Assessing Energy Output of Wind Turbines
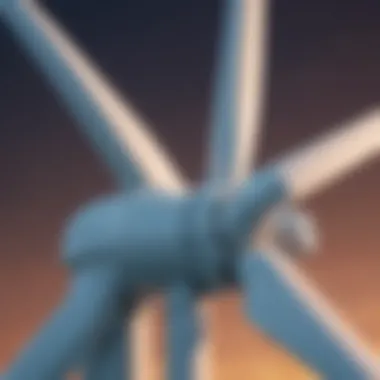
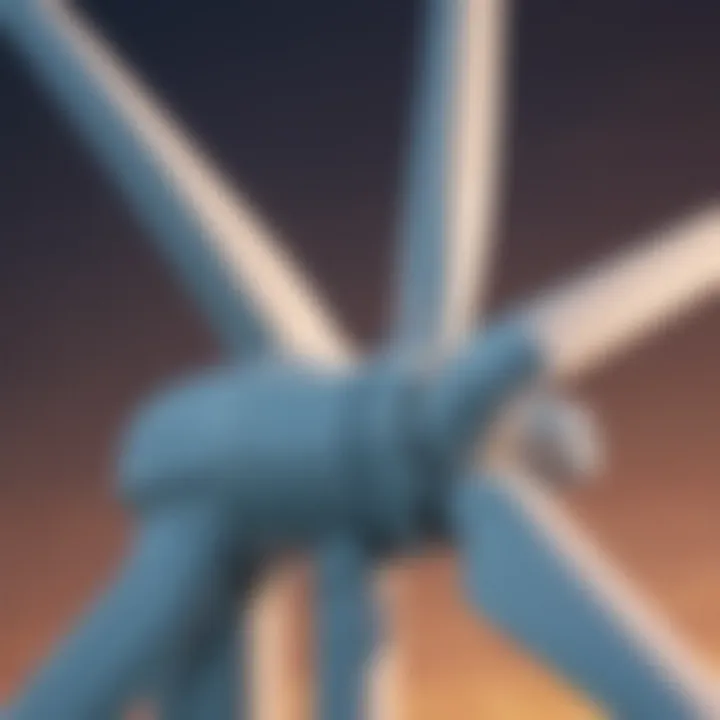
Intro
In recent years, wind energy has become a significant player in the global transition towards renewable energy sources. With growing concerns about climate change and the depletion of fossil fuels, the emphasis on harnessing natural energy has intensified. Wind turbines, as a principal technology in energy generation, offer a way to convert kinetic energy from wind into electricity. However, understanding the factors that influence their energy output is crucial.
Wind turbines are not merely mechanical devices; their efficiency and performance rely heavily on numerous variables. These include design specifications, geographical placement, and various operational conditions. Understanding these dynamics ensures maximum extraction of energy from wind resources, an aim that is central to both commercial and environmental objectives.
This article addresses the intricacies of wind turbine energy output assessment. By exploring related mathematical models, operational efficiencies, and comparing methodologies with other renewable sources, we aim to provide a comprehensive outlook on how wind energy impacts the broader landscape of sustainable practices.
In the sections that follow, we will delve deeper into the research findings that form the backbone of this analysis and the methodologies adopted to obtain them.
Intro to Wind Energy
Wind energy represents a crucial component of the modern energy landscape. As the world turns towards sustainable solutions to address climate change, understanding the essentials of wind energy becomes increasingly pertinent. This section introduces the foundational elements regarding the role of wind energy, offering insight into its historical context and current trends in production.
Wind energy is generated through wind turbines that convert the kinetic energy from wind into electrical power. This process not only diminishes reliance on fossil fuels but also contributes to a reduction in greenhouse gas emissions. As nations invest more in renewable resources, wind energy's importance as a cost-effective and environmentally-friendly solution cannot be overstated. A diverse energy portfolio including wind powers a more robust and resilient energy future.
Wind energy is particularly significant because it provides numerous advantages. It helps in:
- Reducing Carbon Footprint: Wind power generation produces no emissions during operation, thus promoting cleaner air and a healthier environment.
- Economic Development: The wind energy sector has stimulated job creation across various levels, from manufacturing to installation and maintenance.
- Energy Security: By diversifying the energy supply, countries can increase their energy independence and enhance security against market fluctuations in fossil fuel prices.
However, wind energy also presents challenges that need consideration, such as site selection, environmental impacts, and integration with existing grids. Understanding these factors is essential for maximizing energy output and achieving effective installations.
This article will explore these aspects in depth, starting with the historical context and culminate in the discussion of future directions in wind energy research.
Understanding Wind Turbine Mechanics
Understanding the mechanics of wind turbines is crucial for optimizing energy output. A wind turbine transforms kinetic energy from wind into mechanical energy, which can then be converted to electrical energy. This transformation involves various specific components and operational principles that dictate the efficiency and overall productivity of turbines. By comprehensively examining these elements, one can gain insights into improving turbine design and performance.
Components of a Wind Turbine
A wind turbine comprises several integral components that work together to harness wind energy. The primary parts include:
- Rotor Blades: These are aerodynamically designed to capture wind. The shape and length of the blades directly influence the amount of energy captured.
- Nacelle: This houses the generator and other critical components, such as the gearbox and braking system. It sits atop the tower.
- Tower: The height of the tower affects the wind speed experienced by the rotor blades. Taller towers can access winds that are more potent and consistent.
- Generator: Converts mechanical energy from the rotor into electrical energy. The efficiency of this conversion is vital for overall energy output.
- Control System: Monitors wind conditions and adjusts the turbine orientation, ensuring optimal wind capture and safety during extreme weather.
Each component plays a significant role, and understanding their functionalities helps in assessing how modifications or upgrades can enhance performance.
Operational Principles of Wind Turbines
The operational principles of wind turbines hinge on basic physics and engineering concepts. Key principles include:
- Lift and Drag: Wind turbines operate on the principles of lift and drag. Lift occurs when high-pressure air passes over the rotor blades, causing them to rotate. Drag can also affect performance, but optimal design seeks to maximize lift while minimizing drag.
- Power Coefficient: This is a measure of a turbine’s efficiency. It denotes the ratio of actual output power to the power available in the wind. Betz's limit states that no turbine can capture more than 59.3% of the kinetic energy in wind.
- Cut-in and Cut-out Speeds:
- Pitch Control: This is crucial for maintaining efficiency. It involves adjusting the angle of the blades to optimize performance based on changing wind conditions.
- Cut-in Speed: The minimum wind speed at which the turbine starts generating power, usually between 3 and 4 m/s.
- Cut-out Speed: The maximum wind speed where the turbine ceases operation to prevent damage, often around 25 m/s.
Understanding these operational principles is essential for improving turbine design and for optimizing placement in various environments. It guides engineers and designers in making informed decisions to enhance overall energy production.
"The efficiency of energy conversion in wind turbines largely relies on the seamless interaction of their components and a clear understanding of the operational principles."
Such insights into wind turbine mechanics not only contribute to the advancements in technology but also present pathways for research into more sustainable energy solutions.
Factors Affecting Energy Output
In the context of modern renewable energy, understanding factors that affect the energy output of wind turbines is crucial. Wind energy plays a significant role in reducing reliance on fossil fuels and minimizing greenhouse gas emissions. The efficiency and productivity of wind turbines depend on several elements, including physical design choices, local environmental conditions, and operational mechanics. Each factor interacts dynamically with the others, and a thorough assessment is essential for optimizing energy generation.
Wind Speed and Turbine Efficiency
Wind speed is perhaps the most critical component in determining a wind turbine's energy output. The relationship between wind speed and turbine efficiency is generally nonlinear, governed by power laws. As the wind velocity increases, the power generated by a turbine increases cubically, meaning even small increases in wind speed can result in significant boosts in electrical output.
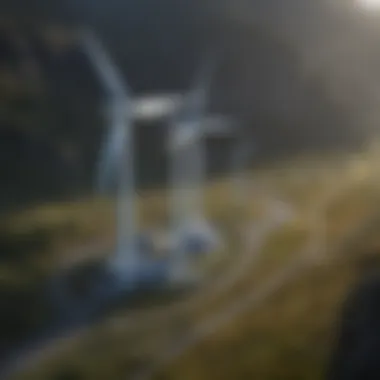
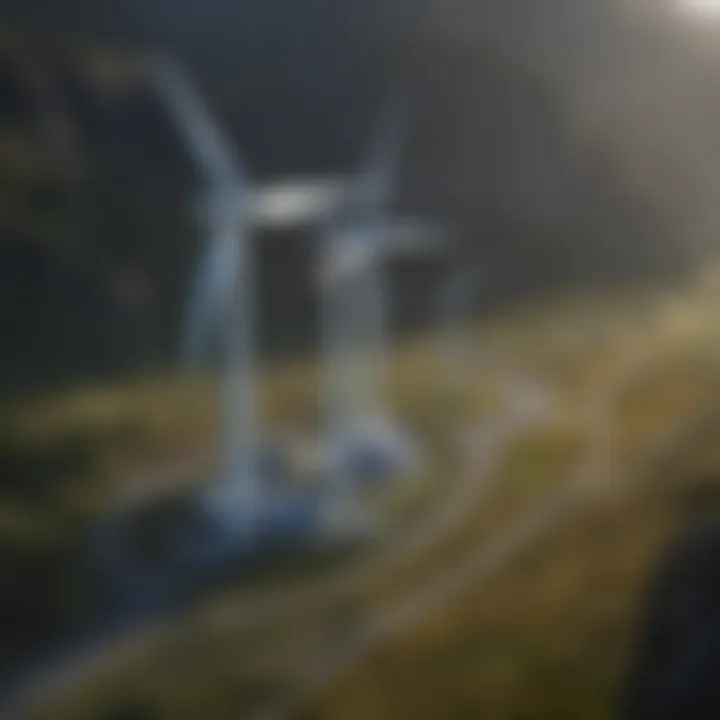
Critical to understanding this relationship is the concept of the cut-in speed, which is the minimum wind speed required for the turbine to start generating power. It often ranges between 3 to 4 meters per second. Conversely, turbines also have a cut-out speed, the maximum wind speed—usually around 25 meters per second—beyond which the turbine must shut down to avoid damage. This cut-off protects the turbine from excessive stress and blade fatigue.
"The power extracted from wind is significantly tied to the wind speed; optimizing the performance of a turbine involves ensuring it operates efficiently across varying wind conditions."
Turbine Design Variations
The design of a wind turbine can tremendously impact its energy output. Key design aspects include rotor diameter, blade pitch, and the hub height.
- Rotor Diameter: A larger rotor typically captures more wind, leading to higher energy outputs. Increasing rotor diameter improves the swept area, maximizing the wind energy harvested.
- Blade Pitch: Adjustable blades allow for changes in angle relative to the wind direction. This flexibility manages the turbine's energy capture at varying wind speeds, optimizing performance.
- Hub Height: Elevating the turbine allows for access to faster wind currents that are often found further above ground. Taller turbines can significantly enhance energy generation, especially in areas with variable wind conditions.
Ultimately, minute alterations in these designs can culminate in dramatic differences in output efficiency.
Environmental Influences
Environmental influences encompass more than just wind speed; they include local climatic conditions such as temperature, humidity, and turbulence.
- Temperature: Temperature affects air density. As temperature rises, air becomes less dense, which can lead to a reduction in energy production. Turbines must be engineered to accommodate temperature fluctuations to maintain optimal performance.
- Humidity: High humidity can also impact turbine performance by introducing additional weight to the rotor blades. This can alter aerodynamic efficiency and in some scenarios, potentially affect overall energy output.
- Turbulence: It is crucial to analyze local topography as it influences wind patterns. Areas with significant turbulence can reduce turbine performance and lead to increased wear on machinery.
Following a thorough evaluation of these environmental aspects, site-specific considerations can further enhance energy predictions for wind farms.
Mathematical Models for Energy Output
Mathematical models are crucial in the assessment of energy output from wind turbines. They serve multiple functions in analyzing how effectively turbines convert wind into usable energy. By utilizing mathematical approaches, engineers and researchers can predict performance, optimize designs, and evaluate potential energy yields at specific sites. The correct model allows for a clearer understanding of factors such as wind speed, air density, and turbine characteristics, guiding decision-making in both the development and operation of wind farms.
Ultimately, engaging with these models also provides a framework for policymakers and investors to make informed decisions regarding the viability and sustainability of wind energy projects. A robust model can minimize risks, enhance financial assessments, and contribute to achieving renewable energy goals.
Power Coefficient and Betz's Limit
The power coefficient is a key parameter that defines the efficiency of a wind turbine in converting kinetic energy from wind into electrical energy. This coefficient ranges from 0 to 1, where a value of 1 represents total conversion. However, according to Betz's limit, a theoretical maximum of 0.593 can be achieved, meaning no turbine can exceed this efficiency.
Betz's limit was derived based on fluid dynamics principles, demonstrating that a turbine can only extract a fraction of the wind's energy while allowing enough airflow to sustain the motion of wind. This conceptual framework helps to gauge the potential output any designed turbine could produce under optimal conditions. Thus, understanding the bounds set by Betz's limit is essential for engineers when designing efficient turbines that strive to maximize energy output.
Calculating Energy Yield
Calculating energy yield is an essential step in assessing wind turbine performance. This process involves mathematical expressions that consider factors like average wind speed, turbine capacity, and operational time. The general formula for energy yield can be expressed as:
[ E = P * T ]
Where:
( E ) = Energy produced (kWh)
( P ) = Average power output (kW)
( T ) = Time (hours)
The average power output can further be calculated using the swept area of the turbine's rotor and the wind speed:
[ P = \frac12 * C_p * \rho * A * v^3 ]
Here,
( C_p ) is the power coefficient,
( \rho ) is air density,
( A ) is the rotor area, and
( v ) is wind speed.
Such calculations help stakeholders understand the expected energy generation over time, facilitating more accurate projections when planning and evaluating wind energy projects.
Challenges in Predictive Modeling
Predictive modeling in wind energy is not without its challenges. Various unpredictable variables significantly influence model accuracy and reliability:
- Wind Variability: Wind is inherently unpredictable. Sudden changes in speed or direction can lead to discrepancies between predicted and actual output.
- Environmental Factors: Terrain, vegetation, and proximity to structures can affect wind patterns, complicating model forecasts.
- Technological Limitations: Models must rely on available data, which can be limited or of varying quality. Enhancing data collection methods is crucial for better predictive accuracy.
"Accurate predictive models are vital for realizing the full potential of wind energy."
Overcoming these challenges requires continuous refinement of modeling techniques and learning from operational data. An iterative approach aids in updating the models to reflect real-world complexities effectively.
Site Assessment and Turbine Placement
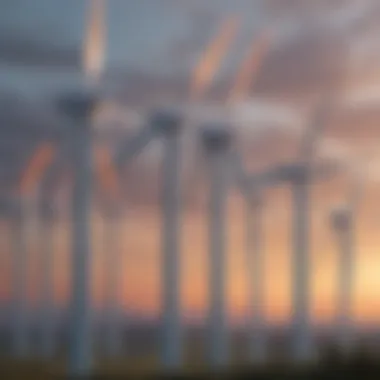
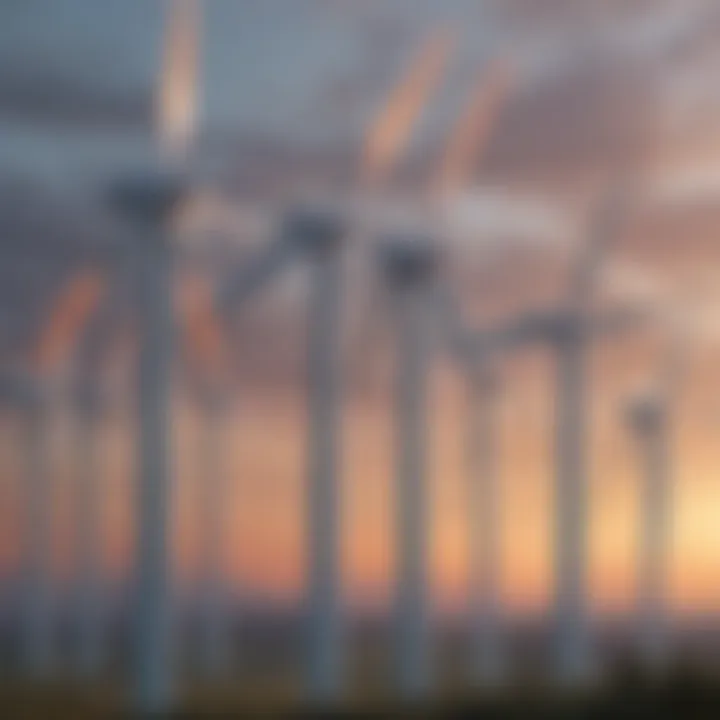
The process of site assessment and turbine placement is crucial for optimizing the energy output of wind turbines. A thorough evaluation of the site ensures that the installation will be effective, efficient, and sustainable over time. This phase encompasses multiple elements including wind resource assessment, land use considerations, and the environmental impact of placing turbines in specific areas. Proper site selection can significantly improve the energy yield and financial viability of wind energy projects.
Wind Resource Assessment
Wind resource assessment involves measuring and analyzing wind patterns in a potential installation area. This step is essential because the quality of wind directly influences the turbine's performance. The assessment typically includes:
- Anemometer Measurements: These devices measure wind speed and direction over a period of time, usually spanning several months to a year. This data helps project the expected energy production.
- Wind Maps: These graphical representations provide insight into the distribution of wind resources across larger geographical areas. They are useful for identifying potential sites that may have favorable conditions.
- Long-Term Data Analysis: Historical wind data can highlight trends over time, assisting in understanding seasonal variations. This further informs decisions regarding turbine design and operational strategies.
Accurate wind resource assessment is critical. It can determine the effectiveness of a wind farm, influencing both initial investment and ongoing operational costs. Once collected, this data enables engineers to select suitable turbine models and make informed decisions on placement.
Land Use and Spatial Considerations
Land use and spatial considerations refer to the evaluation of land where turbines might be placed. Understanding the surrounding environment and land availability is vital for any wind power project. Several factors come into play in this assessment:
- Zoning Laws and Regulations: Local regulations may restrict turbine placement, requiring thorough knowledge of land use policies. Understanding these rules ensures compliance and prevents costly delays.
- Environmental Impact: The potential impact on local wildlife, natural habitats, and ecosystems must be assessed. Certain areas may be more sensitive, necessitating careful planning or alternative site selections.
- Proximity to Infrastructure: The distance to necessary infrastructure such as power lines and roads should be evaluated. Closer access can reduce costs related to installation and maintenance.
- Community Considerations: Engaging with local communities can help mitigate opposition and gather support. The social perception of wind energy projects can influence their success significantly.
By addressing these land use and spatial considerations, stakeholders can better plan for long-term sustainability and energy output maximization.
"Effective site assessment lays the foundation for successful wind energy projects. Skipping this crucial phase can lead to operational inefficiencies and reduced returns on investment."
The assessments conducted in this phase serve to inform stakeholders about critical decision-making factors, leading to more informed and strategic turbine placement.
Case Studies in Energy Output
The exploration of case studies in energy output is essential to understanding wind turbine performance in real-world conditions. By analyzing various projects, we gain valuable insights into the operational efficiency of wind turbines. Case studies provide detailed examples of successful implementations and highlight the challenges faced in different environments. These narratives serve as insightful lessons that contribute to future innovations in wind energy technology. By examining both successes and failures, we can develop a clearer picture of the factors that affect energy output.
Analyzing Successful Installations
Successful installations of wind turbines offer critical lessons in optimizing energy output. Each project provides a context in which certain design features and operational strategies have proven effective. For example, the Hornsea One offshore wind farm in the United Kingdom stands as a remarkable installation. With a capacity of 1.2 gigawatts, it has demonstrated how large-scale projects can capitalize on strong wind resources.
In Hornsea One, careful site assessment played a crucial role. The extensive studies allowed developers to identify the wind profile and select suitable turbine models, ensuring efficiency. Moreover, effective grid integration helped maximize the energy extracted. This case demonstrates that thorough planning and assessment can enhance energy yields significantly.
"Real-world installations like Hornsea One illustrate the importance of site assessment in optimizing turbine performance."
Additional cases, such as the Gansu Wind Farm in China, showcase how strategic investment in technology and infrastructure can lead to success. With a cumulative capacity of over 7 gigawatts, it highlights the benefits of scaling and investing in advanced turbine technologies.
Collectively, these examples underscore the need for meticulous planning, robust technology, and flexibility in implementation to achieve favorable outcomes in energy production.
Failures and Lessons Learned
Failures within wind turbine projects also provide invaluable insights into the complexities of energy output. Examining missteps can reveal broader trends that may not be immediately obvious. Consider, for example, the development of the Cape Wind project in Massachusetts. Initially touted as a pioneer in offshore wind energy, the project faced numerous challenges, including regulatory delays and opposition from local stakeholders. Ultimately, the project was scrapped, highlighting the significance of community engagement and regulatory navigation.
Lessons from Cape Wind emphasize the importance of not only technical feasibility but also sociopolitical factors. Understanding public perception and ensuring stakeholder buy-in can make the difference between a project’s success and its failure.
Another case is the failure of the Alta Wind Energy Center in California due to issues with grid connectivity. Although the turbines operated effectively, the energy produced could not be efficiently transmitted to consumers. This case illustrates the need for integrated planning that includes energy distribution infrastructure alongside turbine installations.
Environmental and Economic Impacts
The intersection of environmental and economic impacts is crucial in understanding the full implications of wind energy. Wind energy presents an opportunity to mitigate climate change, reduce air pollution, and provide sustainable energy. Evaluating its environmental benefits alongside economic viability offers a holistic view of its role in the global energy landscape.
Carbon Footprint Analysis
Wind turbines operate without emitting greenhouse gases during their electricity generation phase. However, a thorough carbon footprint analysis encompasses not only the operation but also the manufacturing, installation, and decommissioning stages.
- Manufacturing emissions: The production of components like blades, towers, and gear systems requires energy, which often comes from fossil fuels. The material choices, such as steel and concrete, also contribute significantly to emissions.
- Transport and Installation: Transporting large turbine components to installation sites involves energy consumption, typically from diesel-powered vehicles. This section of the lifecycle also adds to the overall carbon footprint.
- Lifecycle assessment: Evaluating the entire lifecycle of a wind turbine can show a significant reduction in emissions compared to fossil fuel energy production. Various studies suggest that wind energy has a substantially lower carbon footprint per unit of electricity generated.
By assessing both the direct and indirect emissions associated with wind energy, stakeholders can better understand the potential of wind turbines in combating climate change and improving air quality.
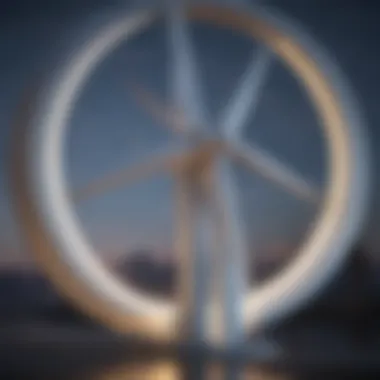
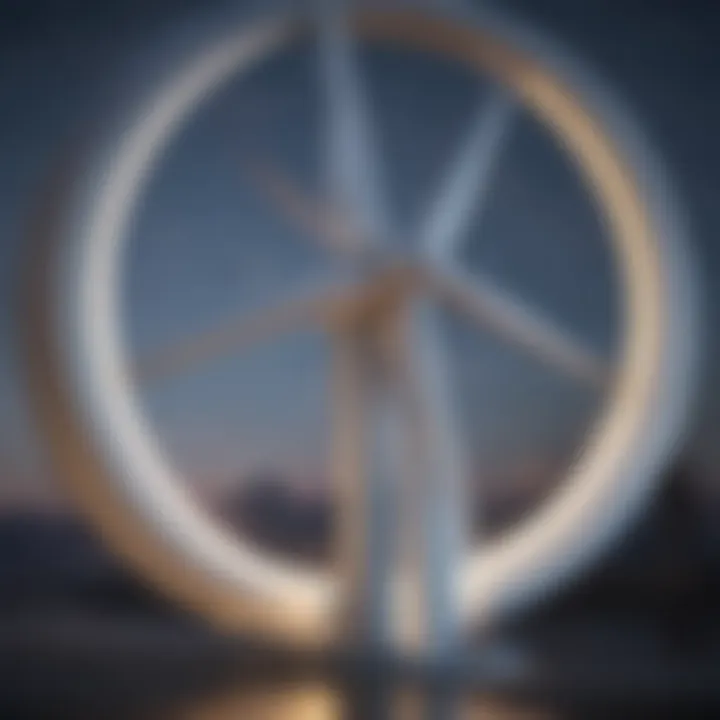
"The shift towards wind energy solutions represents a critical action in the global effort to reduce carbon emissions and limit temperature rise."
Economic Viability of Wind Energy
The economic viability of wind energy is influenced by several key factors, including initial investment, operational costs, and market dynamics.
- Initial Investment: The upfront costs of wind turbine installation are significant. These costs include purchasing the turbines, constructing infrastructure, and connecting to the grid. Financial incentives may mitigate some of these expenses, making investments more attractive.
- Operational Costs: Once installed, wind turbines have relatively low operational costs. Maintenance is generally uncomplicated but must be budgeted to ensure efficiency and longevity.
- Power Purchase Agreements (PPAs): Long-term agreements with utilities can ensure stable revenue for wind energy producers, enhancing the project's economic stability. These contracts guarantee a fixed price for energy, safeguarding against fluctuating market prices.
- Market Competitiveness: As technology advances and efficiencies improve, the cost of wind energy continues to decline. This enhances its competitiveness with traditional energy sources. The falling price of wind-generated electricity makes it an increasingly attractive option for energy generation.
Understanding the economic implications protects investor interests and promotes further development in the wind energy sector. Overall, when balanced against the environmental benefits, wind energy emerges as a promising candidate for sustainable power generation.
Comparative Analysis with Other Renewable Sources
In discussing energy generation, assessing wind turbines involves a necessary evaluation against other renewable sources. This comparative analysis is vital for understanding the relative efficacy, efficiency, and potential of wind energy in the broader context of sustainable energy solutions. Key aspects include cost-effectiveness, environmental impact, and the reliability of energy production.
Wind vs. Solar Energy
Wind energy and solar energy are two predominant players in the search for renewable solutions. These sources present unique advantages and disadvantages.
- Resource Availability: Wind energy generation is highly dependent on wind patterns, which can vary significantly by location. In contrast, solar energy relies on sunlight, which can be more predictable in certain climates, yet it is limited to daylight hours.
- Output Versatility: Wind turbines tend to generate electricity at higher capacity and can operate at night, unlike solar panels, which only produce energy during the day. However, solar panels can be more effective in distributed applications given their smaller footprint.
- Investment and Maintenance: Initial installation costs for solar panels may be lower, but wind farms can yield more electricity over their lifespan. The maintenance costs for wind turbines are usually higher due to moving parts, yet they often produce higher energy outputs.
These considerations shape the decision-making for energy providers and policymakers. Integrating both technologies could maximize energy production by leveraging their complementary characteristics.
Integration with Hydro and Biomass
Combining wind energy with hydro and biomass presents numerous benefits that improve the overall energy output and reliability. Each of these sources complements the other in different ways.
- Hydropower Synergy: Studies show that integrating wind energy with hydroelectric systems can create a more stable energy supply. During periods of high wind generation, excess electricity can be used to pump water back into reservoirs. Conversely, when wind generation decreases, hydro plants can quickly ramp up production, maintaining grid stability.
- Biomass Flexibility: Biomass energy provides a flexible backup energy source. It can generate power when wind conditions are unfavorable. This can help mitigate the intermittency issues associated with wind energy, ultimately leading to a more resilient energy grid.
- Environmental Considerations: The combination of these three energy sources can reduce carbon emissions and create sustainable waste management solutions. Utilizing byproducts from biomass can contribute to a circular economy, while hydropower supports flood control and irrigation.
Combining these renewable sources not only enhances energy reliability but also opens pathways for innovative energy strategies. The optimal mix of wind, hydro, and biomass can yield a more sustainable energy framework.
"The future of energy generation lies in diversification and integration of renewable sources. Each component offers strengths that can be harnessed collaboratively to create a robust energy landscape."
Ultimately, a careful assessment of wind turbines relative to other renewable sources provides a clearer appreciation for their role and potential in achieving global energy goals.
Future Directions in Wind Energy Research
The evolution of wind energy is essential for advancing renewable energy solutions globally. As technology progresses, expectations for efficiency and energy output rise. Understanding future directions in wind energy research is crucial for students, researchers, educators, and professionals alike. It encapsulates innovations that promise to redefine energy generation, along with relevant policy implications that may affect these innovations. This section will delve into key trends in emerging turbine technology and the regulatory frameworks that will shape the landscape of wind energy.
Innovations in Turbine Technology
Recent advancements in turbine technology aim to enhance the efficiency of energy conversion. Innovations such as vertical axis wind turbines and floating wind farms are gaining traction. These designs offer advantages in specific environments over traditional horizontal axis turbines.
- Vertical Axis Wind Turbines: These turbines perform well in turbulent wind conditions and occupy less surface area. Their design allows for easier maintenance since the generator can be placed closer to the ground.
- Floating Wind Farms: Utilizing advances in buoyancy and anchoring, floating wind installations allow development in deeper waters where wind resources are often better. This opens up new hotspots for energy capture, reducing competition for surface space with fishing and shipping industries.
"Innovations in turbine technology not only increase efficiency but also improve environmental compatibility, making wind energy an attractive choice for new regions."
Moreover, advancements in materials science enhance the durability and lifespan of turbine components. The introduction of lighter, stronger materials can result in higher energy output while also reducing maintenance costs. This is critical for the financial viability of large-scale wind projects.
Policy and Regulatory Developments
Effective policy and regulation play a vital role in shaping the wind energy sector. Government support through incentives encourages investment in new technologies. Moreover, stringent environmental regulations must also be considered to ensure sustainability.
- Incentives and Subsidies: Financial support from governments can catalyze the development of wind projects. This includes tax incentives for renewable energy investments and grants for research and development.
- Environmental Regulations: Balancing wind energy growth with ecological protection is essential. Policymakers need to consider the impact of wind farms on local wildlife and habitats. Research into minimizing these negative externalities is increasing.
Furthermore, international collaborations can vastly enhance research efforts. Sharing findings and best practices can lead to technological leaps and improved regulatory frameworks across countries. As nations strive to meet renewable energy targets, collaborating on policies may foster a conducive environment for wind energy’s expansion.
In summary, the future directions in wind energy research highlight a promising trajectory. Innovations in turbine technology are enhancing capabilities, while progressive policies are essential for fostering growth. It is imperative to continue monitoring these developments for their potential impacts on sustainable energy practices.
End
The conclusion of this article emphasizes the significance of understanding energy output in wind turbines. Throughout our exploration, we have highlighted how various factors such as turbine design, location, and operational environment critically influence energy generation. The conclusions drawn here not only reinforce the technical aspects covered earlier but also illuminate the broader implications for sustainable energy practices.
A key point to note is the importance of assessing energy output accurately. This process informs decision making at multiple levels—from selecting appropriate turbine technology to site assessments that optimize resource utilization. Without an in-depth understanding of how these factors interact, projects may face pitfalls that not only reduce efficiency but could also have economic ramifications.
Moreover, as wind energy continues to grow in importance globally, the demand for precise models and methodologies becomes vital. The success of wind projects hinges on our ability to predict outputs, manage expectations, and ensure efficient designs.