An In-Depth Exploration of Phase Shifting Interferometry
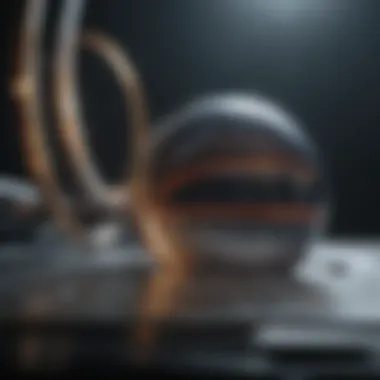
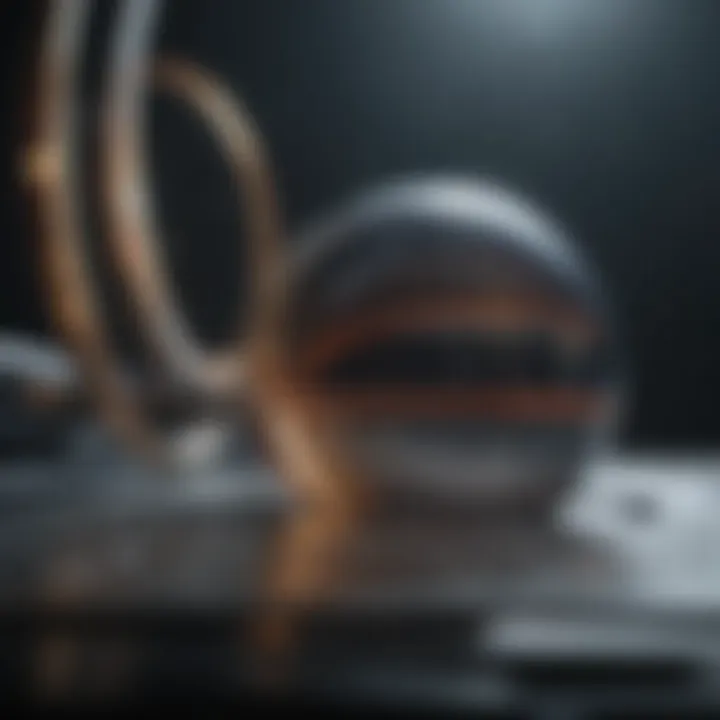
Intro
Phase shifting interferometry (PSI) stands as a crucial optical technique with profound implications across various fields. This method, primarily used for measuring phase differences between light waves, facilitates the acquisition of high-resolution data concerning optical surfaces and materials. The following sections will dissect the fundamental principles of PSI, its applications, and advancements, alongside its comparison with traditional interferometry methods. By examining these elements, we aim to highlight the significance of PSI in enhancing precision measurement and analysis.
Research Overview
Summary of Key Findings
This exploration reveals that PSI delivers a number of advantages over conventional interferometry. Key findings include:
- High sensitivity to phase changes, enabling detailed assessments of surface profiles.
- Efficiency in data processing, attributed to techniques such as digital image processing.
- Diverse applications across disciplines, including materials science, manufacturing, and optics.
Overall, PSI significantly enhances measurement accuracy, leveraging advances in computational methods for improved data interpretation.
Importance of the Research
The relevance of PSI is particularly pronounced in high-precision environments. Various sectors utilize this optical technique to achieve high accuracy in measurements. For instance, industries focused on manufacturing optical components benefit from PSI’s ability to detect minute surface irregularities. Research facilities apply PSI to gain insights into materials at a microscopic level, influencing future developments in technology.
Methodology
Study Design
The methodological framework utilized in PSI involves both systematic and empirical approaches. Each technique follows standard operating procedures to ensure consistency in results. Key phases include:
- Calibration of equipment to ensure accurate measurements.
- Method validation through comparative analysis with conventional techniques.
- Data analysis using sophisticated algorithms to interpret phase shifts.
Data Collection Techniques
In PSI, data collection relies on advanced optical setups that typically consist of:
- Interferometers to project and manipulate light beams.
- Cameras to capture interference patterns.
- Computers equipped with software for processing and analyzing collected data.
This combination of tools allows for a holistic approach to phase measurement, where nuances are captured systematically.
"Phase shifting interferometry reshapes how we perceive and measure light interactions at the microscopic level."
The insights afforded by PSI are paramount for evolving industries that prioritize precision and accuracy in their manufacturing and scientific endeavors.
Throughout this article, we will further dissect the principles, applications, and technological advancements that underscore the value of PSI in fostering greater understanding and innovation.
Prologue to Phase Shifting Interferometry
Phase shifting interferometry (PSI) is a significant advancement in optical measurement techniques. Its ability to provide precise readings of phase differences between two light waves places it at the forefront of modern metrology. The importance of PSI lies in its capacity to enhance the accuracy and resolution of optical measurements across various fields, from material science to biomedical applications.
This section is essential as it lays the foundation for understanding how PSI operates and contrasts with older techniques. It also addresses the benefits of PSI, namely, improved measurement capabilities, sensitivity to minute changes, and quicker data acquisition. These advantages make PSI an attractive choice for researchers and professionals alike, as they seek more reliable and efficient methods of analysis.
Moreover, knowing the basic principles behind PSI allows practitioners to grasp its potential applications. As precision measurement becomes increasingly vital in scientific research and industry, a solid understanding of PSI is indispensable for anyone looking to stay relevant in this dynamic field.
Definition and Basic Principles
Phase shifting interferometry works on the principle of light wave interference. Interference occurs when two or more light waves meet and interact. The resulting interference pattern can reveal critical information about the surfaces being measured. The fundamental concept is based on measuring the changes in phase between the reference and test beams of light.
At the core of PSI is the process of phase shifting, which involves intentionally altering the phase of one beam of light in a controlled manner. This shifting can be achieved using various techniques, such as modulating the optical path length or introducing a variable optical element. As the phase shift occurs, the interference pattern changes correspondingly, which can be recorded and analyzed.
In detail, PSI typically utilizes a series of phase shifts, often recorded as a time sequence of intensity patterns at the detector. It is through mathematical analysis of those changes and applying phase recovery algorithms that one can extract accurate phase information from the interference patterns. This method allows for high-resolution measurements even in the presence of noise or environmental disturbances.
Comparison with Traditional Interferometry Methods
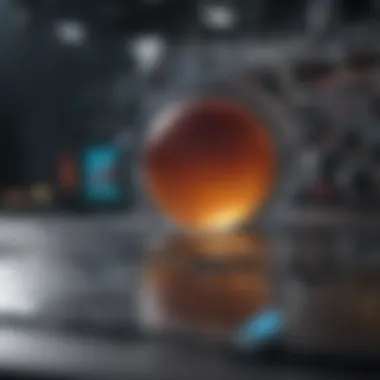
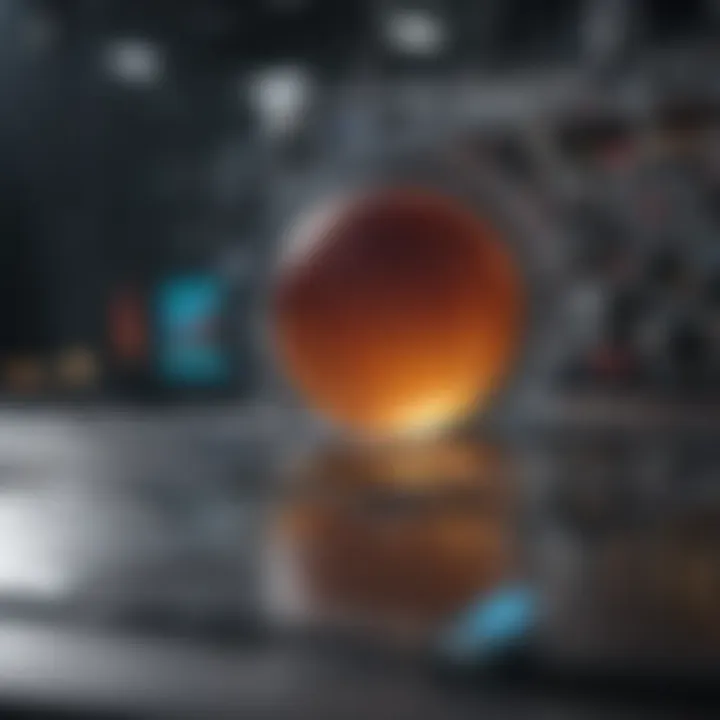
Traditional interferometry methods, such as the Michelson or Mach-Zehnder interferometers, have their limitations. They often rely on maintaining stable and controlled optical paths, which can be challenging in many practical scenarios. These methods typically produce measurements that can be sensitive to environmental factors, leading to inaccuracies.
In contrast, phase shifting interferometry provides several advantages over these classical techniques.
- Increased Sensitivity: PSI can detect smaller phase changes with higher confidence, allowing for the measurement of finer features on optical surfaces.
- Reduced Sensitivity to External Factors: PSI lessens the effects of noise and environmental fluctuations through its phase shifting process, enhancing the reliability of the measurements.
- Faster Data Acquisition: The ability to gather multiple phase-shifted patterns in a brief period leads to faster measurements, making PSI suitable for real-time applications.
Ultimately, the evolution from traditional methods to phase shifting interferometry signifies a critical advancement in the domain of precision measurements. This transition underscores a growing need for techniques that can provide both accuracy and efficiency in increasingly complex experimental settings.
Thus, understanding the definition and principles of PSI, as well as its advantages over traditional methods, sets the stage for the deeper exploration of its mechanisms, applications, and future potential in the sections that follow.
Historical Background of Interferometric Techniques
The evolution of interferometric techniques is crucial to understanding the development of phase shifting interferometry. These historical foundations provide insights into the methodologies and philosophies that shaped modern measurement techniques. Interferometry's origins date back to the 19th century, where the groundwork was laid for obtaining precise measurements of light wave interactions. The exploration of these developments provides context for the innovations present in contemporary PSI methods. As the field advanced, it became evident that refined measurement techniques were essential for scientific progress across multiple disciplines.
Early Developments in Interferometry
The field of interferometry began with experiments by notable physicists such as Augustin-Jean Fresnel and Joseph von Fraunhofer in the early 1800s. Their work primarily focused on the study of light and its properties through diffraction and interference patterns. The Fresnel lens and the Fraunhofer diffraction concepts were pivotal in setting the stage for future innovations. These early techniques allowed scientists to observe and understand light behavior with unprecedented accuracy.
Furthermore, the advent of the Michelson interferometer in the late 19th century marked a significant leap forward. This device enabled the precise measurement of small distances by analyzing the interference patterns formed by light waves split into two paths. Michelson's work led to the famous Michelson-Morley experiment, which aimed to detect the existence of the "aether". Although the experiment ultimately refuted this theory, it remained a landmark moment in optics, establishing a firm basis for further exploration in interferometry.
Evolution of Phase Shifting Techniques
The transition towards phase shifting interferometry came about in response to the limitations of traditional interferometry methods. While early interferometric techniques served well for various applications, they faced challenges in achieving high resolution and accurate phase measurement. By the late 20th century, advancements in optical technology and digital processing paved the way for the development of phase shifting techniques. They harnessed the advantages of capturing phase information, enabling measurement precision that earlier methods could not achieve.
A key feature of these techniques is the ability to extract phase differences more reliably. In phase shifting interferometry, a series of carefully timed phase shifts are introduced into the wavefronts, allowing for the collection of multiple intensity measurements. The subsequent analysis relies on sophisticated algorithms that compute the phase from these intensity data effectively.
These advancements not only improved measurement accuracy but also expanded the applicability of interferometry across various domains, including optical surface profiling and biomedical imaging. The continuous evolution of phase shifting techniques reflects the ongoing pursuit of precision and the importance of adapting traditional methods to meet modern scientific demands.
"The journey of interferometric techniques showcases the relentless pursuit of precision in the field of optical measurements."
In summary, the historical background of interferometry informs our understanding of phase shifting techniques today. This context highlights how early innovations laid the foundational knowledge critical for the development of PSI, shaping its place in the current landscape of optical measurement technologies.
Fundamentals of Phase Shifting Interferometry
Understanding the fundamentals of phase shifting interferometry (PSI) is vital for comprehending its capabilities and applications in precision measurement. PSI is not just an advanced optical technique; it is a vital tool that enhances measurement accuracy in various fields such as engineering, biology, and physics. The importance of this topic lies in its ability to provide high-resolution analysis, enabling researchers to gather data that traditional techniques may overlook. By delving into the essential components of PSI, one can appreciate how it achieves such precision and the implications for future developments in measurement technology.
Working Mechanism of PSI
The working mechanism of phase shifting interferometry is grounded in the concept of phase differences in light waves. In simplest terms, PSI involves splitting a coherent light source, usually a laser, into two beams. These beams travel different paths and then recombine to create an interference pattern. This process happens in several steps:
- Beam Splitter: The coherent light is initially directed through a beam splitter, which divides it into two paths.
- Interference: Each beam encounters the surface or material under analysis. Their phase shifts as they reflect off different surfaces.
- Recombination: When the two beams converge again, they create an interference pattern that varies according to the phase difference.
- Phase Shifting: By systematically altering the path length of one beam, a series of interference patterns are collected. This variation allows for accurate phase measurement.
Ultimately, PSI's ability to measure tiny phase differences enables researchers to characterize optical surfaces with remarkable precision.
Sampling and Data Acquisition
Effective sampling and data acquisition is a critical aspect of PSI. The quality of the data collected directly influences the accuracy of the measurements. In this context, the following components are essential:
- Sampling Rate: The frequency at which the data is collected should be sufficiently high to capture rapid fluctuations in the interference pattern.
- Sensitivity of Detectors: The detectors employed, such as CCD or CMOS sensors, must have high sensitivity to detect phase variations with minimal noise.
- Data Processing: After acquiring the raw data, it is processed to determine the phase information. This includes filtering techniques to remove unwanted noise and improve signal integrity.
Overall, the efficiency of data acquisition in PSI is paramount for ensuring reliable and valid measurements, making it an integral part of the method.
Phase Recovery Algorithms
Phase recovery algorithms are crucial for interpreting the data derived from PSI. These algorithms convert the interference patterns into usable phase information. Several key techniques exist:
- Fourier Transform Method: This algorithm utilizes Fourier transforms to analyze the frequency components of the interference patterns, allowing for efficient phase recovery.
- Least Squares Fitting: This approach is used for fitting the acquired data to a model and minimizing the difference, leading to accurate phase measurements.
- Iterative Algorithms: Some advanced methods involve iterative calculations that refine phase estimates step by step, improving accuracy.
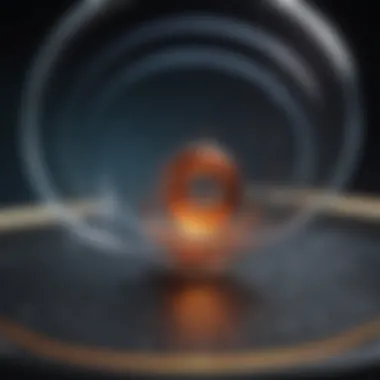
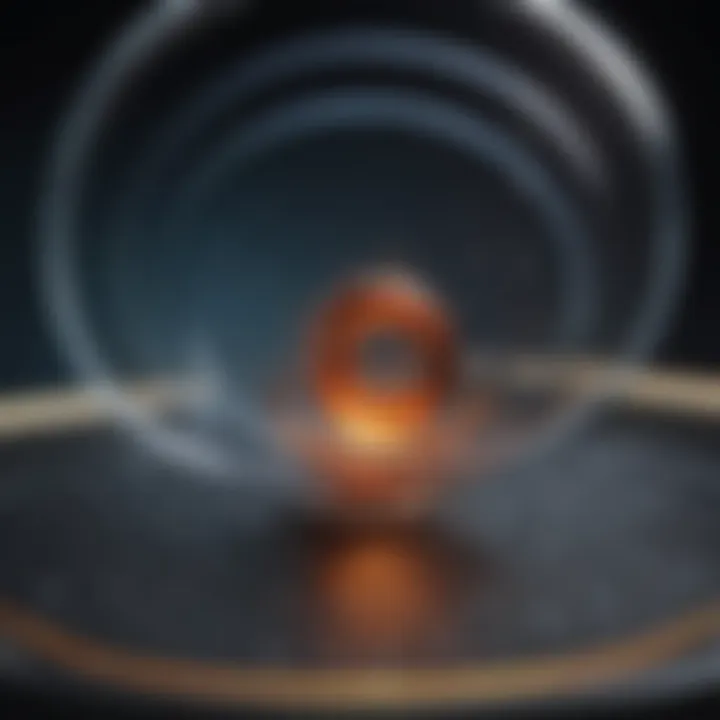
These algorithms ensure that the phase data extracted from PSI is as accurate as possible, which is essential for effective applications in research and industry. In summary, the fundamentals of PSI encompass its mechanism, effective data practices, and the critical algorithms that govern its operation, all contributing to its prominence as an indispensable measurement tool.
Applications of Phase Shifting Interferometry
Phase shifting interferometry (PSI) offers a wide range of applications across various scientific and industrial fields. Its ability to provide high-resolution measurements makes it an invaluable tool for precise analysis. Understanding these applications illuminates the significance of PSI in enhancing research capabilities and quality control processes.
Optical Surface Profiling
Optical surface profiling is one of the primary applications of phase shifting interferometry. PSI is used to measure surface topographies of optical components with high accuracy. This capability is crucial in industries like semiconductor manufacturing, where component quality directly affects functionality.
In this context, PSI helps in detecting surface irregularities, such as scratches or defects, which can impact the performance of optical systems. By applying PSI methods, engineers can create detailed maps of surface profiles, allowing them to identify issues before products reach the market.
The adoption of PSI for optical surface profiling includes several key advantages:
- High precision: PSI enables measurements on the nanometer scale.
- Non-destructive: This technique measures surfaces without altering or damaging them.
- Speed: Quick acquisition of data facilitates efficient testing and quality assurance.
Measurement of Thin Films
Thin film measurement is another significant application of phase shifting interferometry. The ability to accurately quantify the optical thickness and properties of films is essential in fields such as materials science and microelectronics. PSI can assess the uniformity of thin film coatings, crucial in the development of photonic devices.
For instance, in the production of LED technology, precise measurements enable manufacturers to ensure that layers are applied uniformly and meet stringent specifications. Furthermore, PSI can help in identifying material properties like refractive index, which are critical for designing optical components.
Several aspects underscore the benefits of PSI in this area:
- Sensitivity: PSI's high sensitivity allows it to detect minute variations in thin film coatings.
- Versatility: Suitable for various materials, from semiconductors to polymers.
- Real-time measurement: Facilitates immediate feedback during manufacturing processes to enhance productivity.
Biomedical Applications
In the biomedicine field, phase shifting interferometry plays a pivotal role in diagnostic techniques. Its capability to provide precise measurements of biological structures makes it suitable for various applications including cellular imaging and physiological studies. PSI allows researchers to analyze cell membranes and tissue structures without invasive procedures.
One prominent application is in optical coherence tomography, where PSI aids in creating detailed cross-sectional images of tissues. This technology has vast implications in oncology, dermatology, and ophthalmology. By using PSI, clinicians can monitor changes in tissues, which aids in early disease detection and monitoring the effectiveness of treatments.
Key benefits of utilizing PSI in biomedical fields include:
- Improved imaging resolution: Offers clearer images of biological tissues.
- Non-contact measurements: Minimizes the risk of damaging sensitive biological samples.
- Quantitative analysis: Provides accurate data for research and clinical decisions.
Phase shifting interferometry offers unparalleled abilities to address critical challenges across diverse sectors, enhancing observations that were previously difficult or impossible to obtain.
As seen, PSI has established itself as a fundamental method for optical surface profiling, measurement of thin films, and promoting advancements in biomedical applications. The capability of PSI to deliver high-resolution data fosters innovation and precision across various critical industries.
Advantages and Limitations of Phase Shifting Interferometry
Phase shifting interferometry (PSI) is a powerful measurement technique that finds applications in a variety of fields. However, like any advanced technology, it comes with its own set of advantages and limitations. Understanding these aspects is essential for professionals considering its implementation in research or industrial settings.
High Resolution and Sensitivity
One of the primary advantages of phase shifting interferometry is its remarkable high resolution. The ability of PSI to measure minute phase changes allows for detailed surface profiling and precise measurements of optical elements. This sensitivity is crucial for applications where even the smallest discrepancies can lead to significant errors. In fields such as optics and materials science, PSI enables researchers to analyze and develop surfaces and coatings with exceptional clarity.
The interference patterns generated in PSI provide quantifiable data that can be used to assess surface quality, thickness of thin films, and more. This leads to enhanced quality control processes in industries like semiconductor manufacturing, where precise measurements are paramount. The capacity for non-contact measurements also means that delicate materials can be inspected without the risk of damage.
Challenges in Implementation
While PSI offers many benefits, it is not without challenges in its implementation. One notable limitation is the need for high-quality optical components. Any imperfections in the optics can introduce errors in measurement, undermining the precision PSI is known for. Furthermore, aligning the optical system requires precise calibration and setup, which can be time-consuming and may demand specialized knowledge.
Another challenge is environmental sensitivity. PSI equipment can be affected by vibrations, air currents, and temperature fluctuations. Maintaining a stable measurement environment is critical, adding to the constraints for practical applications. Additionally, interpreting the data from PSI can be complex, requiring a strong understanding of the algorithms and phase recovery methods used in the analysis process.
Recent Advances in Phase Shifting Interferometry
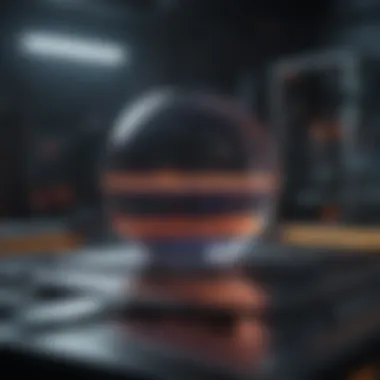
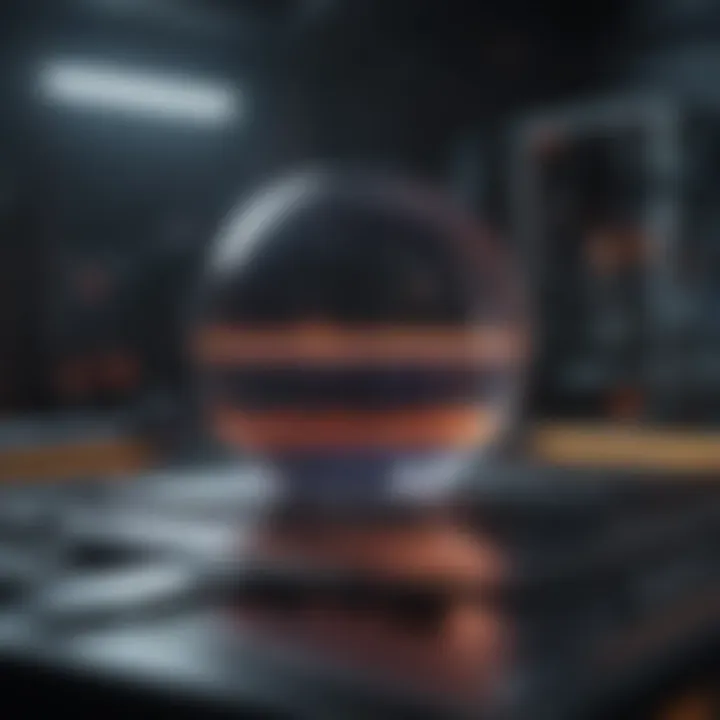
Phase shifting interferometry has witnessed significant growth due to technological advancements. These developments are crucial for enhancing measurement accuracy and expanding the utility of PSI across various fields. This section will explore two main areas of recent progress: the integration with digital technologies and the development of new algorithms.
Integration with Digital Technologies
The integration of digital technologies into phase shifting interferometry has been transformative. High-speed cameras and sophisticated image processing software allow for more accurate data acquisition. This combination enables researchers to handle larger data sets with ease and speed. Advanced digital sensors improve the sensitivity of measurements, leading to better resolution.
Key benefits of this integration include:
- Improved Accuracy: Digital devices reduce noise, increasing the precision of optical surface measurements.
- Real-Time Analysis: Modern software can analyze data on-the-fly, providing immediate feedback during experiments, which is essential in fast-paced research environments.
- Automation: Automation of measurement processes reduces human error and enhances repeatability, making experiments more reliable.
This digital evolution enables the application of PSI in complex and real-time scenarios, expanding its potential use in fields like engineering and medicine.
Development of New Algorithms
Algorithm development is another pivotal area advancing phase shifting interferometry. Algorithms designed for phase recovery play a critical role in extracting phase information from interferometric data. Traditional methods often struggled with noise and complex surfaces, but newer approaches have addressed these challenges.
Recent advancements include:
- Adaptive Algorithms: These algorithms adjust to the specific conditions of the measurement environment, optimizing results based on real-time feedback.
- Machine Learning Techniques: By utilizing machine learning, researchers can enhance phase retrieval processes. Machines can learn from data patterns, improving accuracy over time.
- Multi-Frame Algorithms: These approaches utilize multiple measurement frames to provide better phase information synthesis, thereby enhancing signal quality in noisy environments.
"These innovations expand the horizons of PSI, enabling it to tackle problems previously deemed too complex or challenging."
The development of robust algorithms is essential for maximizing the capabilities of PSI and ensuring it remains at the forefront of metrology disciplines.
In summary, the recent advances in phase shifting interferometry are primarily driven by digital integration and new algorithmic developments. Their implications extend to various sectors, promising to democratize access to precise measurement techniques while simultaneously paving the way for innovative applications.
Future Prospects of Phase Shifting Interferometry
The future prospects of phase shifting interferometry (PSI) are pivotal for advancing various fields of research and technology. As optical measurement techniques continue to evolve, PSI stands out due to its high precision and versatility. The integration of new technologies, such as machine learning and artificial intelligence, promises to enhance the efficacy of PSI methods. This integration not only streamlines data analysis but also improves phase recovery, making it essential for intricate measurements.
Ongoing developments suggest a trend toward miniaturization of equipment, which could make PSI more accessible across different industry applications. Compact PSI systems, capable of delivering high-resolution results in real time, can foster greater implementation in environments that previously could not accommodate larger setups. Furthermore, emerging materials, such as metamaterials, may enable the creation of more sensitive interferometric devices, pushing the boundaries of what PSI can achieve.
Emerging Trends in Interferometry
One significant trend in the field of interferometry, especially related to PSI, is the shift toward digital technologies. Traditional optical components are being augmented with digital signal processing. This shift makes it possible to manipulate and analyze phase data with unprecedented accuracy and speed. As algorithms become more refined, their ability to handle larger sets of data without degradation of performance becomes highly beneficial.
Additionally, there is a growing focus on integrating PSI with other measurement technologies. Combining PSI with techniques like atomic force microscopy or scanning tunneling microscopy could lead to new hybrid approaches, allowing for comprehensive surface characterization at the nanoscale level. These convergences will likely facilitate breakthroughs in various scientific disciplines, especially materials science and nanotechnology.
Potential Impact on Research and Industry
The implications of advanced PSI methods on research and industry are substantial. In academic research, precise measurements can lead to a better understanding of fundamental properties of materials. For instance, in semiconductor industries, PSI could enhance the monitoring of surface quality during production, thereby improving yield rates.
In the biomedical realm, the potential for high-resolution imaging and profiling can yield better diagnostic tools and treatment monitoring systems. As another example, PSI might be instrumental in optical coherence tomography for analyzing tissue at a cellular level. These impacts seem not only beneficial but necessary as the demand for accuracy in measurements grows across various sectors.
Moreover, industries such as telecommunications, aerospace, and defense can leverage PSI for developing advanced sensors and monitoring systems. As we move towards a more connected world, the precision that PSI provides can be instrumental in ensuring quality control and enhancing product reliability.
"The advancement of phase shifting interferometry signifies an important step forward in our capability to measure and analyze complex systems with precision."
End
The conclusion serves as a pivotal part of this article, summarizing the integral components of phase shifting interferometry (PSI) and reflecting on its significance in both research and industry. Throughout this exploration, PSI has emerged not merely as a measurement technique but as an essential tool that enhances precision in various applications. This technique bridges gaps in current optical technologies, offering detailed insights into complex surfaces and materials.
Summation of Key Insights
Phase shifting interferometry notably excels in high-resolution surface measurements, which is significant in multiple disciplines. Key insights include:
- PSI allows for the accurate measurement of phase differences, crucial for surface profiling and characterization, especially in optics and materials science.
- The method enhances accuracy compared to conventional interferometry methods, thus providing more reliable data for scientific applications.
- Recent technological advancements have led to integration with digital tools, improving the efficiency and accessibility of PSI.
- There are challenges, primarily in the implementation phase, that require careful consideration and innovation to overcome.
Final Thoughts on the Future of PSI
Looking ahead, the future of phase shifting interferometry is promising. As research continues to evolve, several trends may shape its development:
- The continuous enhancement in algorithms will likely lead to even higher sensitivity and speed in measurements, making psi a go-to resource in time-critical applications.
- The exploration of novel materials in various fields like biomedical engineering could foster new applications, pushing the boundary of what PSI can achieve.
- Collaborative endeavors between academia and industries can enhance research translation to practical applications, thus fostering innovation.
- Lastly, ongoing advancements in technology, such as artificial intelligence and machine learning, may revolutionize data acquisition and analysis in PSI, paving the way for its broader use.
In closing, the profound impact of phase shifting interferometry extends beyond its immediate applications, embodying a convergence of technology, technique, and scientific inquiry that is likely to shape future explorations in optics and beyond.