GFP Filters in Fluorescence Microscopy Explained
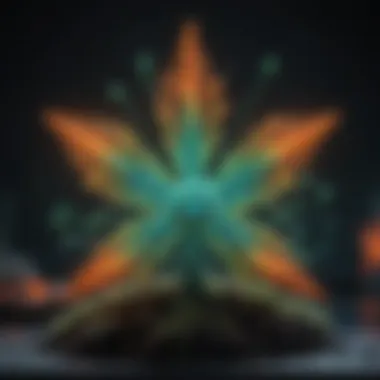
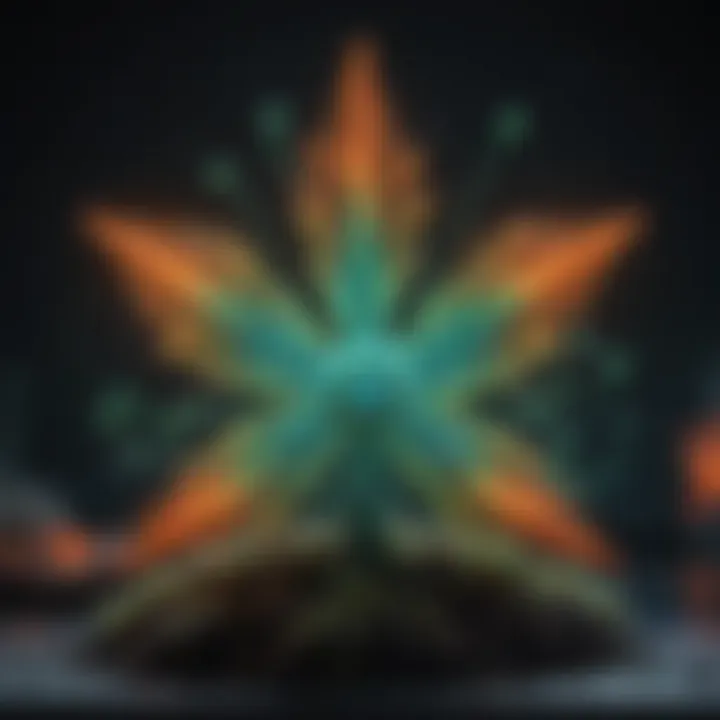
Intro
Fluorescence microscopy is a valuable technique in biological research, allowing scientists to visualize and study specific proteins and other molecules within cells. Green Fluorescent Protein (GFP) has become a staple in this field, providing a green signal that can be easily detected. However, the effectiveness of GFP imaging heavily relies on the excitation and emission filters used in the microscopy process. These filters play a crucial role in isolating the fluorescence signal from background noise, hence optimizing image quality.
This article will delve into the various aspects of excitation and emission filters in fluorescence microscopy, particularly in the context of GFP applications. By examining the principles behind filter functionality, we aim to equip researchers with essential knowledge for effective filter selection and implementation. Furthermore, we will highlight advancements in filter technology and their implications for fluorescence imaging.
Foreword to Fluorescence Microscopy
Fluorescence microscopy serves as a cornerstone technique in modern biological research. This method allows scientists to observe and analyze cellular structures and processes with exceptional clarity and resolution. The importance of fluorescence microscopy lies in its capability to visualize biological specimens that would otherwise be invisible under traditional light microscopy. This article extensively discusses how excitation and emission filters, specifically in relation to Green Fluorescent Protein (GFP), play a crucial role in optimizing imaging results.
In fluorescence microscopy, the interactions of light with the specimen generate signals, which are captured to form images. The selection of appropriate optical filters is integral to this process. Filters determine the specific wavelengths of light that are allowed to pass through the system, ensuring that only the desired fluorescence signals are detected while filtering out unwanted noise.
To appreciate the intricacies of GFP fluorescence imaging, it is essential to understand the principles of fluorescence, the functionality of the filters, and their impact on data interpretation. Filtering out the specific excitation and emission wavelengths ensures that researchers obtain high-contrast images, which is pivotal for accurate analysis and conclusions.
Overview of Fluorescence Principles
Fluorescence arises from the absorption of light by a molecule, transitioning it to an excited state. When the molecule relaxes back to its ground state, it releases energy in the form of light at a longer wavelength. The principle of this process is central in microscopy, particularly with fluorescent dyes such as GFP. Understanding fluorescence is key to effective imaging, and the right choice of excitation and emission filters directly influences the quality of fluorescence observed.
- Key components of fluorescence principles include:
- Absorption of photons by the fluorescent molecule
- Excitation to a higher energy state
- Relaxation and emission of light at a longer wavelength
The effectiveness of fluorescence microscopy hinges on these underlying principles, dictating how images are acquired and analyzed.
Significance of GFP in Biological Research
Green Fluorescent Protein (GFP) is an invaluable tool in the field of cellular biology. Originally derived from the jellyfish Aequorea victoria, GFP provides a means to visualize proteins and other cellular components in real-time within living cells. Its significance lies in its non-invasive nature, allowing researchers to monitor biological processes without altering the subject under study.
Using GFP has transformed our understanding of several biological phenomena, including protein expression, cellular localization, and biological signaling pathways. Its application extends across various fields, such as neurobiology, cancer research, and developmental biology.
The advantages of using GFP include:
- High brightness and photostability
- Versatility in tagging various proteins
- Capacity to be used in live-cell imaging
In summary, GFP's role as a fluorescent marker extends beyond simple visualization; it is essential for investigating the dynamics of living systems, leading to significant advancements in biological research.
Understanding GFP: Structure and Function
In fluorescence microscopy, understanding the Green Fluorescent Protein (GFP) is essential. This knowledge provides insights into how GFP functions and how it can be utilized in various applications. GFP serves as a powerful tool in biological research, enabling scientists to visualize cellular processes in real time. Its structure and function affect how effective GFP is as a fluorescent marker. Thus, discussing the molecular characteristics and the mechanism of GFP fluorescence enhances our comprehension of its role in imaging technologies.
Molecular Characteristics of GFP
The Green Fluorescent Protein is derived from the jellyfish Aequorea victoria. Its structure consists of a beta-barrel formed by 11 strands, enclosing a chromophore that is responsible for its fluorescence. This unique configuration offers stability and allows GFP to function under various cellular conditions.
Some key characteristics include:
- Stability: GFP is relatively stable, which allows for extended observation without significant photobleaching. This is critical in time-lapse imaging.
- Brightness: GFP displays high quantum yield, which means it emits a strong fluorescence signal. This brightness is favorable for clear imaging.
- Variants: Numerous variants of GFP exist, such as enhanced GFP (eGFP) and mCherry. These variants have different excitation and emission properties, enabling multi-color labeling in multiplex experiments.
Understanding these molecular aspects helps researchers select suitable GFP variants and predict their behavior in specific applications.
Mechanism of GFP Fluorescence
GFP fluorescence results from a specific chemical reaction involving the chromophore within the protein. The chromophore undergoes a process called proton transfer after it is formed. The following steps summarize the mechanism:
- Excitation: When GFP is exposed to light of an appropriate wavelength, the electrons in the chromophore become excited.
- Emission: As electrons return to their ground state, they release energy in the form of light, resulting in the characteristic green fluorescence of GFP.
- Dynamic Stability: The protonated state of the chromophore is necessary for optimal fluorescence. Variations in environmental pH can affect this state, impacting fluorescence intensity.
Understanding this mechanism is vital for optimizing imaging conditions and choosing the right excitation and emission filters. Proper filtering enhances the captured signal and reduces background noise, improving the overall imaging quality.
By grasping the structure and function of GFP, researchers can maximize its potential in various investigative scenarios and refine their microscopy techniques.
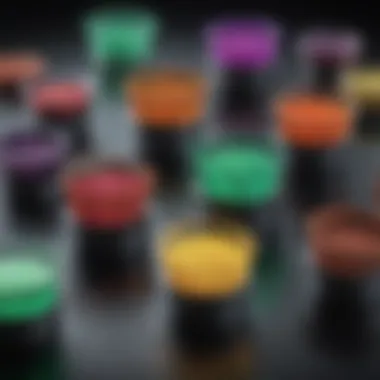
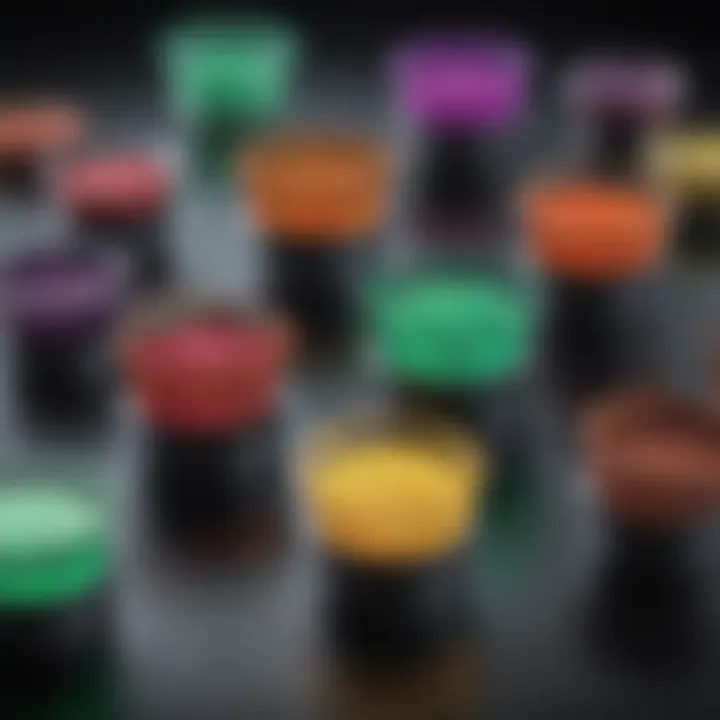
Key Components of Fluorescence Microscopy
Fluorescence microscopy is a powerful technique widely used in biological research. It relies on key components that work together to produce high-quality imaging results. Understanding these elements is crucial. This section will cover the role of light sources and the importance of optical filters. Both are essential for maximizing the potential of fluorescence microscopy.
The Role of Light Sources
Light sources are the starting point for fluorescence microscopy. They provide the excitation light necessary for exciting fluorescent molecules. Different types of light sources can be used, including mercury vapor lamps, xenon lamps, and LED lights. Each has its advantages.
- Mercury Vapor Lamps: These are common due to their high intensity and broad-spectrum light. They can excite a wide range of fluorescent dyes, including GFP. However, they generate a lot of heat and have a limited lifespan.
- Xenon Lamps: Similar to mercury lamps in intensity, xenon lamps offer a continuous spectrum. They are ideal for applications requiring multiple fluorescent labels. The downside is that they can be quite expensive.
- LED Lights: LEDs are increasingly popular. They are energy-efficient and have a long lifespan. LEDs can be tuned to specific wavelengths, allowing precise excitation for different fluorophores.
Choosing the right light source depends on the specific requirements of the experiment. Factors such as intensity, stability, and spectral output should be considered. Proper selection enhances signal quality and can minimize photobleaching of the samples.
Importance of Optical Filters
Optical filters play a vital role in fluorescence microscopy. They serve to select the appropriate wavelengths of light for excitation and emission, ensuring the specific detection of the fluorescence signal from the sample. Two main types of filters are typically used: excitation filters and emission filters.
- Excitation Filters: These filters allow only the wavelengths needed to excite the fluorescent dye to pass through. Any unwanted wavelengths that could lead to background noise are blocked. This enhances the clarity of the fluorescence signal.
- Emission Filters: After the sample fluoresces, emission filters only permit the desired wavelengths to reach the detector. This is crucial to reduce crosstalk between different fluorescent signals, which can occur when multiple dyes are used.
Overall, the careful selection and application of optical filters significantly influence the quality of the images produced. High-performance filters contribute to better resolution and contrast in the resulting images, thereby aiding in accurate interpretations of the biological samples.
"The effectiveness of fluorescence microscopy heavily relies on the synergy between the light source and optical filters. Properly optimized components lead to enhanced imaging capabilities."
In summary, both light sources and optical filters are indispensable in fluorescence microscopy. They ensure that the right light reaches the sample while effectively capturing the emitted fluorescence. This results in clearer, more precise images that are essential for meaningful analyses in biological research.
GFP Excitation Filters: A Technical Overview
GFP excitation filters play a crucial role in fluorescence microscopy. They selectively allow specific wavelengths of light to pass through, targeting the excitation range of the Green Fluorescent Protein. Without these filters, achieving clear and focused images of fluorescent samples would be difficult.
The primary function of excitation filters is to reject wavelengths that do not match the absorption spectrum of GFP. This specificity enhances the brightness and clarity of images. In turn, researchers can detect even the faintest signals emitted from fluorescently labeled specimens. This capability is essential for many biological research applications, such as studying cellular processes and protein interactions.
Functionality and Applications
Excitation filters are integral to fluorescence microscopy systems. They work by blocking unwanted light while passing the desired excitation wavelengths. Typically, the excitation light source emits a broad spectrum. This light needs filtration to isolate wavelengths optimal for GFP excitation, usually around 400 - 480 nm.
This filtration ensures that only the desired excitation light reaches the specimen. As a result, the emission from the neon-green light of GFP is maximized. Researchers use this filtered light in various applications, like subcellular localization, tracking dynamic processes in live cells, and analyzing genetic expressions.
Beyond basic functionality, the application of excitation filters extends into advanced techniques like spectral imaging. Here, multiple fluorescent proteins are imaged simultaneously. The excitation filters can be tailored to effectively manage the different excitation ranges of diverse fluorophores, achieving better specificity and resolution in complex experiments.
Selection Criteria for Excitation Filters
Choosing the right excitation filter is vital for effective imaging. Researchers consider several criteria before making specific selections:
- Wavelength range: Analyze the spectral properties of the GFP and ensure that the chosen filter matches its excitation peak.
- Transmission Efficiency: High transmission efficiency is crucial. The filter should allow maximum light to pass through while blocking other wavelengths.
- Quality of Coating: The quality of the optical coating on the filter affects light transmittance and reflections. High-quality coatings reduce stray light, improving image quality.
- Material and Build: The construction of the filter matters. Glass filters generally offer better durability and stability compared to plastic options.
"Proper selection of excitation filters is fundamental in fluorescence microscopy, directly affecting experimental outcomes and data validity."
In summary, the efficacy of GFP excitation filters greatly enhances the potential of fluorescence microscopy. They not only improve signal strength and quality but also broaden the scope of applications in biological research.
GFP Emission Filters: Importance in Imaging
GFP emission filters play a crucial role in enhancing the quality of images obtained through fluorescence microscopy. Without these filters, the effectiveness of detecting and analyzing specific fluorescent signals is significantly diminished. Their primary function is to selectively transmit light emitted by the fluorescent probe, such as GFP, while blocking out other wavelengths that may cause interference. This selectivity is essential for clear and precise imaging, enabling researchers to draw accurate conclusions from their experiments.
Effective use of emission filters can lead to several advantages. First, they improve the signal-to-noise ratio in images. By filtering out unwanted wavelengths, emission filters enhance the visibility of the target signals in the presence of background fluorescence or other light interference. This increased clarity is vital in applications involving low-abundance signals. Additionally, the right emission filter can mitigate issues related to spectral overlap and crosstalk, which commonly occur when multiple fluorophores are used in the same experiment. By implementing appropriate filtering techniques, they ensure that data is both reliable and interpretable.
It’s noteworthy that the choice of emission filter directly impacts the overall quality of fluorescence microscopy results. A poorly selected filter may result in misleading interpretations of cellular structures or functions.
Principles of Emission Filtering
The fundamental principles behind emission filtering lie in their ability to isolate specific wavelengths of light based on the emission spectrum of the fluorophore. When GFP is excited at a certain wavelength, it emits light in the green spectrum. Emission filters are designed to transmit this green light while absorbing or reflecting other wavelengths.
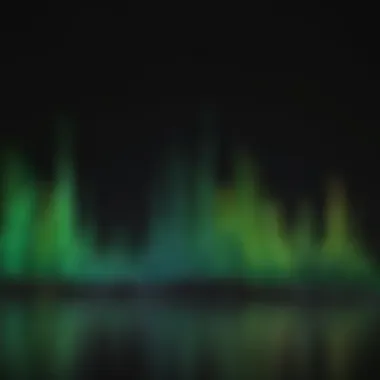
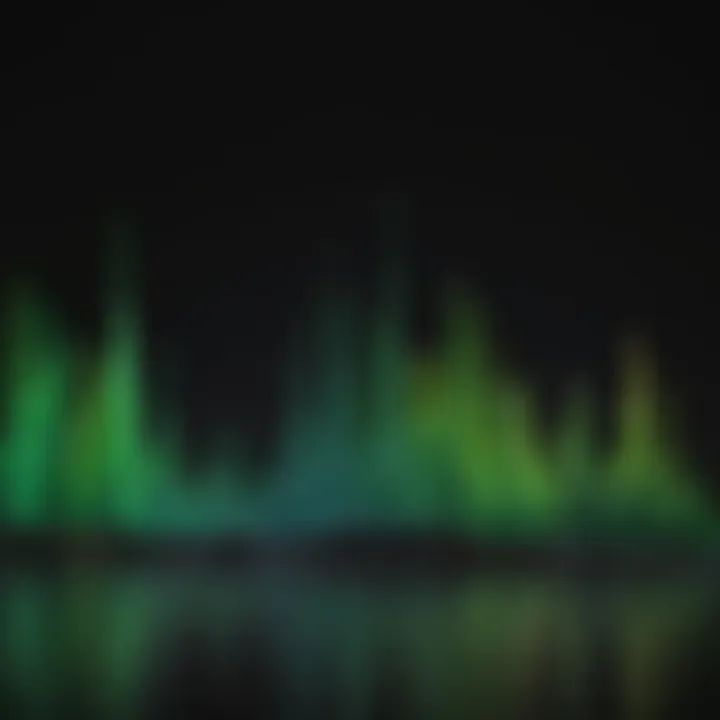
The bandwidth of the emission filter is a critical consideration. A narrower bandwidth allows for more selective resolution of the target fluorescence, leading to cleaner images. Conversely, a broader bandwidth might allow more wavelengths to pass through, risking the introduction of unwanted signals into the image. This balance between sensitivity and specificity defines the choice of filters in various experimental setups.
Furthermore, researchers must take into account the quantum yield of the fluorophore and the efficiency of the filter. An efficient emission filter enhances the emitted signal, while a low efficiency can lead to decreased image quality. The positioning of the filter within the optical pathway also has implications for signal integrity and image resolution.
Best Practices for Emission Filter Use
To optimize the performance of GFP emission filters in fluorescence microscopy, certain best practices can be employed. These practices include:
- Choose Appropriate Filters: Select emission filters that match the peaks of fluorescence emission from GFP or other probes used in the study.
- Regular Calibration: Regularly maintain and calibrate filters to ensure optimal performance. Dust or scratches can significantly degrade image quality.
- Use Complementary Filters: When working with multiple fluorescent probes, use filters that minimize spectral overlap to maintain clarity in multiparametric imaging.
- Test Conditions: Before beginning extensive testing, conduct preliminary tests to evaluate filter performance under specific experimental conditions.
- Monitor Signal Levels: Ensure proper signal levels are achieved by adjusting light intensity and employing suitable gain settings, making necessary adjustments based on filter performance.
Following these best practices not only enhances the reliability of images obtained from fluorescence microscopy but also contributes to the overall robustness of the research findings.
Effects of Filter Selection on Image Quality
The role of filter selection in fluorescence microscopy cannot be underestimated, especially when working with Green Fluorescent Protein (GFP). Filters dictate which wavelengths of light are allowed to reach the detector, consequently influencing the overall image quality of the samples being observed. It is crucial to choose the right filters based on the specific requirements of the experiment. Selecting inappropriate filters can lead to poor image quality, which can compromise research results.
Impact on Signal-to-Noise Ratio
The signal-to-noise ratio (SNR) is a key element in the evaluation of imaging performance. SNR represents the level of the desired signal compared to the background noise. In GFP applications, high SNR is necessary for distinguishing the fluorescence signal from background interference.
A well-chosen excitation filter allows only the relevant wavelengths of light to excite the GFP, thus increasing the fluorescence signal. Conversely, emission filters need to effectively block any unwanted wavelengths that could contribute to noise.
Considerations for improving SNR through proper filter selection include:
- Filter bandwidth: Narrower bandwidth often boosts SNR by selectively allowing only specific wavelengths to pass.
- Quality of the filter material: High-quality filters ensure minimal scattering and absorbance, reducing background noise.
Using inappropriate filters can reverse the impact by allowing extraneous wavelengths, thus increasing noise. For instance, an excitation filter that does not sufficiently reject the near-infrared light may induce additional background, interfering with the perception of the GFP signal.
Influence on Spectral Overlap and Crosstalk
Spectral overlap occurs when the emission spectra of different probes or fluorescent labels overlap with one another, creating challenges in separating signals. This overlap can lead to crosstalk, wherein signals from one fluorophore affect the detection of another. In fluorescence microscopy involving GFP, careful consideration of the emission filters is essential to mitigate these issues.
Factors to consider for minimizing spectral overlap and crosstalk include:
- Choosing complimentary filters: Filters that closely match the peak emission spectra of GFP while excluding other signals can enhance clarity.
- Leveraging sophisticated filter sets: Advanced systems with narrow band-pass filters specifically aligned with GFP can reduce overlap, enhancing image fidelity.
A poorly selected emission filter can contribute heavily to spectral overlap, resulting in crosstalk that obscures critical details in experimental imaging.
In summary, the right filter selection is pivotal in fluorescence microscopy for ensuring high-quality images. Researchers must carefully assess factors like signal-to-noise ratio and potential spectral overlap in their choice of filters to achieve accurate imaging results.
Recent Advances in Filter Technology
Importance of Recent Advances in Filter Technology
Advancements in filter technology play a crucial role in the effectiveness of fluorescence microscopy, specifically when working with Green Fluorescent Protein (GFP). These innovations enhance image clarity and reliability, providing sharper and more detailed visualizations of biological samples. New materials and manufacturing techniques improve filter precision, which in turn contributes to better overall performance in microscopy applications. This progress is essential for researchers who require high-quality imaging and accurate data for their studies.
Innovations in Excitation Filters
Recent developments in excitation filters have introduced materials that efficiently transmit specific wavelengths while blocking unwanted light. This innovation is critical for maximizing the excitation of GFP, thereby improving fluorescence intensity.
Some notable advancements include:
- Advanced Coating Techniques: New multilayer coatings have been developed that further refine the transmission spectrum. These coatings help in reducing background noise, allowing for clearer images.
- Broadband Filters: The introduction of broadband excitation filters allows for more flexibility in imaging protocols. This blending of different wavelengths can improve versatility in applications, enhancing the potential to visualize multiple markers.
- Smart Filters: These adaptive filters can automatically adjust their characteristics based on the environmental conditions or specific experimental conditions. This innovation facilitates real-time optimizations that can lead to superior imaging outcomes.
Emerging Trends in Emission Filtering
Emerging trends in emission filtering are also shaping the future of fluorescence microscopy. Innovations are making these filters more efficient and tailored for specific applications. Key trends include:
- Nano-Optic Filters: Utilizing nanotechnology has enabled the creation of ultra-thin filters that do not compromise optical quality. These filters provide excellent light transmission while being lightweight and easy to use.
- Spectral Imaging: Emission filters are increasingly used in spectral imaging systems, where capturing detailed spectral data becomes feasible. This trend allows for more precise identification of multiple fluorescence signals from different probes.
- Dynamic Filters: The use of digital light processing to create dynamic filters enhances control over the wavelengths that are allowed through. This provides unprecedented flexibility in selecting the emission spectrum appropriate for various experimental setups.
Practical Considerations for Filter Use
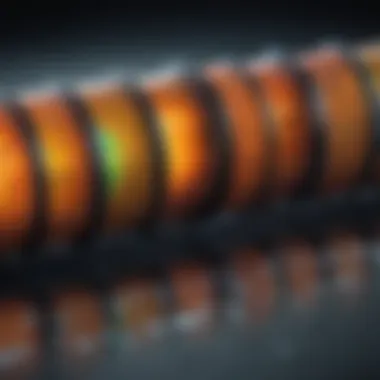
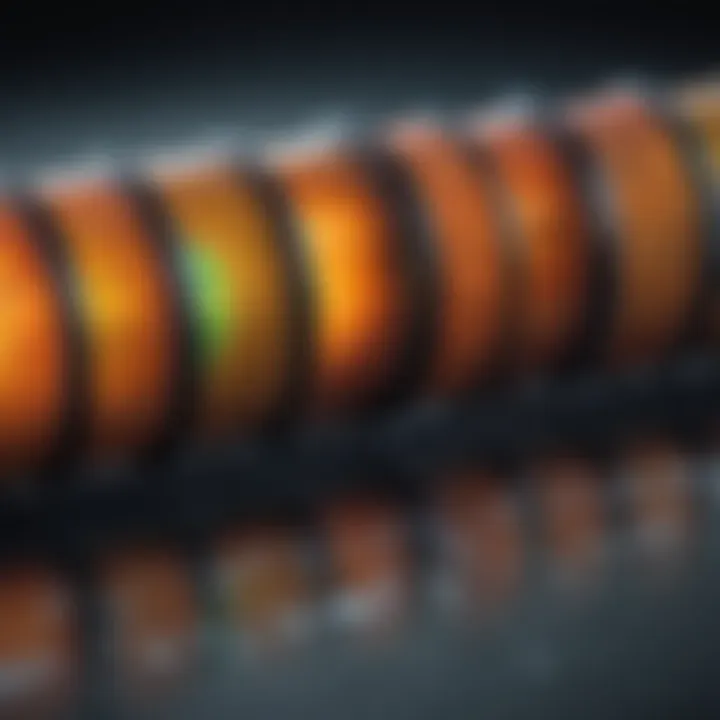
In fluorescence microscopy, the role of excitation and emission filters is pivotal. Understanding practical aspects of filter use ensures optimal imaging results. Practical considerations involve filter choice, mounting techniques, and maintenance. Whether one is new to these methods or has considerable experience, knowledge of these factors is critical for success.
Setting Up a Fluorescence Microscope
The initial setup of a fluorescence microscope is crucial for achieving quality images. Proper arrangement of the excitation and emission filters must be done carefully. Start by ensuring that excitation filters align correctly with light sources. This alignment maximizes the light intensity directed at the GFP.
Next, the emission filters must be placed to capture the desired fluorescence light, discarding other wavelengths. Following the manufacturer’s specifications, users should inspect the filter cube assembly. The cube should include the correct filters, a beam splitter, and optics.
Utilizing a fluorescence microscope requires careful adjustments. The specific settings of objective lenses and sample positioning also are important. Attention should be given to the focus and illumination settings. If done correctly, these steps lead to clearer imaging of the GFP in samples, promoting better scientific conclusions.
Maintaining and Calibrating Filters
After setting up the microscope, maintaining and calibrating the filters is essential. Clean filters to prevent dust and contamination that can distort results. Use appropriate solvents and lint-free materials during cleaning. The routine maintenance extends the life of filters and improves performance.
Calibrating filters is another important task. This helps in optimizing the imaging process. Researchers should conduct routine checks on filter efficiency, especially before critical experiments. It ensures that the emission and excitation spectra remain within the expected limits. Failure to calibrate can lead to poor signal quality and misinterpretations.
"Regular maintenance and calibration of filters can significantly enhance imaging quality in fluorescence microscopy."
Proper calibration practices include using standard reference samples. This helps in confirming the accuracy of the filter wavelengths. Adhering to a maintenance schedule not only improves microscope performance but also fosters consistent results in research.
Case Studies: Filter Applications in Research
The application of GFP in various fields of research emphasizes the crucial role that excitation and emission filters play in fluorescence microscopy. These filters are not simply accessories; they directly influence the clarity and integrity of the images captured. The cases discussed below provide insights into how filter applications can yield significant outcomes in specific research contexts. They highlight both the benefits of proper filter selection and the considerations essential for effective research methods.
GFP in Cell Biology
GFP has revolutionized cell biology by enabling researchers to visualize cellular processes in real-time. When examining cell dynamics, precise filter settings are critical. Excitation filters allow only the wavelengths necessary to activate the GFP molecules, while emission filters ensure that only the fluorescent signal emitted by the GFP is captured. This specificity is particularly important in studies of protein localization and gene expression.
Researchers have shown that optimizing filter combinations directly affects image quality and accuracy in studies involving complex cellular interactions. For instance, in experiments involving live-cell imaging, an appropriate excitation filter enhances the signal, reducing background noise and improving signal-to-noise ratios.
Key Benefits:
- Enhanced visualization of cellular structures.
- Insight into dynamic processes such as cell division, migration, and apoptosis.
- Ability to track multiple proteins if appropriate filters are selected.
"Utilizing the right filters significantly improves our ability to interpret biological phenomena at the cellular level."
Furthermore, some studies have demonstrated that mismatched filters can lead to misleading results. For example, using an excitation filter that allows additional wavelengths can result in crosstalk and decreased specificity. Thus, careful filter selection in GFP applications is not merely technical but profoundly impacts scientific conclusions.
Environmental Monitoring with GFP
The employment of GFP extends beyond cell biology into environmental monitoring, particularly in assessing the health of ecosystems. Organisms engineered to express GFP can serve as biosensors for detecting pollutants. The successful application hinges on using the appropriate filters during microscopy to ensure accurate readings of fluorescence intensity.
In these studies, specific excitation and emission filters capture the fluorescence from GFP-expressing organisms. Environmental conditions can alter the fluorescence, causing variations that need precise capturing and interpretation. As such, filter choice significantly influences the reliability of environmental monitoring studies. Researchers must be aware of potential disturbances from background fluorescence and ensure the filters chosen are effective in minimizing interference.
Key Benefits:
- Real-time monitoring of environmental health through living organisms.
- Ability to assess the impact of pollutants in natural habitats.
- Contribution to conservation efforts by tracking organism health.
In summary, case studies illustrate that the choice of GFP excitation and emission filters is not negligible. The impacts are profound across various research areas. These filters enable researchers to obtain clearer, more detailed imagery, leading to more insightful conclusions. As the use of GFP continues to grow in scientific endeavors, understanding filter applications will remain an essential competency for researchers.
The End
The conclusion plays a vital role in synthesizing the key points discussed in this article concerning GFP excitation and emission filters in fluorescence microscopy. This final section encapsulates the significance of filter selection and its direct impact on imaging results.
Through detailed analysis, we have established that the right excitation and emission filters not only enhance the visibility of fluorescent signals but also ensure precise differentiation between overlapping spectra. These filters enable researchers to capture more accurate and reliable results, which is crucial for advanced studies in cell biology and various environmental monitoring applications.
Moreover, the importance of ongoing improvements in filter technology cannot be overstated. This aspect is essential for adapting to the evolving needs of microscopy techniques, ensuring that researchers remain at the forefront of discovery. Filters must not only fit well within current methodologies but also anticipate future requirements.
Summary of Key Points
- Key Components: GFP excitation and emission filters are essential for optimizing fluorescence microscopy.
- Signal Quality: Proper filter selection improves signal-to-noise ratios, reducing background interference.
- Technological Advances: Recent innovations in filter technology have broadened the capabilities in fluorescence imaging.
- Practical Considerations: Researchers must consider the setup, maintenance, and calibration of filters to achieve optimal performance.
Future Directions in GFP Imaging
Future advancements in GFP imaging will likely focus on the integration of novel filter designs and materials that can better facilitate multi-spectral imaging. As biological research introduces more complex fluorescent markers, there will be a growing need for filters capable of filtering multiple wavelengths without crosstalk. Continuous development in optics will also emphasize the reduction of light loss and enhancement of transmission efficiency.
In addition, the incorporation of artificial intelligence and machine learning into imaging analysis may lead to more intelligent filter selection processes, adapting to specific samples in real-time. Such innovations promise to refine imaging quality and efficiency further, propelling the field of fluorescence microscopy into new realms of possibility.