Exploring UV Light Spectroscopy: Principles and Advances
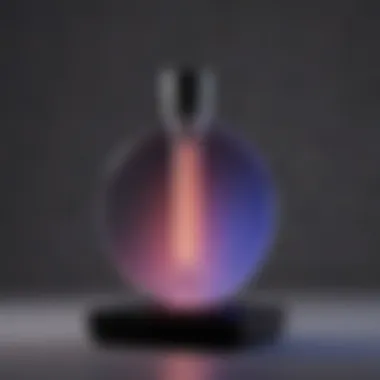
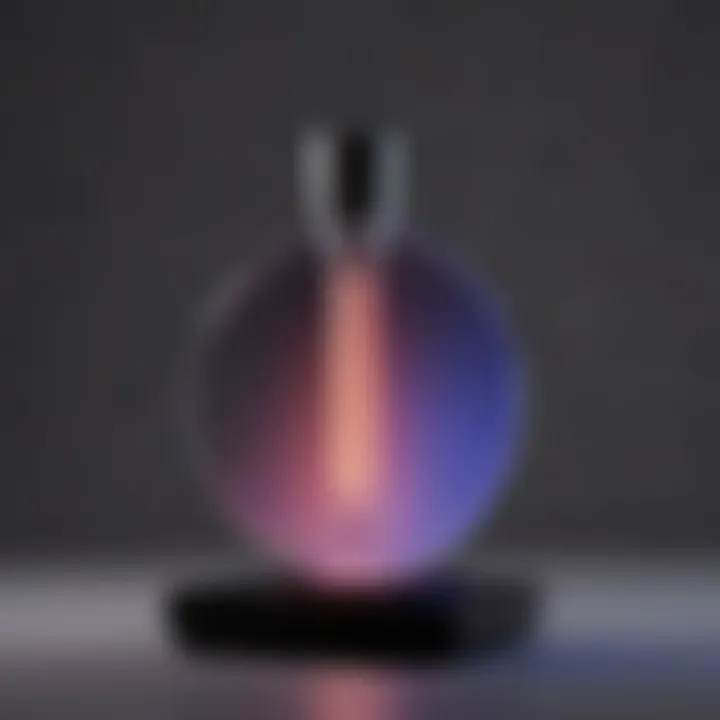
Intro
UV light spectroscopy is a powerful analytical technique utilized across various disciplines, including chemistry, biology, and materials science. This method involves the interaction of ultraviolet light with matter, providing insights into the molecular structure and concentration of substances. By understanding the principles of UV spectroscopy, one can appreciate its significance in both qualitative and quantitative analysis.
The techniques and tools available today have evolved significantly. Modern instrumentation has improved sensitivity, specificity, and resolution. This evolution facilitates more complex analyses, enabling researchers to explore intricate systems. Moreover, UV spectroscopy's applications are diverse, influencing areas such as drug development, quality control, environmental monitoring, and many more.
In this article, we will examine the fundamental principles of UV spectroscopy, explore its methodologies, and discuss its practical applications. We will also highlight recent advancements in instrumentation and identify future directions for research in this important field.
Preamble to UV Light Spectroscopy
UV light spectroscopy is a significant analytical technique utilized in various scientific disciplines, including chemistry, biology, and materials science. This introductory section will elucidate its importance, focusing on both its foundational concepts and its diverse applications.
UV light, or ultraviolet light, has wavelengths shorter than visible light, making it a powerful tool for examining the properties of molecules. The ability of UV light to excite electrons in atoms and molecules is central to its application in spectroscopy. Understanding the principles behind UV light spectroscopy not only provides insight into the composition of materials but also empowers researchers to innovate in fields ranging from pharmaceuticals to environmental science.
Definition and Overview
UV light spectroscopy refers to the study of the interaction between ultraviolet radiation and matter. It involves measuring the absorption of UV light by different substances. The resulting data allows scientists to deduce various characteristics of the material, such as concentration, structure, and electronic properties. This technique falls under the umbrella of absorption spectroscopy, characterized by the quantification of light absorbed by a sample as a function of wavelength.
In practical terms, UV spectroscopy is frequently employed to analyze biological samples, quantify chemical compounds, and monitor environmental pollutants. The crucial point is that different substances absorb UV light at characteristic wavelengths, permitting identification and quantitation. Its importance in fields such as analytical chemistry cannot be overstated, given its precision and reliability in measuring diverse samples.
Historical Context
The exploration of ultraviolet light began in the 19th century. The foundation for UV light spectroscopy was laid when Johann Wilhelm Ritter discovered ultraviolet radiation in 1801. This marked a significant turning point, as it opened new avenues for research and analytical techniques. Subsequent developments in technology led to the design of the first spectrophotometer in the early 20th century. This invention permitted more accurate measurements of light absorption, facilitating the rise of UV spectroscopy as a respected analytical method.
During the mid-20th century, significant advancements were made in instrumentation. The introduction of double-beam spectrophotometers improved the accuracy and reliability of measurements. This evolution has allowed UV spectroscopy to become integrated into various scientific practices. As a result, UV light spectroscopy has evolved considerably, remaining relevant in modern analytical techniques. The historical trajectory of UV light spectroscopy underscores its adaptability and essential role in scientific inquiry, paving the way for innovations that continue to emerge today.
Fundamental Principles of UV Spectroscopy
Understanding the fundamental principles of UV spectroscopy is crucial for comprehending its applications and relevance across scientific disciplines. It serves as the foundation upon which various analytical techniques are built. By grasping the principles, one can effectively interpret results and optimize methodologies used in UV spectroscopy. This section will delve into the nature of UV light, molecular absorption, and the Beer-Lambert law, all of which play a pivotal role in the functioning and interpretation of UV spectroscopy.
Nature of UV Light
Ultraviolet (UV) light encompasses a region of the electromagnetic spectrum with wavelengths ranging from approximately 10 nm to 400 nm. UV light can be categorized into three primary regions: UVA (long-wave), UVB (medium-wave), and UVC (short-wave). Each of these categories has distinct properties and effects on matter, critical for various experimental conditions.
UV light is utilized primarily because of its ability to cause electronic transitions in molecules. When a molecule absorbs UV light, electrons are promoted from a ground state to an excited state. This energy absorption leads to changes that can be measured, providing insights into the molecular structure and composition of samples. The interaction of UV light with matter makes this region of the spectrum particularly important in qualitative and quantitative analysis.
Molecular Absorption
Molecular absorption refers to the process by which molecules absorb light energy, leading to transitions between energy levels. In UV spectroscopy, it is the differential absorption of UV light that allows for the assessment of the concentration of specific molecules in solution. Each molecule has a unique electronic structure, which corresponds to specific wavelengths of light that it can absorb.
This principle forms the basis for identifying compounds in a mixture. The resulting absorption spectrum is a characteristic fingerprint, which provides valuable information about concentration and identity. Factors such as temperature and solvent can affect absorption characteristics, necessitating careful control during analysis to ensure accurate results.
Beer-Lambert Law
The Beer-Lambert Law is a mathematical equation that describes the relationship between absorbance, the concentration of a solution, and the path length through which light travels. The law states:
Where:
- A is the absorbance (no units),
- ε is the molar absorptivity (L mol^-1 cm^-1),
- c is the concentration of the absorbing species (mol L^-1), and
- l is the path length of the light through the sample (cm).
Understanding the Beer-Lambert Law is essential for performing quantitative analyses. It provides a linear relationship between absorbance and concentration, thereby allowing researchers to determine unknown concentrations from known standards. However, this law holds under certain conditions, such as low concentration and limited scattering of light, highlighting the necessity for careful experimental design.
"The Beer-Lambert Law remains a vital equation in UV spectroscopy, acting as a bridge between concentration and observable absorbance, making it essential for analytical work."
In summary, the fundamental principles of UV spectroscopy not only govern the operation of the technique but also influence how results are interpreted in the context of various scientific inquiries. Grasping these basic elements lays the groundwork for a deeper understanding of more complex applications as outlined in subsequent sections.
Instrumentation in UV Spectroscopy
Instrumentation is at the heart of UV spectroscopy. It determines the reliability and accuracy of measurements obtained from the UV spectrum. The complex nature of UV absorption implies that the design and choice of instrument play a vital role in various scientific outcomes. This section dives into the core components, their functions, and the significance of proper instrumentation in the process of UV spectroscopy.
Spectrophotometers
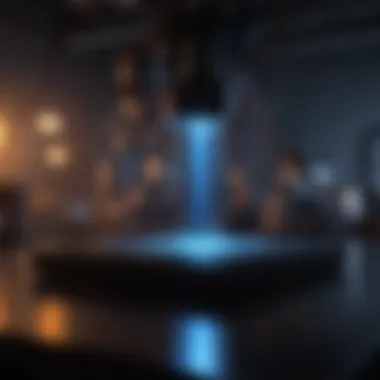
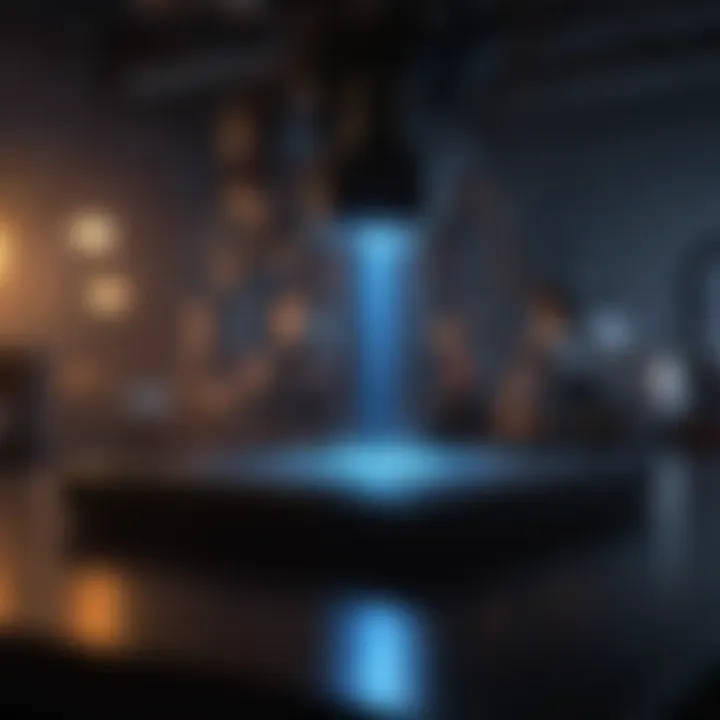
Spectrophotometers are crucial devices used in UV spectroscopy. They measure the intensity of light absorbed by a sample at specific wavelengths. The operation of spectrophotometers typically involves multiple components including a light source, a monochromator, a sample holder, and a detector.
- Light Source: The light source can be a deuterium lamp or a tungsten lamp. Each lamp covers a specific range of wavelengths. Deuterium lamps are preferred in the UV region as they emit light from about 160 nm to 400 nm, while tungsten lamps cover the visible range.
- Monochromator: This component isolates specific wavelengths from the light source, allowing only a certain wavelength to pass through to the sample. Monochromators can use prisms or diffraction gratings. The choice of monochromator affects the resolution and sensitivity of the spectroscopy technique.
- Sample Holder: Samples are placed in cuvettes. Cuvettes come in different materials such as quartz or glass. The choice of material impacts the UV transparency and ultimately the measurements taken.
- Detector: The detector measures the amount of light passing through the sample. Photomultiplier tubes or photodiodes are common types of detectors used, converting light into an electrical signal for data analysis.
Proper calibration and maintenance of these components ensure the accuracy and reliability of spectral data. By understanding the function of each part, users can better utilize spectrophotometers in various applications—from analytical chemistry to material science.
Detectors and Their Functionality
Detectors play a pivotal role in determining the effectiveness of UV spectroscopy. The choice of detector can influence the sensitivity and speed of measurements. The most common types of detectors used in UV spectroscopy include:
- Photomultiplier Tubes (PMTs): PMTs are highly sensitive and can detect low levels of light. They are suitable for applications requiring high sensitivity.
- Photodiodes: These detectors offer faster response times and are robust, making them suitable for real-time measurements in various industrial applications.
- Charge-Coupled Devices (CCDs): CCDs are common in modern UV spectrometers. They provide spatial resolution and can capture multiple wavelengths simultaneously, improving data collection efficiency.
Each detector type has its advantages and limitations. The selection should be based on the specific needs of the experiment, such as light intensity, speed, and sensitivity.
Calibration Techniques
Calibration is essential in ensuring the accuracy of UV spectroscopy measurements. Several techniques can be employed to calibrate spectrophotometers:
- Wavelength Calibration: This ensures that the instrument reports the correct wavelengths. It often involves using standard reference materials with known absorbance peaks.
- Absorbance Calibration: This procedure establishes a relationship between concentration and absorbance (according to the Beer-Lambert Law). Calibration standards must be prepared to ensure that the spectrophotometer readings correlate with known concentrations.
- Detector Calibration: Essential to correct any drift in sensitivity over time, recalibration of the detector guarantees that the readings remain consistent.
- Baseline Correction: This technique accounts for any background interference in the measurements, allowing for more accurate results.
Regular calibration ensures precision and reduces errors caused by instrument variability.
Proper instrumentation in UV spectroscopy leads to reliable data, which is crucial for making informed decisions in research and industry.
In summary, the instrumentation in UV spectroscopy incorporates multiple components that work together to provide accurate spectroscopic data. Understanding how each element functions leads to better usage of UV spectroscopy in various applications.
Types of UV Spectroscopy
Understanding different types of UV spectroscopy is essential in comprehending the broad range of applications and methodologies within this field. Each type has distinct advantages and considerations that influence its use in various scientific contexts. The selection of a specific UV spectroscopy method can greatly affect the results obtained and the efficiency of the analysis process. This section will discuss three main types: Single-Beam, Double-Beam, and Time-Resolved UV spectroscopy, as well as FT-UV spectroscopy, shedding light on their importance and utility.
Single-Beam vs. Double-Beam Spectroscopy
Single-beam and double-beam UV spectrophotometers serve pivotal roles in the analysis of light absorption.
In single-beam spectroscopy, a single pathway is utilized for the light to pass through. Samples are measured sequentially, meaning calibration against a blank must be performed each time a measurement is taken. While this method is more straightforward and less expensive, it is susceptible to fluctuations in light sources, which may lead to reduced accuracy in results.
In contrast, double-beam spectroscopy employs two separate light paths: one for the sample and one for the reference. This setup allows for real-time comparison, as it mitigates the impact of light source variability. The continuous evaluation of reference and sample enhances precision, making this option preferable for intricate analyses, especially where high accuracy is crucial.
Time-Resolved UV Spectroscopy
Time-resolved UV spectroscopy is primarily utilized to study fast processes or reactions that occur on very short timescales. This technique enables the observation of transient species and reaction intermediates that would usually be undetectable in standard spectroscopic methods. By employing pulsed light sources, the system captures time-dependent changes in the sample’s absorption.
This spectroscopic method proves vital in areas like photochemistry and biological processes, where the understanding of dynamic changes aids in revealing mechanisms that govern reactions. For example, researchers can utilize this approach to dissect complex biochemical pathways, offering insights critical for drug development and therapeutic interventions.
FT-UV Spectroscopy
Fourier Transform UV (FT-UV) spectroscopy is another advanced technique that merges the principles of UV spectroscopy with Fourier transform methodologies. This method enables the rapid acquisition of spectra, thanks to its ability to gather data across a wide frequency range in one single measurement. The implementation of FT-UV enhances resolution and sensitivity compared to traditional methods, allowing for the detection of minute quantities of analytes.
Benefits of FT-UV include improved speed in data collection, providing more efficient workflows in laboratory settings. It finds applications across multiple disciplines, including chemistry, biology, and materials science. The integration of FT-UV into analytical processes underscores the ongoing evolution in instrumentation that boosts capabilities and enriches research outcomes.
"The right choice of UV spectroscopy type can enhance accuracy and efficiency in analysis, crucial for scientific integrity."
In summary, knowing the distinctions and applications of the types of UV spectroscopy is vital for practitioners. Each method offers unique advantages that are suited for specific analytical needs and scenarios. Thus, a thoughtful approach to selecting the appropriate type is essential.
Applications of UV Spectroscopy
The applications of UV spectroscopy are vast and varied, reflecting its significance across multiple scientific disciplines. This section delves into the specific contexts in which UV spectroscopy is employed, underscoring its advantages and utility. With its ability to analyze materials and quantify substance concentrations, UV spectroscopy serves as a crucial tool in laboratories, industries, and research settings. Key benefits of its application include high sensitivity, rapid analysis times, and versatility in detecting various analytes.
Analytical Chemistry
In analytical chemistry, UV spectroscopy is fundamental for both qualitative and quantitative analysis. It enables chemists to determine the composition of substances by measuring their absorption of ultraviolet light. As light interacts with different molecules, unique absorption spectra emerge. Each compound has a specific absorption pattern that can act as a fingerprint for identification. One common application is in the analysis of pharmaceuticals where UV spectroscopy can ascertain purity and concentration of active ingredients.
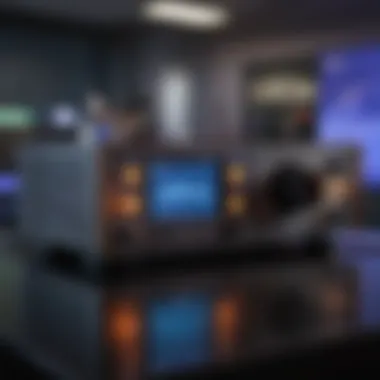
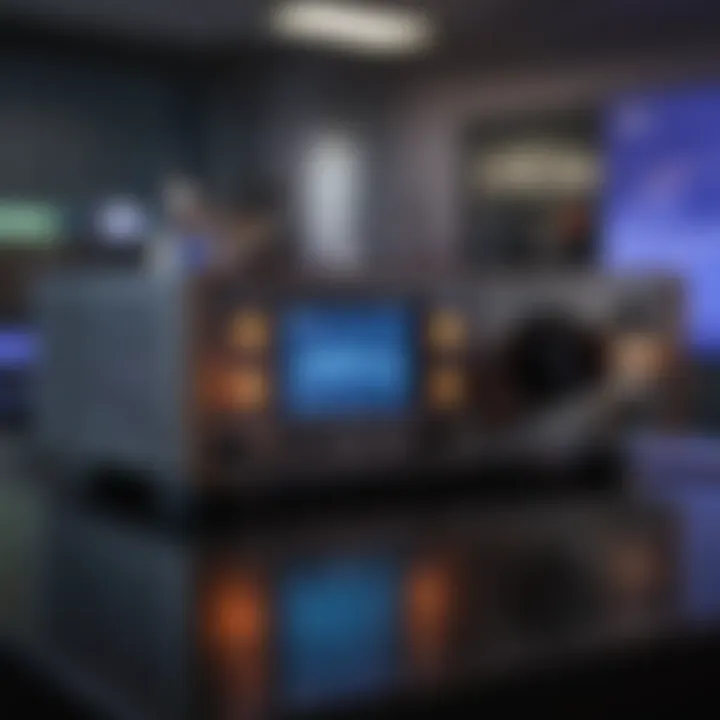
Benefits of UV Spectroscopy in Analytical Chemistry:
- High Sensitivity: Capable of detecting low concentration levels, essential for trace analysis.
- Speed: Provides quick results, which is crucial in high-throughput laboratories.
- Ease of Use: Generally, requires minimal sample preparation compared to other methods.
For instance, in the context of drug formulation, scientists can utilize UV spectroscopy to ensure the correct dosage of active ingredients is present in a new pharmaceutical formulation. By creating standard calibration curves, they can accurately measure concentrations, leading to consistency in therapeutic efficacy.
Biological Studies
UV spectroscopy plays a vital role in biological studies, especially in assessing biomolecules such as proteins and nucleic acids. The technique helps researchers to investigate molecular interactions alongside their conformation and concentration. A common example is the study of protein folding through UV absorption analysis at specific wavelengths. Additionally, it aids in monitoring enzyme activities and interactions with substrates.
Notably, UV spectroscopy can provide insights into the stability of proteins over time, offering critical data in biopharmaceutical development.
Use Cases in Biological Studies:
- DNA and RNA Analysis: Measuring the absorbance of nucleic acids at 260 nm allows accurate quantification and purity assessment.
- Protein Concentration Determination: Absorbance at 280 nm is often used to estimate protein concentrations due to specific aromatic amino acids.
As a result, UV spectroscopy aids in advancing our understanding of biological processes, making it indispensable for research in fields such as biochemistry and molecular biology.
Material Science
In material science, UV spectroscopy is valuable for characterizing the optical properties of materials and coatings. The analysis can reveal the absorption characteristics of polymers, films, and nanomaterials, crucial for applications in electronics and photonics. Furthermore, UV spectroscopy is employed to evaluate the photostability of materials, determining how well they perform when exposed to UV light.
Key Applications in Material Science:
- Quality Control: Testing the consistency and quality of materials and coatings before their application.
- Comparative Studies: Evaluating different materials for use in solar cells, sensors, or other optical devices based on their UV absorption properties.
In summary, the applications of UV spectroscopy are not limited to one field but extend across multiple disciplines such as analytical chemistry, biological studies, and material science. By facilitating rapid analysis and providing critical data, this technique continues to be an indispensable asset in modern scientific research.
Case Studies in UV Light Spectroscopy
In exploring UV light spectroscopy, it is essential to consider practical applications through case studies. These case studies serve as a bridge between theoretical understanding and real-world implementation. They illuminate the significance of UV spectroscopy across various fields, underscoring its capabilities in addressing complex scientific questions. By examining specific examples, we can evaluate the effectiveness, reliability, and adaptability of UV light spectroscopy techniques in different scenarios.
UV Spectroscopy in Pharmaceutical Analysis
Pharmaceutical analysis often relies on UV spectroscopy for the assessment of drug purity and concentration. Using this technique, scientists can quantify active pharmaceutical ingredients in formulations. The method is particularly advantageous due to its speed and simplicity. For instance, UV spectroscopy was successfully used in the analysis of ibuprofen, allowing researchers to determine the compound's concentration with minimal sample preparation. This efficiency makes UV spectroscopy a go-to technique in quality control laboratories.
Furthermore, comparison with chromatographic methods indicates that UV spectroscopy can achieve comparable results without extensive operation or lengthy procedures. Comprehensive studies confirm that this approach is effective in detecting impurities, a critical aspect in drug formulation.
Environmental Monitoring Using UV Techniques
Environmental sciences benefit greatly from UV light spectroscopy, especially in monitoring water quality. A noteworthy case study involved the assessment of organic pollutants in river water. By employing UV spectroscopy, researchers detected and quantified substances like phenols and nitrates. The results demonstrated a clear correlation between absorbance levels at specific wavelengths and the concentration of contaminants.
The application of UV techniques increases in importance when considering regulatory compliance. For example, the EPA has established specific guidelines for permissible levels of various contaminants, and UV spectroscopy provides a quick and cost-effective means of evaluation. The results from these environmental studies emphasize the reliability of UV methods for timely decision-making in pollution control.
Food Safety and Quality Control
The field of food safety has also seen enhanced monitoring with UV spectroscopy. Researchers used this method to analyze adulteration in olive oil. UV spectral data provided insight into the authenticity of the samples by identifying specific absorbance peaks that indicate the presence of lesser-grade oils.
Moreover, UV spectroscopy aids in assessing the quality of fresh produce. A notable study examined the firmness of apples. By measuring the UV light absorption characteristics at various stages of ripeness, researchers correlated these values with textural properties.
These examples highlight how UV techniques not only ensure compliance with safety standards but also enhance product quality and consumer trust. Effective UV spectroscopy applications in the food industry stress the approach's versatility and significance in maintaining safety and standards.
In summary, through these case studies, it is clear that UV light spectroscopy holds a crucial position in pharmaceutical analysis, environmental monitoring, and food safety. The technique’s capacity for rapid, accurate, and cost-effective analysis underscores its growing relevance in contemporary scientific practices.
Understanding case studies is vital as they provide a practical context to the theoretical aspects of UV light spectroscopy. Each application demonstrates how the technique can be adapted to serve various critical fields, from healthcare to environmental safety, proving its versatility.
Challenges in UV Spectroscopy
UV light spectroscopy is a powerful analytical tool, but like any technology, it faces its share of challenges. These challenges can affect the accuracy and reliability of results. Identifying and understanding these barriers will help guide future innovations in the field. This section presents a detailed examination of the limitations of current techniques and issues with sample preparation. By addressing these challenges, researchers can enhance the efficacy of UV spectroscopy applications.
Limitations of Current Techniques
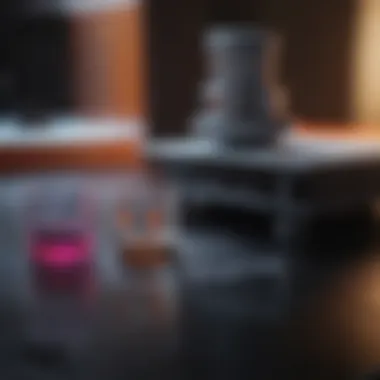
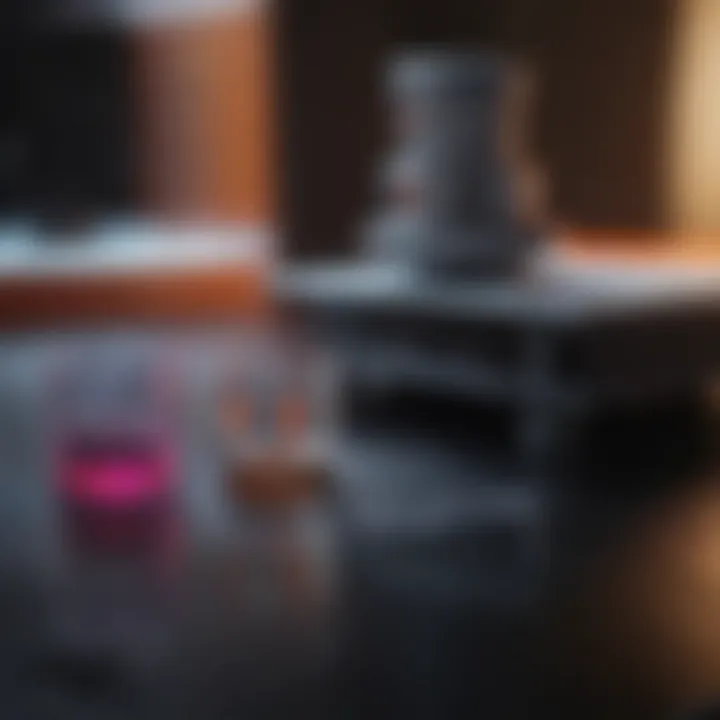
The field of UV spectroscopy has made significant strides since its inception. However, some limitations remain prominent.
- Sensitivity and Detection Limitations: Many spectrometric instruments struggle to detect low concentrations of analytes. The sensitivity is particularly an issue when analysts work with complex matrices, leading to interference that complicates the results.
- Resolution Issues: In some applications, such as distinguishing similar compounds, the resolution of existing UV spectrometers might not suffice. This poor resolution means that closely related molecules can yield overlapping absorbance spectra, making interpretation difficult.
- Range of Detection: Most UV spectrometry methods operate effectively within a specific range of wavelengths. This limitation can hinder analysis in cases where substances absorb at wavelengths outside of this range. Expanding the range of detection could unlock new analytical possibilities.
These limitations highlight the need for ongoing refinement in UV spectroscopy techniques and instrumentation. Evolution in this field is crucial for improving specificity and overall analytical accuracy.
Issues with Sample Preparation
Sample preparation plays a critical role in the analytical success of UV spectroscopy. Missteps during this phase can lead to erroneous data.
- Matrix Effects: Compounds present in the sample matrix can interfere with the measurement. Ideally, samples should be prepared in such a way to minimize these effects, but this can be technically challenging due to the diverse nature of samples in real-world applications.
- Contamination Risks: The risk of contaminating samples during preparation is ever-present. Such contamination can significantly alter the optical properties of the samples, leading to inaccurate readings. It is vital to use proper techniques and clean environments to protect samples from unwanted substances.
- Homogeneity of Samples: Ensuring the uniformity of samples is essential. Heterogeneity can lead to inconsistencies in absorbance measurements, thus skewing the results. Adequate mixing and sample handling techniques are necessary to achieve reliable and reproducible results.
"Addressing the challenges in UV spectroscopy is not just about overcoming obstacles; it is about enhancing scientific understanding and expanding its utility across disciplines."
In summary, addressing these challenges is essential for the ongoing development and application of UV spectroscopy in various fields.
Future Directions in UV Spectroscopy Research
The field of UV spectroscopy is evolving rapidly, with numerous advancements poised to enhance its applications across various disciplines. As scientists explore the capabilities of ultraviolet light, it becomes critical to discuss future directions in this area. Innovations in technology offer promising solutions to the limitations currently faced in UV spectroscopy and broaden its potential applications,
Emerging technologies play a crucial role in transforming how UV spectroscopy is used in research and industry. These advancements increase accuracy, sensitivity, and overall effectiveness. Considering how the integration of contemporary methods alongside traditional techniques could further elevate the impact of UV spectroscopy is essential.
Emerging Technologies
In the realm of UV spectroscopy, several emerging technologies are gaining momentum. One significant trend is the development of portable UV spectrometers. These devices enable field analysis, making it easier to gather data in diverse environments.
Additionally, advances in computational power have also transformed data analysis. Machine learning algorithms are beginning to be integrated into UV spectroscopic analysis, allowing for faster and more accurate interpretation of results. This is particularly important in complex samples where traditional analysis may falter.
Moreover, the development of novel materials for detectors has increased the sensitivity and range of UV spectrometers. For instance, nanoscale materials can provide improved detection limits and spectral range, further exploring previously unquantifiable substances.
These innovations not only streamline processes but also extend the application of UV spectroscopy into fields such as environmental monitoring, food safety, and biomedical research.
Integration with Other Spectroscopic Techniques
Another promising direction is the integration of UV spectroscopy with other spectroscopic techniques. This synergistic approach amplifies the strengths of each method, leading to more comprehensive analysis.
For instance, combining UV spectroscopy with infrared spectroscopy can create a more nuanced picture of molecular structures and interactions within various samples. Such integration improves the capacity for qualitative and quantitative analysis, leading to insightful interpretations.
Moreover, hyphenated techniques like UV-Vis coupled with chromatography, such as High-Performance Liquid Chromatography (HPLC), provide enhanced separation and identification of compounds. This is particularly useful in complex matrices where specific components need to be isolated and studied.
The future of UV spectroscopy hinges on its adaptability and integration with advancing technologies. As researchers employ these emerging technologies and collaborative approaches, they will unlock new potential applications and enhance the reliability and efficiency of UV spectroscopy in contemporary scientific exploration.
End
UV spectroscopy has emerged as a pivotal tool in various scientific fields, maintaining its significance through both historical and contemporary lenses. This article has explored the fundamental principles and methodologies that underpin this technique, as well as its diverse applications across analytical chemistry, biology, and materials science.
The Continued Relevance of UV Spectroscopy
The ongoing relevance of UV spectroscopy can be primarily attributed to its versatility. In an age where precision in measurements is paramount, UV spectroscopy stands out due to its ability to provide both qualitative and quantitative data. Its applications range from simple concentration measurements in lab settings to complex analyses in environmental science.
Moreover, advancements in instrumentation continue to enhance its efficiency and accuracy. Newer spectrophotometers incorporate features like greater sensitivity and faster data acquisition, allowing researchers to extract more information from their samples in less time. As a result, UV spectroscopy is not only relevant today but is also vital for future research and innovation.
"UV spectroscopy is a foundational tool that merges simplicity with powerful analytical capabilities."
Additionally, the integration of UV spectroscopy with other spectroscopic techniques is expanding its horizons further, facilitating multidimensional analyses. This capability reflects the adaptability of UV spectroscopy to meet evolving scientific demands.
Cited Works and Further Reading
Citing authoritative texts and articles enhances the academic rigor of this piece. Here are some key references that support the content:
- Principles of Instrumental Analysis by Douglas A. Skoog et al. - This book delivers an extensive overview of different analytical techniques, including UV spectroscopy.
- Spectroscopy: Fundamentals and Applications by A. John. This text details a multitude of spectroscopy types, making it an ideal reference for comparative studies.
- Articles from Analytical Chemistry Journal that highlight the latest advancements in UV spectroscopy instruments and methodologies.
Additionally, following these resources, a reader will gain a comprehensive understanding of UV light spectroscopy:
- Wikipedia's page on Ultraviolet Spectroscopy for a concise overview.
- Britannica's informative articles that offer insights into both fundamental principles and applications.
- Engaging discussions on platforms like Reddit provide a community perspective, showcasing practical applications and troubleshooting methodologies in laboratory settings.
While these references serve as a fundamental part of this article, they also allow for a broadening of knowledge beyond the primary text. Engaging with these works cultivates a more intricate understanding of UV spectroscopy. It is always beneficial to review additional literature to enhance one's grasp of the subject.