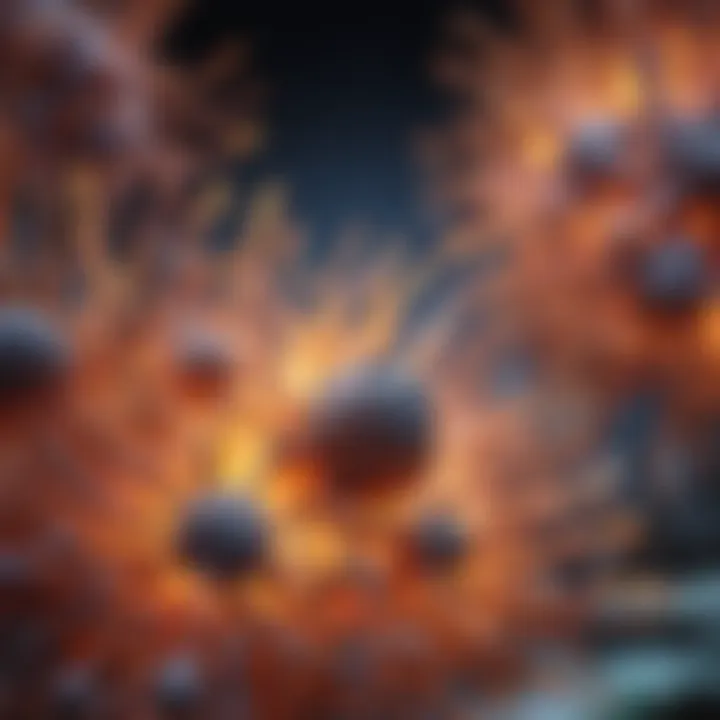
Intro
In the realm of molecular biology, Cas9 proteins have emerged as remarkable tools, particularly known for their pivotal role in gene editing technologies such as CRISPR. At the crux of this innovation lies the concept of nuclear localization signals (NLS), which are critical for the transport and localization of the Cas9 enzyme to the cell nucleus. This article aims to unpack the intricate relationship between Cas9 and its NLS, shedding light on how these elements not only influence gene editing but also shape the future of genetic engineering as a whole.
The story of Cas9 isn't merely about an enzyme's functional role; it delves deep into mechanisms that ensure this protein makes its way into the nucleus effectively. Understanding how NLS work with Cas9 offers valuable insights into optimizing this technology for research and potential therapeutic applications. Each section of this article will be methodically crafted to elucidate the structural intricacies, functional dynamics, and ongoing research in this area.
As we move forward, the upcoming sections will dive into the latest research findings, methodological approaches employed in studies involving Cas9 and its NLS, and the implications of these insights for both academia and industry. The intention here is to create a narrative that is both engaging and informative, suited to the needs of students, researchers, educators, and professionals alike.
Intro to Cas9
The journey into the realm of CRISPR technology begins with a cornerstone protein known as Cas9. Understanding Cas9 is crucial for anyone who aims to grasp the complexities of gene editing. This protein, derived from the adaptive immune system of bacteria, serves as the precision tool for targeting specific DNA sequences. In todayâs context, its implications stretch beyond mere research; they reach into the realms of medicine, agriculture, and biotechnology.
When we wade into the waters of Cas9, weâre not just discussing a single protein but entering an intricate system of interactions, mechanisms, and potential applications. The attention on Cas9 highlights critical aspects pertaining to its functionality, structural significance, and the role of nuclear localization signals (NLS) in its operation.
Historical Perspective on CRISPR-Cas9
To fathom Cas9âs significance today, we must first take a detour into history. The roots of CRISPR trace back to the late 1980s when scientists stumbled upon an unusual sequence in the DNA of certain bacteria, notably Escherichia coli. Fast forward to 2005, researchers recognized that these sequences function as an adaptive immune system, storing genetic material from viruses that previously invaded the bacteria.
From this curious discovery sprung the versatility of CRISPR-Cas systems, and studies in subsequent years highlighted the role of Cas9 as a transformative agent in gene editing. The seminal work of Doudna and Charpentier in 2012, translating this bacterial system into a tool for targeted genome editing, opened the floodgates for a revolution in genetic manipulation.
This historical lens not only elucidates how the scientific community arrived at the current understanding of CRISPR-Cas9 but also emphasizes the continual interaction between basic research and technological advancement. It showcases how scientific curiosity can evolve into powerful tools with transformative potential.
Basic Structure and Origin of Cas9
Delving into the basic structure of Cas9 reveals a protein marvel intricately designed for its function. Structurally, Cas9 consists of two distinct lobes connected by a flexible linker. Itâs the harmonious cooperation of these lobes that enables Cas9 to perform its duties with precision. The protein primarily functions as an RNA-guided endonuclease, meaning it relies on a guide RNA to hone in on specific DNA sequences, inducing double-strand breaks.
The origin of Cas9 lies in a variety of bacterial species, with the Streptococcus pyogenes variant being the most widely used in gene editing applications. The adaptability and modular nature of Cas9 have given rise to myriad engineered variants, each tailored to enhance targeting capabilities or minimize off-target effects.
Understanding the structure and origins of Cas9 lays the groundwork for unraveling its interactions with nuclear localization signals. This understanding is fundamental when considering the practical applications of CRISPR technologyâwhere gene editing is not purely theoretical but manifests in real-world innovations.
Nuclear Localization Signals Explained
Nuclear localization signals are a pivotal aspect of the broader context of gene editing and CRISPR technology. In essence, NLS are the molecular addresses that guide proteins like Cas9 to their rightful placeâthe nucleus of a cell. The significance of NLS extends beyond mere transportation; they embody the mechanism by which the intricate machinery of CRISPR can influence genetic material within the confines of a cell's nucleus. This segment will unpack the importance, the core elements, and the myriad benefits of understanding NLS within the landscape of genetic engineering.
Definition and Function of NLS
To grasp the essence of nuclear localization signals, one must first understand their definition and function. NLS are short amino acid sequences that serve as indicators for a protein's entry into the nucleus. Like a ticket required to board a train, the presence of an NLS enables proteins to navigate through the nuclear pore complex (NPC) and gain access to the nuclear compartment.
In practical terms, think of it this way: without these signals, even the most robust protein like Cas9 would be left knocking at the door, unable to execute its role. The function of NLS is crucial in ensuring that proteins reach their destination in a timely manner, enabling them to engage in various cellular processes, including gene editing. In the landscape of genetic engineering, this is paramountâafter all, you want your tools where they can do the most good.
Types of Nuclear Localization Signals
Understanding the different types of nuclear localization signals is like knowing your options at a workshop: it expands your toolkit and enhances your ability to customize your approach. There are primarily two categories of NLS:
- Classical NLS: These are typically characterized by their basic amino acids, generally arginine and lysine. Classical NLS often consists of either a monopartite or bipartite signal.
- Non-classical NLS: Unlike their classical counterparts, these signals do not follow the same basic residue motif. They can take various forms and may be more complex, engaging with different transport mechanisms.
- Monopartite: A single cluster of basic residues, making it straightforward yet effective.
- Bipartite: Comprising two clusters of basic residues, separated by a flexible linker. This setup allows for enhanced targeting flexibility, resulting in potentially greater nuclear import efficiency.
By having a firm grasp on these types, researchers and professionals can fine-tune their genetic editing tools, making them more effective. Itâs not just about choosing a method; itâs about understanding the nuances of these signals to potentially optimize the delivery of Cas9 to its target genes.
"NLS are like the GPS coordinates for proteinsâcritical for reaching the right destinations within the cellular landscape."
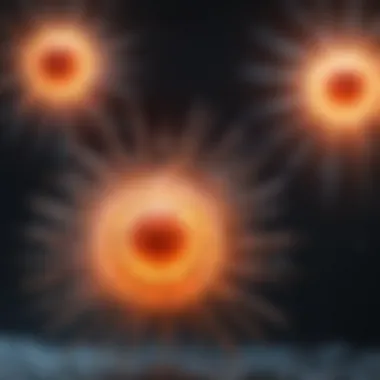
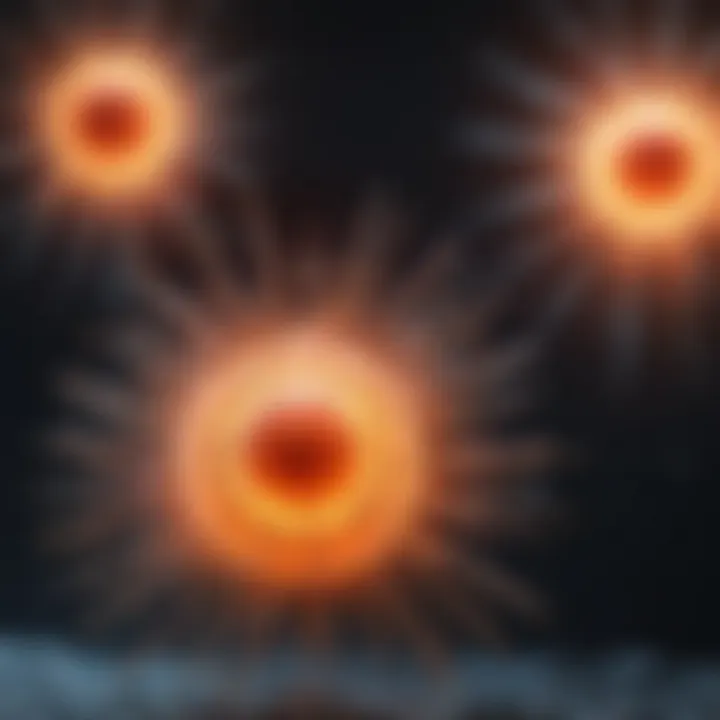
The Mechanism of Cas9 Nuclear Import
The nuclear import of Cas9 is a crucial aspect that underpins its functionality in gene editing. As the Cas9 protein must reach the nucleus to perform its role in DNA modification, understanding the mechanisms of its import is essential. This section highlights various elements, benefits, and considerations regarding Cas9 nuclear entry and how they relate to its effectiveness in genetic engineering.
Transport Pathways: Insights into Mechanism
When examining how Cas9 makes its way into the nucleus, it becomes clear that multiple transport pathways are involved. These primary routes typically employ complex protein interactions and depend largely on specific signals embedded within the Cas9 structure. The significance of this process canât be understated; without proper transport, even the most potent gene-editing tool becomes essentially ineffective.
Viral proteins often inspire insights into these pathways. In nature, viruses like the Human Immunodeficiency Virus (HIV) exploit cellular transport mechanisms to gain nuclear access. Similarly, Cas9 leverages these pathways, utilizing its nuclear localization signals (NLS) often mirrored from such viral strategies to hitch a ride on the cellular transport system.
The transport routes can be categorized into direct and indirect pathways. The direct route implies the protein's entrance is mediated solely by its NLS. On the other hand, indirect pathways may involve interactions with other proteins that facilitate Cas9âs arrival at the nuclear pore complex. Itâs a coordinated ballet of molecular machinations that ensures Cas9 is precisely where it needs to be.
Unraveling these pathways not only enhances our understanding of Cas9 but also lays the groundwork for future modifications in gene editing technologies. By tweaking these pathways, researchers might optimize Cas9âs efficiency, allowing for more effective genome editing.
Role of Importins in Nuclear Localization
Now, letâs consider the role of importins in the nuclear localization of Cas9. Importins are a family of proteins crucial for transporting cargo proteins into the nucleus. Essentially, they act as the ferry service for molecules wishing to enter nuclear territories. The partnership between importins and Cas9 is pivotal.
Upon entry into the cytoplasm, Cas9 recognizes and binds to specific importins through its NLS. Here's how it usually unfolds:
- Binding: The NLS of Cas9 attaches to importins like importin-α or importin-ÎČ.
- Transport: Together, they then travel towards the nucleus through the nuclear pore complex, which acts as a gatekeeper to the nuclear environment.
- Release: Once inside the nucleus, a process often involving Ran-GTP results in the release of Cas9 from importins, permitting it to engage with the DNA.
The presence of multiple types of importins means that the stability and efficiency of Cas9âs nuclear import can vary based on the cellular context and the type of importin engaged. Also, the differential expression levels of these importins can impact gene-editing outcomes.
"Understanding the role of importins illuminates the pathway to innovating Cas9 applications beyond the traditional genome editing approaches."
Thus, the intricate relationship between Cas9 and importins continues to be a rich area for explorationâunraveling this interaction could offer avenues for enhancing the precision and efficiency of gene editing methodologies.
Impact of Cas9 NLS on Gene Editing Efficiency
The effectiveness of CRISPR technology, particularly the Cas9 protein, goes hand in hand with the role of nuclear localization signals (NLS). These sequences are pivotal for ensuring that Cas9 arrives at its intended destination within the cellâthe nucleus. Without efficient nuclear import, even the most accurate gene-editing tool risks becoming ineffective. This aspect holds major implications not just for laboratory research but also for therapeutic applications where precision is paramount.
One of the beauties of NLS is its ability to dictate the targeting accuracy of the Cas9 protein. When the NLS is appropriately designed, it enhances the ability of Cas9 to correctly reach its target DNA sequence, which is crucial when you consider the consequences of off-target editing.
Relationship Between NLS and Targeting Precision
The relationship between NLS and targeting precision is intricate. You can think of NLS as a sort of passport that allows Cas9 to enter the nucleus where it can perform its gene-editing magic. But not all NLS sequences are created equal. Some might afford better transport efficiencyâor in layman's terms, a smoother ride into the nucleus.
Research suggests that NLS sequences rich in basic amino acids often correlate with higher targeting precision. For instance, the classic bipartite NLS, found in proteins like the SV40 large T antigen, provides a robust mechanism for nuclear import. Differences in length and composition can lead to varying levels of nuclear import efficiency, ultimately swaying the levels of editing accuracy within the genome.
"The design of an NLS is crucial for achieving desired gene editing outcomes, marking a definitive shift in how we approach CRISPR technologies."
Enhancements Through NLS Modifications
Given the integral role NLS plays, modifications have been explored to enhance the effectiveness of the Cas9 protein. These include adjustments in amino acid composition and structure, which can offer numerous benefits.
Some innovative strategies involve fusing additional NLS sequences or mutating existing ones. For example, by attaching a stronger or more efficient NLS to Cas9, researchers have been able to improve its nuclear import rates significantly. This modification often translates to a higher likelihood of engaging with the target genome accurately, which is crucial when you're trying to edit specific genes for therapeutic purposes.
Also, the timing of involvement can matter. Engineers have experimented with NLS-modified Cas9 proteins differently based on the cell state, leading to improved engagement with nucleotides during the editing process.
Structural Analysis of Cas9-NLS Interactions
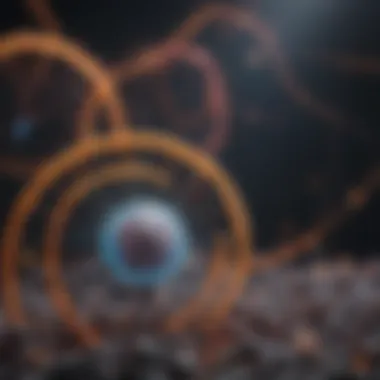
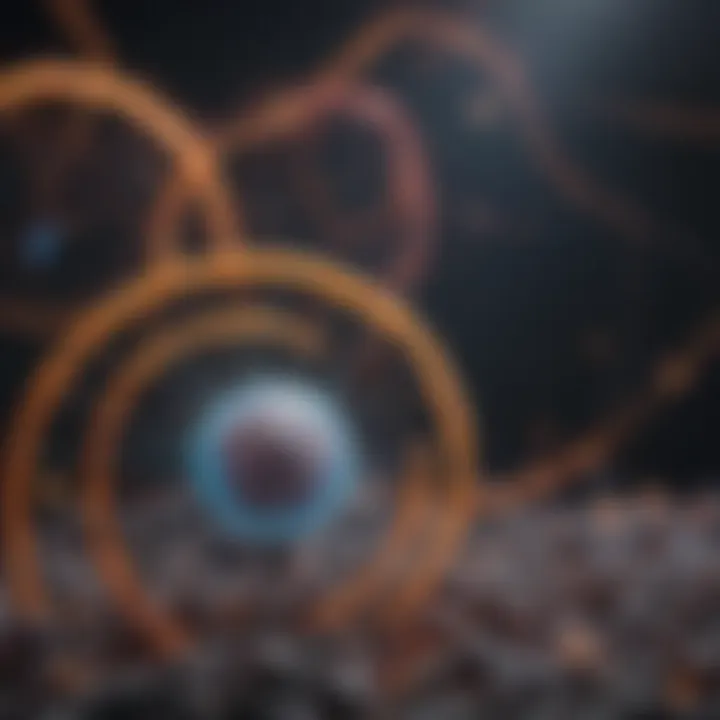
The examination of how Cas9 proteins interact with their nuclear localization signals (NLS) is essential, not just for understanding the fundamental biochemistry behind CRISPR technology, but also for its application in real-world genetic engineering practices. An in-depth structural analysis reveals the intricate molecular machinery that facilitates the nuclear traffic of Cas9, illuminating both its design and functional capabilities.
By understanding the structural nuances of Cas9-NLS interactions, researchers can uncover potential avenues for optimizing the accuracy and efficiency of gene editing. This analysis helps identify specific structural elements that influence binding affinity and transport efficacy, thus providing a platform for potential enhancements in NLS design and overall Cas9 functionality.
X-ray Crystallography Insights
X-ray crystallography has played a pivotal role in the structural elucidation of Cas9-NLS interactions. This method has enabled scientists to visualize Cas9 at an atomic level, where it becomes possible to identify the binding sites for nuclear localization signals.
For instance, detailed structural images produced through X-ray crystallography can clarify fusion relationships and hydrogen bond formations within the protein complex. Such insights are invaluable as they highlight the specificity of NLS engagement with Cas9, which is crucial for ensuring reliable translocation into the nucleus. Regarding the implications of these structural features, consider how alterations in the Cas9 architecture could inadvertently impede its nuclear importâa factor critical to both efficiency and accuracy in gene editing.
Moreover, these crystallographic studies reveal conformational changes in Cas9 upon binding with NLS, demonstrating how structural dynamics can impact function. These details help us bridge the gap between structure and performance, aiding in the design of next-generation genome editing tools.
Biophysical Characterizations of Binding Affinity
Besides X-ray crystallography, biophysical techniques, such as surface plasmon resonance (SPR) and isothermal titration calorimetry (ITC), have emerged as powerful tools in assessing the binding affinity between Cas9 and its NLS. Understanding the binding parameters stands as a cornerstone in the quest for efficient nuclear localization.
With these methods, researchers can quantify how strongly and specifically NLS interact with the Cas9 protein. For example:
- K_D (dissociation constant): A lower value indicates a high affinity, which is preferable for effective nuclear import.
- Thermodynamic profiles: Insights into enthalpy and entropy changes during binding provide a deeper understanding of the energetics involved in the interaction.
Employing such biophysical characterizations can help identify optimal NLS sequences that facilitate stronger and more discrete interactions with Cas9. Even subtle variations in NLS properties can lead to significant differences in overall gene-editing effectiveness. This careful, nuanced understanding is crucial, especially when designing novel modifications aimed at enhancing specificity or reducing off-target effects.
Applications in Genetic Engineering
The discourse around genetic engineering has taken a sharp turn with the advent of CRISPR technology. Central to this evolution is Cas9, a protein that acts like molecular scissors, allowing scientists to edit gene sequences with remarkable precision. Applications in genetic engineering extend far beyond simple modifications. They encompass a spectrum of precise interventions in crops, livestock, and human cells, pushing the boundaries of what was once thought possible in genetic manipulation.
CRISPR Technologies: Current and Emerging Applications
Currently, the applications of CRISPR-Cas9 technology are profound and varied. Scientists utilize this method across numerous fields, including agriculture, medicine, and environmental science. Some notable application areas include:
- Agricultural Innovation: Enhanced genetic traits in plants such as drought resistance and pest resilience. By employing CRISPR, researchers edit the plant genome for improved yield and sustainability, lessening the reliance on chemical pesticides.
- Medical Treatments: In humans, CRISPR is being explored as a revolutionary therapy for genetic diseases. Sickle cell anemia and cystic fibrosis are two conditions that scientists target with precision, making strides toward potential cures.
- Biotechnology Advances: The industrial sector benefits as CRISPR is employed to develop biofuels and bioplastics, thereby paving the way for sustainable methods of production that are less harmful to the environment.
Emerging applications are equally promising. Recent developments indicate potential uses in gene drives that could control pest populations or disease vectors like mosquitoes, ultimately leading to reduced incidence of malaria and dengue. Researchers are also probing into personalized medicine, utilizing CRISPR to tailor treatments based on individual genetic profiles.
NLS-Modified Cas9 in Therapeutic Contexts
The modification of Cas9 proteins to enhance their nuclear localization signals holds significant promise in therapeutic contexts. When we talk about NLS-modified Cas9, we refer to engineered versions of Cas9 that have been fine-tuned to improve their delivery efficiency into the cell nucleus, where the actual gene editing occurs.
- Enhanced Delivery: By adjusting nuclear localization signals, scientists can boost the likelihood that these modified Cas9 proteins reach their intended target sites within the nucleus. This potential increases both the efficacy of treatment and decreases the likelihood of off-target impacts.
- Targeting Precision: Improved NLS allows for more stringent control over where the edits occur within the genome. For instance, in research focused on hemophilia, NLS-modified Cas9 could direct genetic edits to the exact gene causing the disorder, limiting unintended modifications elsewhere.
- Therapeutic Potentials: This targeted approach manifests in therapies for genetic disorders, where precise changes are essential to ameliorate conditions without disrupting other essential functions in the genome.
âInnovations in NLS design are paving the way for transforming traditional gene therapy approaches into more effective solutions for complex genetic diseases.â
In summary, the applications of Cas9 in genetic engineering are transforming our understanding of genetics and opening doors to innovations that blend scientific inquiry with real-world solutions. This trajectory promises to redefine how we perceive challenges in genetics, providing a canvas for future exploration and application.
Challenges and Limitations of Cas9 NLS Utilization
When diving into the sea of genetic editing, particularly with CRISPR technologies, it's crucial to acknowledge that while Cas9 proteins and their nuclear localization signals (NLS) offer groundbreaking advancements, they come tethered to a few serious challenges and limitations. Understanding these hurdles not only aids researchers in harnessing this technology more effectively but also sets a realistic outlook on the future of gene editing.
Issues in Nuclear Localization
One of the most significant challenges associated with Cas9 is the efficiency and specificity of nuclear localization. The nuclear envelope can present quite a barrier. Factors affecting the transport of Cas9 into the nucleus include the size of the Cas9 protein itself, the specific cell type, and the conditions within that environment.
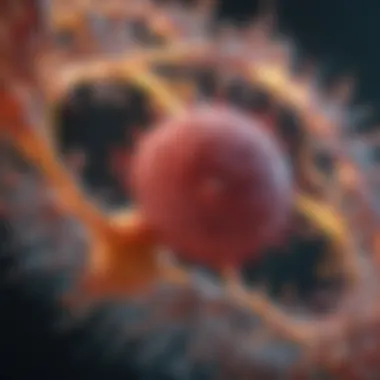
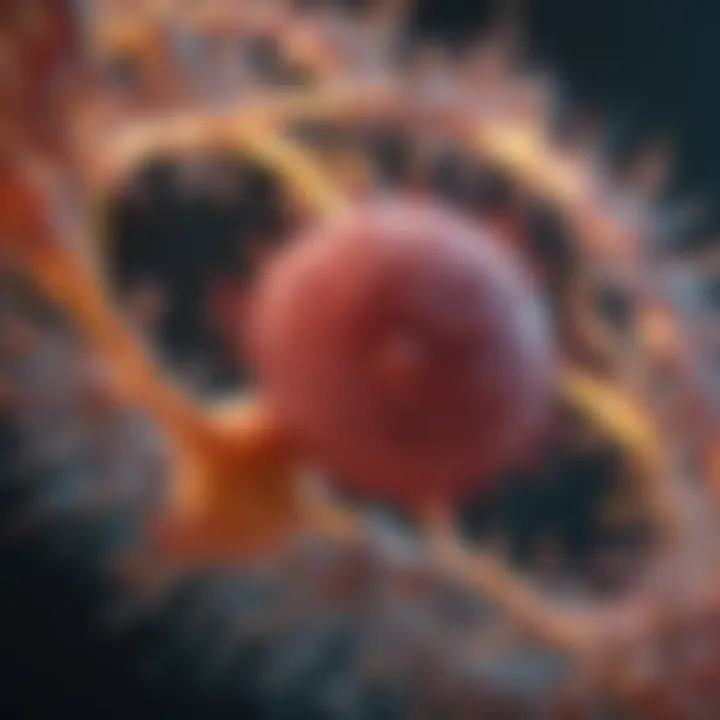
- Size Matters: Cas9, a relatively large protein, struggles with entry through nuclear pores, which are selective about what they allow through. If itâs too big or bulky, it may not pass as readily as smaller proteins.
- Cell Type Variance: Different cell types can express varying levels of transport proteins (called importins). In some scenarios, particularly in less common cell types, there may be an insufficient number of importins to facilitate efficient transport.
- Cellular Context: The cellular environment can also change the dynamics of nuclear import. Stress conditions or changes in cellular metabolism might affect the localization process, leading to variability in how well Cas9 reaches the nucleus.
These factors combined can lead to suboptimal delivery of Cas9 to its target, dampening the effectiveness of genetic alterations.
Potential Off-target Effects
The conversation surrounding Cas9's utility often brings up another cloudâpotential off-target effects. While the versatility of Cas9 in targeting specific DNA sequences is impressive, the risk of unintended modifications remains a considerable concern. It's a bit like throwing a dart; while you're aiming at the bullseye, it's all too easy to hit the wall.
- Mismatches and Mutations: Cas9 might bind to similar, but not identical, sequences within the genome, causing cuts in unintended locations, leading to mutations. These offtarget sites can potentially disrupt essential genes or regulatory elements, eliciting unforeseen consequences.
- Widespread Impact: Unlike targeted therapies that aim for a single genetic defect, Cas9 modifications can have broader impacts due to the overlapping nature of genetic sequences, particularly within complex genomes like human DNA.
- Evaluation Challenges: Detecting off-target events can be hugely complex. Traditional sequencing methods may not efficiently reveal all unintended edits, which complicates assessments of the safety and reliability of Cas9 applications.
In short, addressing the challenges of nuclear localization and the risk of off-target effects requires nuanced strategies and rigorous research to enhance the precision and safety of Cas9 utilization in genetic engineering.
Understanding the implications of Cas9's limitations is key to advancing efficient and safe genetic engineering practices.
Future Directions in Cas9 Research
The field of gene editing has taken a giant leap forward with the advent of Cas9 and its nuclear localization signals (NLS). As researchers grapple with existing challenges, the future promises a wealth of innovations. This section aims to highlight the crucial trends and innovations that can reshape how we approach genetic engineering, making significant strides in both precision and efficiency.
Innovations in NLS Engineering
The engineering of nuclear localization signals is gaining traction as a pivotal avenue for enhancing the efficiency of Cas9. Traditional NLSs are often not sufficient alone to ensure reliable nuclear delivery, given the myriad of cellular barriers. By customizing NLSs, researchers can fine-tune the nuclear entry process.
Some notable strategies being explored include:
- Synthetic NLS Creation: Tailoring new NLS sequences through synthetic biology approaches can lead to more effective transport mechanisms. This has seen earlier successes, particularly in the use of peptide designs which are optimized for specific cellular contexts.
- Fusion Proteins: By fusing Cas9 with known strong NLS from other proteins, such as SV40 large T antigen, scientists are leveraging existing robust transport experiences, resulting in heightened delivery efficiency to the cell nucleus.
- Utilization of Ligand-Triggered NLSs: Developing ligand-dependent nuclear localization strategies offers flexibility. This might allow for controlled delivery based on specific cellular conditions or signals.
These innovations promise not just improvements in delivery but also adaptations for specific applications, be it therapeutic use in humans or enhancements in plant genomic editing.
Predicted Trends in Gene Editing Technologies
As the research landscape continues to evolve, we can anticipate certain key trends shaping the future of gene editing technology:
- Global Collaboration: The rise of open-source platforms for genetic editing tools will likely encourage collaborative efforts across borders, enhancing collective problem-solving capabilities while inventing cutting-edge gene editing methodologies.
- Integration with Other Technologies: Combining CRISPR with emerging technologies such as artificial intelligence and machine learning can facilitate advancements in predictive modeling for off-target effects, enhancing precision in gene editing.
- Ethical Framework Development: As gene editing becomes more widespread, the need for comprehensive ethical guidelines will grow. Discussions around bioethics, especially concerning germline editing, could lead to a more standardized global approach, balancing innovation with safety and ethical practices.
"The future is not something we enter. The future is something we create."
These trends will necessitate ongoing research and discussion, forming the backbone of advancements in genetic engineering technologies as we strive to ensure responsible and effective applications of Cas9 and related tools. The incorporation of refined NLS strategies, building on these trends, holds substantial potential for the gene editing field.
Epilogue
The exploration of Cas9 and its nuclear localization signals (NLS) is not just an academic exercise. It holds paramount importance for understanding how we can harness gene editing technologies effectively. In this article, we've dissected various components associated with Cas9 and how NLS facilitates its movement into the nucleus, a vital step for its functionality in gene editing applications.
Recap of Key Insights
To summarize the key points:
- The role of Cas9 proteins is central to CRISPR technology, enabling accurate gene editing.
- Nuclear localization signals are essential for guiding Cas9 into the nucleus, making them critical for the success of gene editing workflows.
- Different types of NLS can dramatically influence the efficiency and precision of CRISPR applications, highlighting the importance of NLS engineering.
- Importins serve as crucial factors in the transport process, illustrating the complex interplay between Cas9 and cellular machineries.
These insights underscore that understanding these mechanisms is a launching pad into more sophisticated applications in genetic engineering.
Implications for Future Genetic Research
The implications for future research and applications derived from the insights discussed are significant:
- As we develop novel NLS modifications, it will lead to enhanced specificity and efficacy in gene editing. This could bridge critical gaps in genetic therapies, particularly for previously hard-to-target diseases.
- Innovations in NLS engineering may inform better therapeutic strategies, pushing the boundaries of synthetic biology and medicine.
- The understanding of NLS can foster collaborations between researchers from diverse fields, driving interdisciplinary approaches to solve complex genetic engineering problems.
"The future of gene editing will rely heavily on the precise interplay between molecular signals and cellular responses, as dictated by the innovative tweaking of mechanisms like NLS."