Exon Sequencing: Comprehensive Insights and Applications
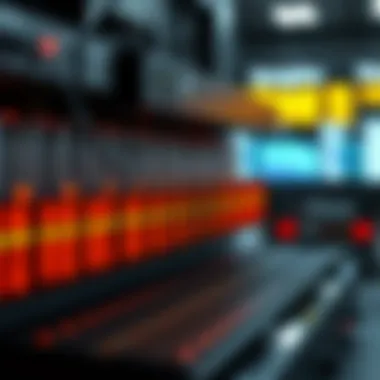
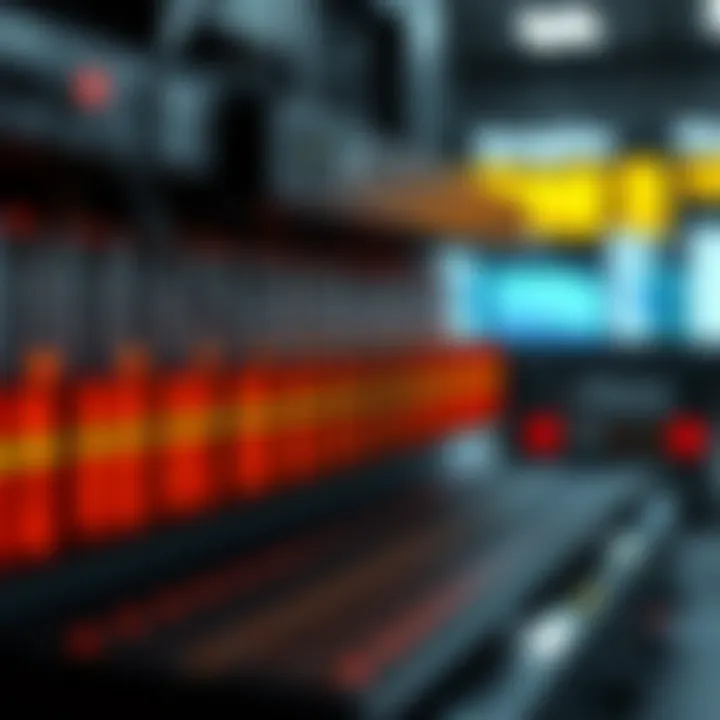
Intro
Exon sequencing stands at the intersection of genetics and clinical diagnostics, one might say it's like the detective work of the genetic world. Every bit of information secured can unveil significant insights into how genes express themselves and where variations occur.
As we dive into this topic, consider the sheer scale of what is being analyzed. The human genome comprises roughly 20,000-25,000 genes, with exons – the regions that code for proteins – playing a crucial role. Understanding these segments not only enhances our grasp of fundamental biology but also opens doors to evolving applications in medicine.
The significance of exon sequencing cannot be overstated. It serves as a cornerstone in disciplines such as cancer research, unraveling the genetic underpinnings of various malignancies, and in the realm of personalized medicine, where tailored therapy can significantly impact patient outcomes. The implications of this analysis extend beyond academic curiosity; they resonate in real-world applications.
In the following sections of this comprehensive review, we will elucidate methodologies, highlight key findings, and consider challenges in this rapidly evolving field. From the nuts and bolts of how exon sequencing functions to its broader implications, our aim is to provide a well-rounded discussion that caters to an audience with a vast array of interests and expertise. Let's embark on this journey through the intricate landscape of exon sequencing.
Prelude to Exon Sequencing
Exon sequencing is rapidly becoming a linchpin of genetic research and clinical diagnostics, as it offers crucial insights into the complex world of gene expression and variation. By focusing on the protein-coding regions of the genome, exon sequencing enables researchers to pinpoint mutations that could influence health and disease. This methodology's significance extends beyond mere academic curiosity; it has real-world implications for understanding genetic diseases, personalizing treatment strategies, and unraveling the genetic underpinnings of various ailments. Essentially, it transforms the way researchers and practitioners approach genomic data, prioritizing immediate and actionable insights.
Definition and Importance
Exon sequencing specifically targets the exons, which are segments of DNA that encode proteins, making up roughly 1-2% of the human genome but harboring a substantial number of clinically relevant variants. These variants can lead to rare inherited disorders or contribute to complex diseases such as cancer. By isolating and analyzing these regions, exon sequencing facilitates the identification of pathogenic mutations, thereby serving as a critical tool in diagnostic laboratories.
This targeted approach not only aids in genetic disease identification but also supports the expanding field of pharmacogenomics. Understanding a patient’s genetic makeup allows clinicians to tailor treatment plans, ensuring higher efficacy and reduced adverse effects. The ability to link genotype to phenotype through robust exon sequencing processes is invaluable in today's personalized medicine landscape, as it could potentially reshape therapeutic approaches for a myriad of health conditions.
Historical Context
Delving into the historical backdrop of exon sequencing reveals a trajectory of discovery and technological innovation. The roots can be traced back to the sequencing of DNA itself; notable milestones include the introduction of Sanger sequencing in the 1970s, which laid the groundwork for future methodologies. As researchers delved deeper into genetics, the Human Genome Project emerged in the 1990s, which propelled the exploration of genomic sequences, creating a renewed focus on exons.
Over the years, advancements in sequencing technologies yielded a diverse array of methodologies, leading to the birth of Next-Generation Sequencing (NGS) in the 2000s. This not only drastically reduced sequencing costs but also increased throughput, making it feasible to conduct large-scale studies focusing on exons. The gradual evolution of these techniques reflects how exon sequencing occupies a pivotal position in both basic research and clinical settings—just as adaptable as it is essential. The strides made in understanding exons continue to shape our approach to genetics, pushing the boundaries of what is possible in both research labs and hospitals.
The Basics of Exon Sequencing Techniques
Exon sequencing stands as a pivotal technique within the realm of genetics. It enables researchers to focus on the coding regions of the genome, making it indispensable for various applications, including disease research and personalized medicine. Understanding the fundamentals of exon sequencing techniques is critical. Through these techniques, scientists glean insights that lead to advancements in diagnosing and treating genetic disorders. This section will unravel the core methodologies underpinning exon sequencing and offer a comparative overview of the two primary techniques: Next-Generation Sequencing and Sanger Sequencing.
Comparative Overview
When it comes to exon sequencing, the choice of technique can significantly influence the outcomes of a study. Both Next-Generation Sequencing (NGS) and Sanger Sequencing have their unique strengths and applications. NGS is like the new kid on the block, having dramatically changed the landscape of genomic research. On the other hand, Sanger Sequencing, though older, remains a reliable method that has stood the test of time. Each approach brings with it its own set of benefits, limitations, and contexts in which it excels. To summarize:
- Depth of sequencing: NGS can analyze millions of fragments simultaneously, while Sanger is suited for targeted approaches.
- Cost and time efficiency: NGS has lowered costs per base, yet Sanger provides faster results for smaller sets of data.
- Accuracy and error rates: Sanger is considered gold standard for accuracy, even if NGS improves over time.
Next-Generation Sequencing
Next-Generation Sequencing has revolutionized the way scientists approach genetics. One of its most essential features is its ability to sequence entire exons rapidly and cost-effectively. Unlike traditional methods, NGS can generate vast amounts of data in a single run, enabling comprehensive analyses previously beyond reach.
Key Features
The hallmark of Next-Generation Sequencing lies in its scalability and efficiency. It functions by breaking down the DNA into smaller fragments and simultaneously sequencing these fragments. This parallel processing accelerates data collection, offering an expansive overview of gene variants, which is crucial for understanding complex genetic diseases. Its ability to identify rare mutations makes NGS incredibly valuable in precision medicine and research.
Unique feature: Real-time analysis during sequencing allows researchers to adapt their strategies on-the-fly. This flexibility is a game-changer in ongoing projects where new findings can reshape research focus almost instantaneously.
Advantages Over Traditional Methods
One significant advantage of NGS is its high throughput capability. In one sequencing run, it can analyze thousands of genes at once, making it a cost-effective method for large-scale studies. This factor is particularly beneficial for teams investigating hereditary conditions, cancer mutations, or other complex traits.
Unique feature: NGS results in a higher resolution of variant detection as well. It not only identifies single nucleotide polymorphisms but also larger structural variations, contributing to a more comprehensive understanding of genomic landscapes.
Sanger Sequencing
Sanger Sequencing has been a foundational technique in genetics since its introduction. Although newer methods have taken the limelight, Sanger continues to hold value, especially in situations requiring high accuracy.
Procedure
The Sanger method involves amplifying specific DNA segments and then incorporating labeled nucleotides during the sequencing process. This sequencing method is straightforward and delivers reliable results for targeted sequences. Compared to NGS, Sanger allows researchers to confirm findings from large-scale studies with precise resolution.
Unique feature: Its simplicity and low error rate make it ideal for validating findings from Next-Generation Sequencing, especially in clinical settings where precision is critical.
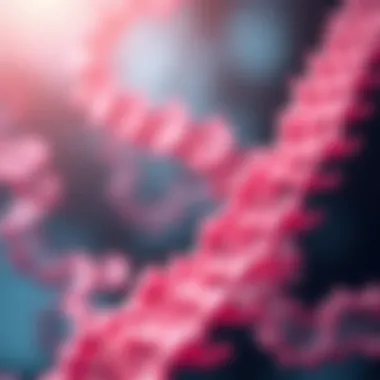
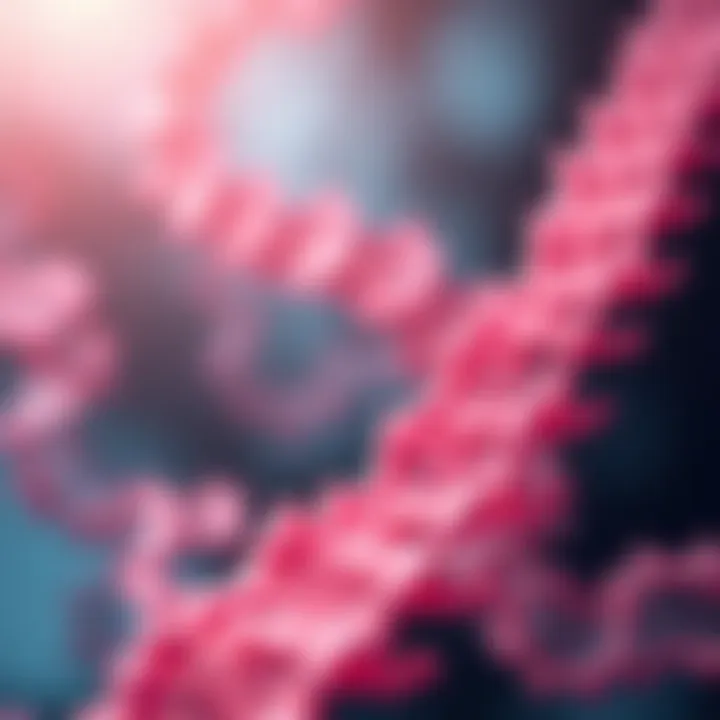
Limitations and Applications
While Sanger Sequencing excels in accuracy, it's not the most efficient for extensive genomic studies. This method is labor-intensive, making it costly for large-scale applications. However, it’s extremely useful in clinical diagnostics, particularly for confirming variants discovered by NGS.
Unique feature: Sanger Sequencing serves as a solid safety net, ensuring that critical mutations are accurately documented before embarking on treatment plans or additional interventions.
In summary, understanding the basic techniques of exon sequencing, including the functionalities and applications of Next-Generation and Sanger Sequencing, provides invaluable context for researchers aiming to harness these methodologies for advancing genetic discoveries.
Applications of Exon Sequencing
Exon sequencing plays a pivotal role in the modern understanding of genetics. Its ability to isolate and examine the expressed parts of genes offers valuable insights across various fields, particularly in clinical diagnostics and genomics research. In this section, we delve into the significant applications of exon sequencing, highlighting genetic disease identification, cancer genomics, and pharmacogenomics, all of which demonstrate how this technology can lead to innovative breakthroughs in these areas.
Genetic Disease Identification
Rare Genetic Disorders
Rare genetic disorders often slip through the cracks of traditional diagnostic methods, leaving patients and families in the lurch. Exon sequencing brings these elusive conditions into the spotlight by enabling the identification of pathogenic variants quickly and precisely. A key characteristic of rare genetic disorders is their low prevalence, sometimes affecting only a handful of individuals worldwide. This makes pinpointing the underlying genetic cause even more critical, as it opens doors to potential treatments and therapies specific to the individual's genetic makeup.
The beneficial aspect of focusing on rare genetic disorders is that exon sequencing can reduce the time and complexity often associated with multi-gene tests. Its targeted approach allows researchers to zero in on specific genetic areas, avoiding the fluff that can often come with broader screenings. However, one downside is the cost; while exon sequencing is a step forward, it can still be expensive for families, particularly those without robust insurance coverage.
Carrier Screening
Carrier screening is another crucial application where exon sequencing shines. It helps individuals and couples understand their genetic compatibility and risk for passing on hereditary conditions. A notable characteristic of carrier screening is its emphasis on preconception testing, where prospective parents can take proactive steps based on their genetic backgrounds.
For this article, the focus on carrier screening is beneficial because it not only raises awareness of potential hereditary risks but also facilitates informed reproductive choices. The unique feature here is the chance to identify carriers of mutations that could lead to serious conditions in offspring, such as cystic fibrosis or sickle cell disease. Yet, the process is not without challenges; ethical dilemmas about what to do with the information can arise, especially if parents discover they are carriers of severe conditions.
Cancer Genomics
Mutation Detection
The landscape of cancer diagnosis and treatment is rapidly evolving, with exon sequencing leading the charge in mutation detection. In cancer genomics, the identification of specific mutational changes in exons can significantly impact patient management strategies. A vital part of mutation detection is its ability to reveal actionable mutations that drive cancer progression, often making the difference between a standard and a targeted treatment approach.
For our audience, the attractiveness of mutation detection lies in its promise for precision medicine. By identifying mutations specific to a tumor, oncologists can tailor treatment plans that directly target those modifications. However, one caveat is that not all mutations can be effectively targeted by therapies at this stage, and the continued development of targeted treatments is essential.
Therapeutic Target Identification
Therapeutic target identification is seamlessly linked with mutation detection and forms a cornerstone of personalized cancer therapy. The aim here is to find specific molecular targets that can be acted upon by new drugs. A key feature of therapeutic target identification is how it leverages insights from genetic sequencing to unearth the best candidates for targeted treatment.
This aspect of exon sequencing is popular in the oncology field because it shifts the approach from generalized treatments to individualized regimens. The advantage here is the potential for increased efficacy of treatment while minimizing side effects associated with conventional therapies. Yet, it is crucial to note that the current understanding of many targets is still in its infancy, and ongoing research is needed to unlock the full potential of this approach.
Pharmacogenomics
Personalized Treatment Plans
Personalized treatment plans represent a cutting-edge application of pharmacogenomics using exon sequencing. This branch of genomics seeks to tailor medication and treatment strategies based on the individual genetic makeup of each patient. A notable characteristic of personalized treatment plans is their aim to improve drug efficacy and reduce adverse drug reactions.
This approach is particularly beneficial in an era where trial-and-error methods often undermine patient care. With detailed insights into how a patient's unique genetic profile may influence drug metabolism, healthcare providers can make more informed decisions. One limitation, though, is the variability in how different populations respond to drugs, making it a complex field requiring careful consideration and research.
Drug Response Prediction
Drug response prediction is closely tied to personalized treatment plans, as it helps anticipate how different patients will react to specific medications. By analyzing exons, pharmacogenomic profiling enables clinicians to select drugs that are more likely to be effective based on the existing genetic markers. The key characteristic here is precision; drug response prediction aims to mitigate the trial-and-error approach that has long been the norm in pharmacotherapy.
The compelling aspect of drug response prediction for our discussion lies in its ability to enhance treatment outcomes across diverse patient groups. However, this is not without its challenges. The predictive models are still evolving and may not capture the complete picture, requiring ongoing validation in clinical settings to ensure that predictions hold true in practice.
Methodological Considerations in Exon Sequencing
In the field of exon sequencing, methodological considerations are crucial. They influence not just the outcomes of each project, but also the reliability and applicability of the findings. Understanding the intricacies involved in sample preparation, data analysis, and quality control can notably enhance the overall quality and impact of exon sequencing studies.
Sample Preparation
Source Material
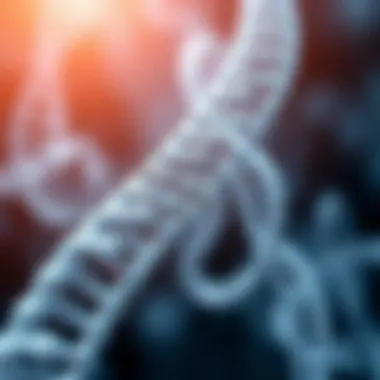
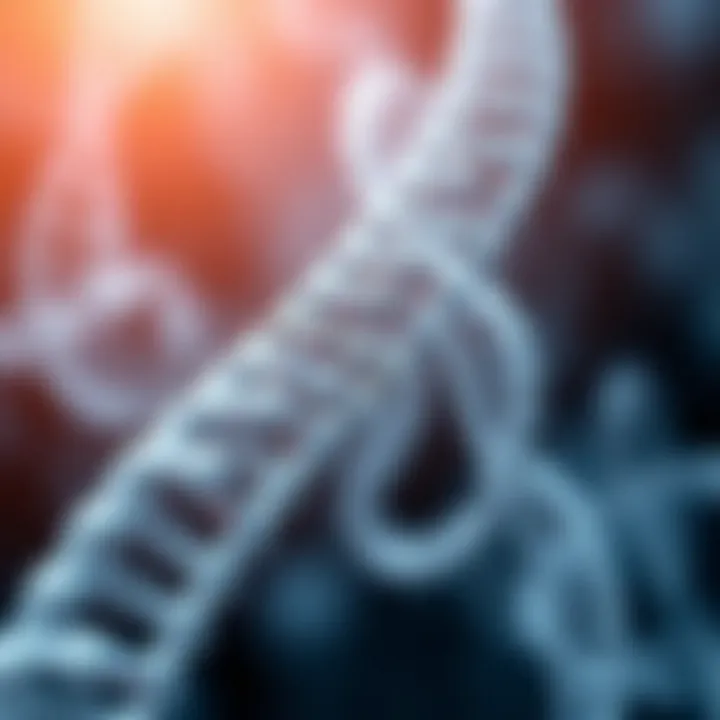
When it comes to source material, the origin of biological samples is vital. Commonly, blood, saliva, and even tumor tissues serve as the foundation for extracting the nucleic acid needed for sequencing. Each type possesses unique characteristics that affect downstream applications. For instance, blood samples are a popular choice because they can be collected with minimal invasiveness and are relatively stable. On the other hand, tumor tissues could contain heterogeneity that complicates the analysis but also provide rich data regarding genetic mutations associated with specific cancers.
The unique feature of using such various source materials lies in their contribution towards targeted research areas. Blood samples are often associated with genetic disease studies. In contrast, tumor tissues are indispensable in cancer genomics, enabling researchers to understand tumor evolution.
RNA vs. DNA
The choice between RNA and DNA for sequencing has significant implications for the types of insights gained. RNA sequencing allows for a look into gene expression and can identify active genes in a given state or condition, thus revealing a lot of dynamic information about cellular functions. In contrast, DNA sequencing offers insights into genetic variations and mutations.
Among the key characteristics, RNA is particularly useful when examining conditions like cancer. It can help clarify how gene expression levels change. Conversely, DNA sequencing lays the groundwork for understanding inherited genetic conditions. Each approach has its own strong and weak points. RNA sequencing, while providing real-time insights, can introduce challenges related to RNA stability. DNA sequencing, being more stable, offers a rich depository for long-term studies but may not always reflect active processes.
Data Analysis and Interpretation
Computational Tools
The use of computational tools in analyzing sequencing data is indispensable. These tools enable researchers to handle large data sets efficiently and extract meaningful biological insights. Software packages such as GATK (Genome Analysis Toolkit) and SAMtools help in variant calling and data preprocessing.
A key characteristic of these computational tools is their ability to automate many steps, which reduces human error and improves reproducibility. However, the complexity and need for appropriate training can be a double-edged sword, as these tools have steep learning curves for those unfamiliar with bioinformatics.
Bioinformatics Pipeline
Setting up a bioinformatics pipeline is a critical step in managing the sequencing data workflow. This pipeline encompasses various stages such as data preprocessing, alignment, variant calling, and annotation.
The importance of a well-structured bioinformatics pipeline cannot be overstated. A robust and carefully designed pipeline can significantly improve the quality of the results and facilitate easier interpretation. However, building such a pipeline demands both time and expertise, which could be a hurdle for smaller labs. The unique feature here lies in how varying analytical approaches can lead to different interpretations of the same data set, emphasizing the necessity for clear documentation and standardization.
Quality Control Measures
Ensuring Data Integrity
Maintaining data integrity is paramount in exon sequencing. Inaccuracies can arise from various sources, including sample contamination and technical variances during sequencing runs. Data integrity ensures that the results accurately reflect the true genetic landscape of the samples being studied.
A primary characteristic of ensuring data integrity involves implementing strict laboratory protocols, including regular calibrations of sequencing machines and preventing cross-contamination between samples. Such measures foster a reliable output, though they may require significant upfront investment regarding time and resources.
Error Management
Error management strategies are essential to detect and resolve potential discrepancies during the sequencing process. Errors can arise in many forms, including sequencing errors and systematic failures in data processing.
A strong focus on error management can allow researchers to trace back issues to their source, effectively limiting the implications of such errors on their results. The characteristic feature is how adaptive these measures can be, enabling teams to modify protocols as needed for future experiments. While these strategies add to operational costs, the benefits of minimizing erroneous conclusions often outweigh the downsides.
In summary, the methodological considerations surrounding exon sequencing encompass a wide array of factors that affect the overall success of the approach. From careful selection of source materials to rigorous data analysis and robust quality control mechanisms, each piece adds a vital layer of complexity and opportunity for researchers. Understanding these aspects is crucial for maximizing the potential benefits of exon sequencing in both research and clinical applications.
Challenges in Exon Sequencing
Exon sequencing presents immense potential in the realm of genomics, but it also faces its share of hurdles. These challenges are paramount as they can hinder the effectiveness of exon sequencing, thus limiting its application in both clinical and research environments. The discourse around these issues not only highlights the inherent complexities involved but also directs future efforts aimed at overcoming them. By acknowledging these challenges, we better equip ourselves to navigate the ever-evolving landscape of genetics.
Technological Limitations
Coverage Gaps
One of the most glaring issues in exon sequencing is the problem of coverage gaps. Coverage gaps refer to the regions within an exome that do not receive sufficient sequencing depth. This is critical since inadequate coverage can lead to undetected mutations, skewing results and potentially leading to incorrect conclusions about genetic predispositions. The key characteristic of coverage gaps is their ability to arise from various factors including, but not limited to, sequencing biases and the inherent difficulties presented by GC-rich regions of DNA which do not amplify well.
When coverage gaps occur, the unique feature here is that certain genetic variations can be missed entirely. This disadvantage can be particularly troubling when it comes to identifying mutations that are crucial for understanding complex diseases or tailoring treatments based on genetic profiles. In many cases, tackling this issue requires additional sequencing runs or a shift towards improved library preparation techniques. This enhances confidence and reduces the risk of missed variants, which is crucial for accurate interpretation in both research and clinical settings.
Cost Implications
Another critical challenge tied to exon sequencing is the cost implications. The evolution of sequencing technologies, particularly next-generation methods, has revolutionized genomics but often comes at a steep price. The high costs associated with quality exon sequencing can be a barrier for many researchers and institutions, particularly in resource-limited settings.
The key characteristic of cost implications is that they can significantly influence the scope and scale of genomic research. For example, a lab may have to prioritize which projects to pursue based on their budgetary restrictions, potentially sidelining significant investigations due to financial constraints. A unique feature of the cost is how it fluctuates based on the technique employed—some methods may be more advanced yet prohibitively expensive compared to traditional approaches. This poses a disadvantage as it directly affects the accessibility of exon sequencing for larger populations who could benefit from genetic insights.
Ethical Considerations
As exon sequencing continues to develop, it brings along a set of ethical considerations that cannot be overlooked. These issues are particularly significant given how genomics can directly impact individuals' lives, health decisions, and societal norms. Delving into these ethics is crucial for responsibly advancing this field further, ensuring that science progresses hand-in-hand with moral accountability.
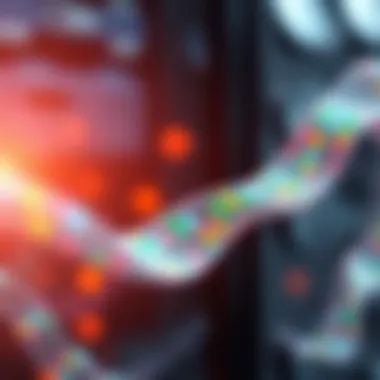
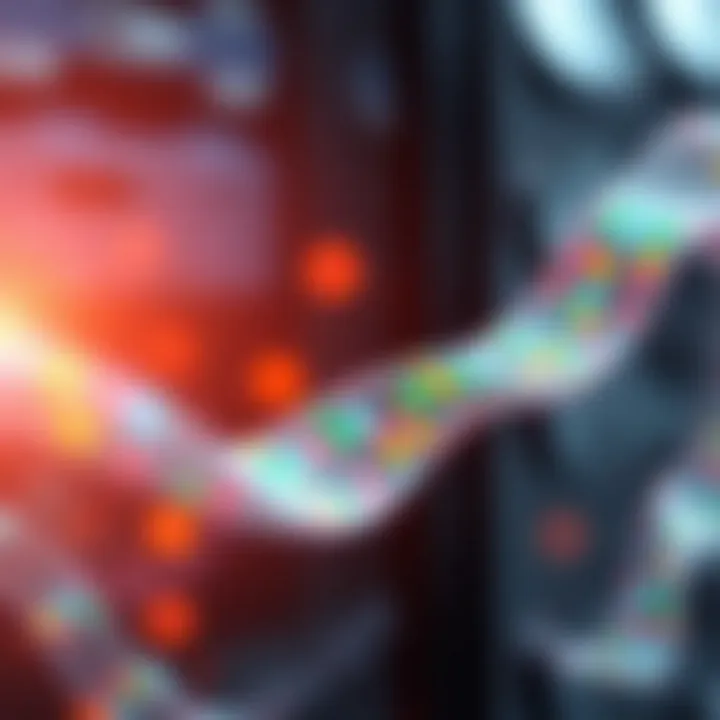
Data Privacy
In the digital age, data privacy stands as a critical issue in the realm of exon sequencing. The unique aspect of data privacy concerns revolves around how genetic information is stored, shared, and used. Genetic data could potentially reveal sensitive information about an individual’s health predispositions, thus calling for strict protocols on data handling. The risks associated with data breaches can lead to misuse, discrimination, or even stigmatization in certain scenarios. This makes data privacy not only a technical requirement but a fundamental ethical obligation that researchers must take seriously.
The advantages of safeguarding data privacy are manifold; it builds trust in research, encourages participation among subjects, and ensures compliance with legal frameworks. Conversely, stringent privacy measures may also complicate collaborative efforts, potentially hindering advancements in genetic research. Balancing protection with progress is a pivotal concern in handling genetic data responsibly.
Informed Consent
Informed consent is another vital element intertwined with ethical considerations in exon sequencing. This concept emphasizes that participants are fully aware of what their genetic testing entails, including potential risks and benefits before they agree to be involved in any study. It’s crucial for ensuring that individuals maintain control over their genetic information. The key characteristic here is that informed consent goes beyond mere paperwork; it embodies an ethical framework that respects individual autonomy and promotes transparency in the research process.
Moreover, the unique nature of genetic data means that implications can extend beyond the individual to their families, raising complexities regarding consent and the sharing of results. While informed consent protects individuals, its execution can sometimes limit the speed of research due to the necessity of ensuring clarity and understanding among participants. Thus, the process requires careful consideration, balancing the rights of individuals with the objectives of scientific inquiry.
In the rapidly advancing field of exon sequencing, recognizing these challenges is imperative for guiding ethical best practices and technological advancements.
As we advance in the study and application of exon sequencing, addressing these challenges not only enhances research methodologies but fosters a robust ethical framework, paving the way for innovations that are as responsible as they are groundbreaking.
Future Prospects of Exon Sequencing
The future of exon sequencing is not just a pathway but rather a sprawling landscape filled with promising technologies and methodologies. It shapes the genetic blueprints and could potentially alter the trajectory of medicine and research. As we peer into the horizon, the implications reverberate across various disciplines: from understanding rare diseases to tailoring cancer therapies. Each innovative stride provides researchers and clinicians with the tools to delve deeper into genetic complexities.
Technological Innovations
Single-Cell Sequencing
Single-cell sequencing represents a leap in technology that allows the examination of individual cells rather than bulk samples. This is a game changer. One of the cornerstone traits of single-cell sequencing is its ability to capture cellular heterogeneity. This characteristic enables researchers to differentiate between cell types, revealing insights previously masked by averaging techniques. For researchers, working on cancer or developmental biology, this approach bears immense potential.
A unique feature of single-cell sequencing is its capacity to illuminate how individual cells respond to various stimuli or treatments. This granular level of detail can be critical in identifying mutations that are pivotal in diseases. Yet, it’s not without its drawbacks; the method can be relatively costly and technically demanding. Despite these challenges, the benefits outweigh the limitations, making it a popular choice within the scope of genomic studies.
Long-Read Sequencing Techniques
Long-read sequencing techniques further enhance the capabilities of exon sequencing by providing a broader view of the genomic landscape. Traditional methods often struggle with complex regions of the genome; however, long-read technologies can span thousands of base pairs in a single read. This key feature makes it particularly useful for uncovering structural variants and comprehensive transcript isoforms—a feat that’s quite elusive with shorter reads.
Moreover, the ability to sequence larger fragments means that researchers can achieve a more complete representation of genomic architecture. One downside is that these techniques can be less efficient and are still developing in terms of throughput compared to their short-read counterparts. Nevertheless, their proficiency in tackling nomenclature challenges of the human genome is invaluable—paving the way for future applications in genomics.
Integration with Other Genomic Approaches
Whole Genome Sequencing
Whole genome sequencing represents a holistic approach furthering the understanding of the entire genetic landscape, not just the exons. This technique’s primary characteristic is providing a comprehensive view of an organism's genetic makeup. In this juncture, whole genome sequencing captures all variation, from the exceedingly common to the exceedingly rare.
The unique feature here lies in its capacity for a complete genetic assessment, facilitating the identification of not only coding variants but also regulatory elements that might impact gene expression. This could be a turning point in personalized medicine, allowing clinicians to devise advanced treatment plans. That being said, it can generate vast amounts of data requiring significant computational resources and expertise to analyze—something that may be a roadblock for some institutions.
Transcriptome Analysis
Transcriptome analysis delves into gene expression at a given point in time. This process involves examining all RNA transcripts, providing a dynamic view of what genes are actively being expressed within cells. The essential characteristic of transcriptome analysis is its ability to capture the intricacies of gene regulation and expression profiles. This forms the backbone of functional genomics, shedding light on pathways involved in various diseases.
A unique advantage of transcriptome analysis is its ability to reveal non-coding RNAs' roles, which are often overlooked in standard sequencing methodologies. Yet, it too has its challenges; issues concerning data interpretation can arise, particularly because it requires a contextual framework to discern meaningful biological relevance from the sheer volume of data.
"In the realm of genomics, integration is not merely beneficial; it is essential for harnessing the power of sequence data for novel discoveries."
Closure
In wrapping up our comprehensive exploration of exon sequencing, it's essential to recognize its far-reaching implications in the realms of genomics and medicine. Exon sequencing stands as a pivotal player in the decoding of genetic information, serving as a lens through which both researchers and clinicians examine the intricate dance of gene expression and variation. It doesn't just hold the keys to understanding genetic diseases and cancers; it also plays a vital role in tailoring personal treatment regimens, ushering us into an age where medicine becomes increasingly individualistic.
Summary of Key Points
Throughout the article, we’ve traversed various facets of exon sequencing, including:
- Definition and Importance: We’ve established that exon sequencing is vital for understanding gene function and the genetic landscape of diseases.
- Technique Exploration: A comparative analysis of multiple sequencing techniques highlighted the innovative strides made in next-generation sequencing, with its high throughput and efficiency standing out.
- Wide-ranging Applications: From diagnosing rare genetic disorders to identifying targeted therapies in cancer genomics, the application potential appears limitless.
- Methodological Considerations: The importance of rigorous sample preparation and error management was underscored to ensure quality and integrity in data collection and interpretation.
- Challenges and Solutions: We addressed technological constraints and ethical dilemmas that persist in exon sequencing, focusing on how these challenges can be navigated.
- Future Prospects: Innovations, like single-cell and long-read sequencing, alongside integrations with other genomic techniques, present exciting avenues for research and application.
These points come together to illustrate exon sequencing not merely as a technological advancement but a pivotal aspect of modern medicine and genetic research.
The Path Forward
Looking ahead, the path for exon sequencing unfolds with promise and possibility. While there are hurdles to overcome, such as addressing data privacy concerns and making sequencing more cost-effective, the continuous advancements in technology offer a silver lining.
- Emphasis on Collaboration: The future could very well depend on interdisciplinary collaboration among geneticists, bioinformaticians, and ethicists to harness the full potential of exon sequencing while navigating its complexities responsibly.
- Enhancing Accessibility: Efforts to democratize access to sequencing technology can catalyze breakthroughs in understanding and treating diseases while ensuring that a broader population can benefit from these advancements.
- Innovative Research Directions: Future research should also focus on developing algorithms that can better interpret exon sequencing data, especially as we delve deeper into personalized medicine.