Comprehensive Insights into Polymer Production Techniques
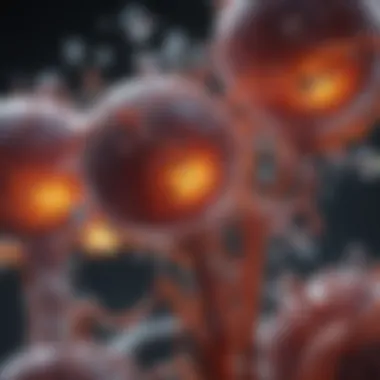
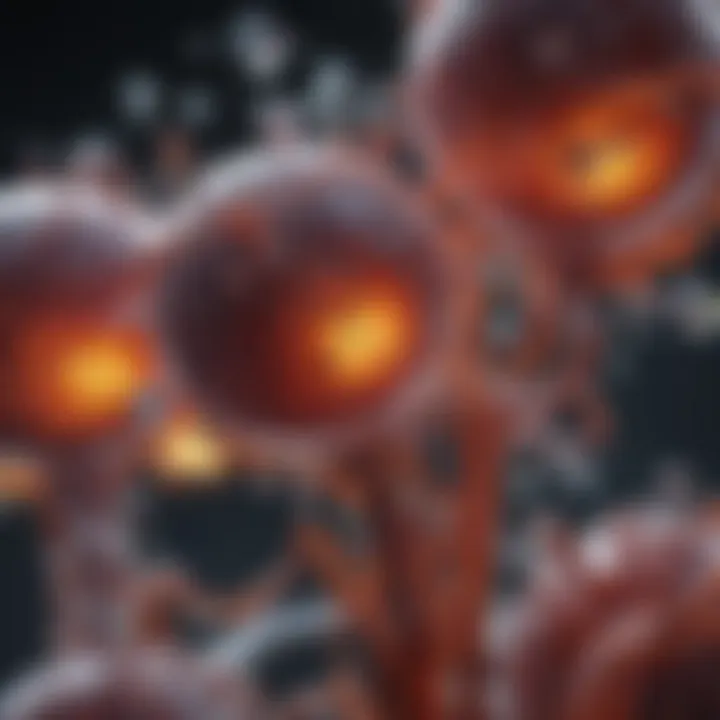
Intro
Polymer production stands as one of the vital components in modern material science. With vast applications ranging from everyday household items to advanced industrial tools, understanding polymer synthesis is crucial. This analysis aims to systematically unveil the intricate processes involved in polymer production, emphasizing methods, materials, and technologies utilized across various industries. Moreover, attention will be given to the environmental implications tied with polymer manufacturing, prompting critical discussions on sustainability practices.
Research Overview
Summary of Key Findings
In the exploration of polymer production, several important points emerge:
- Methods of Production: Traditional methods, such as addition and condensation polymerization, are crucial to understand, alongside modern approaches like biopolymer synthesis and 3D printing.
- Materials Used: The choice of monomers greatly affects the properties of the final product, conversations around the nuances of natural versus synthetic polymers are pivotal.
- Technological Advancements: Innovations, including automated production lines and smart materials, are transforming the landscape of polymer manufacturing.
- Environmental Impact: The analysis addresses the ecological footprint of polymers, discussing recycling technologies and alternative biodegradable options.
"Polymers hold an essential position in the material hierarchy; understanding their production is paramount to advancing materials science."
Importance of the Research
The relevance of studying polymer production cannot be overstated. With the increasing demand for sustainable practices, researchers need awareness of how production methods affect the environment. While polymers are integral to numerous applications, understanding their lifecycle fosters responsibility and innovation in production techniques. Furthermore, for students and professionals in the field, this research provides a solid foundation, encouraging informed discussions about future advancements in polymer science.
Methodology
Study Design
The methodology employed in this analysis is comprehensive, covering both qualitative and quantitative aspects of polymer production. A systematic review of literature serves as the primary foundation, supplemented by case studies highlighting real-world applications of various polymers.
Data Collection Techniques
Data collection involves:
- Literature Review: Exploring published works, from peer-reviewed journals to industry reports.
- Case Studies: Analyzing documented instances of polymer production to illustrate practical implications and innovations.
- Surveys: Gathering insights from industry professionals to understand current challenges and advancements witnessed in polymer production.
Through this structured approach, the analysis aims to provide an in-depth understanding of polymer production, paving the way for informed discussions and future research initiatives.
Prelude to Polymer Production
Polymer production plays a crucial role in modern material science and engineering. This section serves as a gateway to understanding the fundamental aspects of polymers, which influence various industries, from packaging to biomedical applications. The importance of this topic cannot be understated; polymers are integral to everyday life and have led to significant advancements in technology and innovation.
Definition and Importance
Polymers are large molecules composed of repeating structural units called monomers. These compounds are significant due to their versatile properties that can be tailored for numerous applications. The flexibility, durability, and variability in the synthesis of polymers allow them to be used in many sectors, enhancing their importance.
The significance of polymer production can be observed through several factors:
- Economic Impact: The polymer industry contributes substantially to global economies through job creation and sustainability initiatives.
- Technological Advancements: Innovations in polymer chemistry have propelled research and development, leading to better materials and applications.
- Environmental Concerns: Understanding polymer production helps address the environmental challenges associated with plastic waste and encourages the development of biodegradable options.
Overview of Polymer Types
Polymers can be classified into various categories based on their origin, molecular structure, and thermal properties. Understanding these types is essential for comprehending their vast range of applications. Here are a few notable categories:
- Natural Polymers: These are polymers that occur naturally, such as cellulose, proteins, and rubber.
- Synthetic Polymers: Man-made polymers include polyethylene, polystyrene, and nylon, which are widely used in various applications.
- Thermoplastics and Thermosets: Thermoplastics can be remolded through heat, while thermosets form irreversible bonds when heated initially, making them more rigid.
These classifications offer insight into how polymers can be utilized effectively in different fields, from consumer products to high-performance engineering applications. Understanding these aspects sets the foundation for deeper discussions regarding polymerization processes and material properties, which are covered in the subsequent sections.
The Chemistry of Polymers
The chemistry of polymers is fundamental to understanding how these materials are produced and utilized across various industries. This section delves into essential concepts like monomers and the processes that convert them into complex polymer structures. A solid grasp of the chemical principles involved is vital for researchers and professionals seeking to optimize production and innovate within the field.
Monomers and Polymerization
Monomers are the building blocks of polymers. They are small, organic molecules that can bond together, forming long chains known as polymers through various reactions. The significance of monomers lies in their structural diversity, which enables the synthesis of an array of polymer types, each with unique properties and applications. For instance, ethylene and propylene are common monomers that lead to polyethylene and polypropylene, respectively.
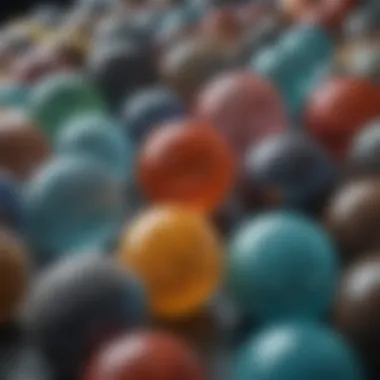
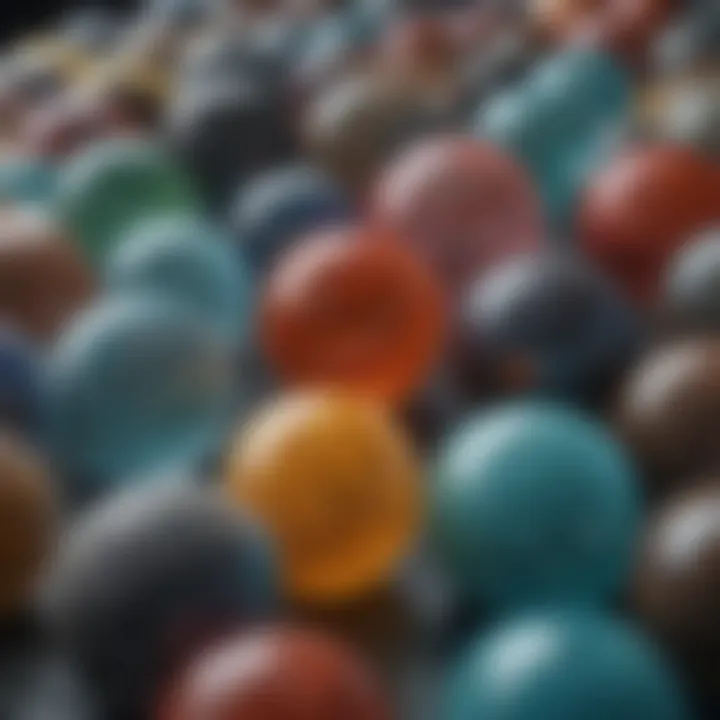
Polymerization is the chemical process where monomers are chemically bonded into larger structures. There are two main types of polymerization: addition and condensation. Understanding these mechanisms is crucial for the production of specific polymers and affects the properties of the resultant materials.
Types of Polymerization Processes
Polymerization processes can be classified mainly into three types: addition polymerization, condensation polymerization, and copolymerization. Each method has unique features and benefits that affect production efficiency and material properties.
Addition Polymerization
Addition polymerization is a process that involves the linking of monomers that contain double bonds. A key characteristic of this method is that it does not produce any by-products, making it a clean process. This is beneficial for industries focused on reducing waste and enhancing efficiency.
Moreover, addition polymerization allows for swift reactions and can produce high molecular weight polymers relatively easily. Its unique feature is the ability to control the molecular weight and structure of the polymer through varying reaction conditions. The advantages of this process include rapid production rates and the ability to create diverse polymer types efficiently. However, some limitations, like the requirement for specific monomers and conditions, may affect its application in certain contexts.
Condensation Polymerization
Condensation polymerization involves the reaction between two different types of monomers. A significant aspect of this method is that it often results in the release of a small molecule, such as water or alcohol, during the polymer formation. This offers different chemical characteristics that can be exploited for specific applications.
A notable characteristic of condensation polymerization is the diversity of reaction types and products it can achieve. This can lead to polymers with enhanced thermal and mechanical properties. The advantages of this method include the ability to create complex structures, but it may take longer to reach completion compared to addition polymerization. The presence of by-products can also complicate the process.
Copolymerization
Copolymerization refers to the process of combining two or more different types of monomers to form a polymer. This approach allows for greater flexibility in tailoring polymer properties for specific applications. One main characteristic of copolymerization is its ability to blend the properties of different monomers, resulting in materials that can exhibit a combination of traits, such as improved toughness or thermal stability.
This method is particularly beneficial as it expands the range of possible polymers that can be synthesized. The unique feature of copolymerization is its versatility, as it can enhance specific properties that are desirable in various applications. However, its complexity in balancing multiple monomers might pose challenges in the production process.
Overall, understanding these polymerization processes adds depth to the discussion of polymer chemistry. It allows for better control of material properties and informs production methodologies that can impact the polymer industry significantly.
Materials Used in Polymer Production
In analyzing polymer production, the materials utilized play a fundamental role. Both the origin of the materials and their properties significantly influence the performance of the resulting polymers. Understanding the distinction between natural and synthetic polymers is essential, as this classification affects everything from production methods to applications and sustainability.
Natural vs Synthetic Polymers
Natural polymers have been used for centuries. They are derived from biological sources, such as plants and animals. Examples include cellulose, proteins, and natural rubber. They are often biodegradable and can be more environmentally friendly. However, their availability can be limited, and their properties may vary based on the source.
Conversely, synthetic polymers are man-made and consist primarily of petrochemicals. These materials include polyethylene, polystyrene, and nylon. They usually provide more consistent properties and can be tailored for specific applications. However, synthetic polymers often come with concerns about environmental impact and their dependence on fossil fuels.
Key Differences:
- Source: Natural polymers come from living organisms, while synthetic polymers are chemically manufactured.
- Properties: Synthetic polymers can be engineered for better performance, whereas natural polymers can have inherent variability.
- Environmental Impact: Natural polymers tend to be more biodegradable; synthetic options are known for durability but raise concerns regarding pollution and waste.
Common Monomers in Industry
Monomers serve as the building blocks for polymer production. A wide variety of monomers are utilized in the industry, each contributing unique characteristics to the polymer. Understanding common monomers is vital for researchers and practitioners, as they determine the properties of the produced polymers.
Common monomers include:
- Ethylene: Used to create polyethylene, one of the most widely used plastics.
- Propylene: The basis for polypropylene, known for its toughness and flexibility.
- Styrene: A key component of polystyrene, widely used in packaging and insulation.
- Vinyl Chloride: Used to produce PVC, known for its versatility in construction and plumbing.
These monomers can be used alone or in combination to create copolymers, which may exhibit improved qualities or a balance of properties.
"The choice of monomers directly impacts the polymerโs mechanical properties, thermal stability, and chemical resistance."
Techniques and Technologies
The realm of polymer production is fundamentally dependent on various techniques and technologies that facilitate the creation of diverse materials. The efficiency of these methods can greatly influence the quality, cost, and impact of the final polymer products. Understanding the different approaches used in polymer synthesis is essential for students, researchers, and professionals aiming to innovate or improve existing practices.
Batch vs Continuous Production
In polymer production, two primary processes are distinguished: batch and continuous production. Each offers unique advantages based on the desired output and application.
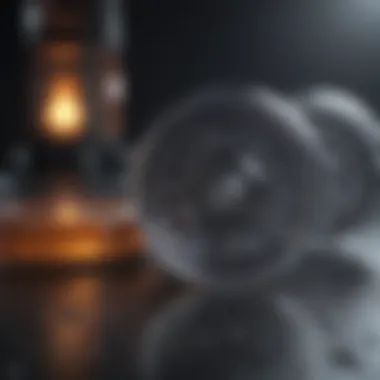
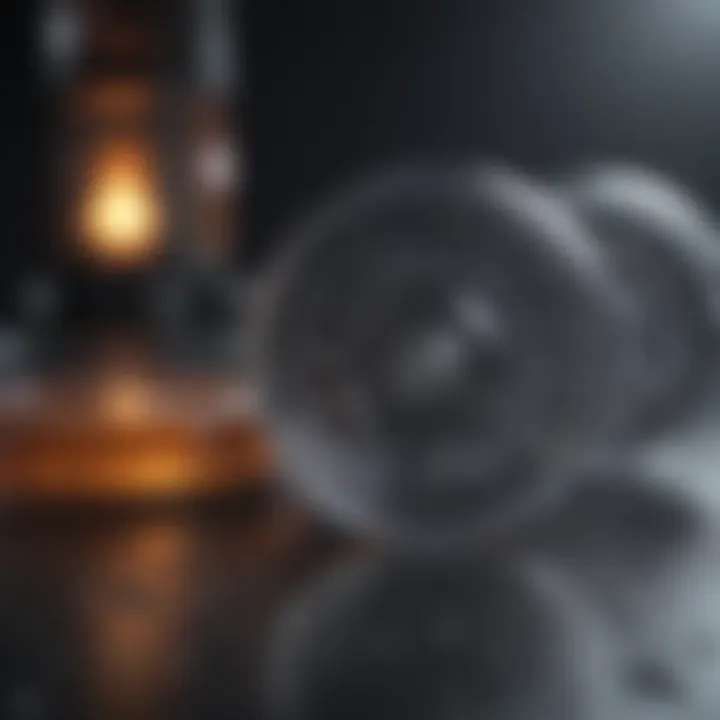
Batch production involves processing a specific quantity of material in one cycle. This method is often preferred for producing customized or small-scale polymer batches. Key advantages include:
- Flexibility to change formulations easily.
- Reduced risk of contamination due to isolated processing.
- Ideal for producing specialty polymers.
Nonetheless, batch production can lead to higher labor costs and longer processing times, especially when switching between different polymers. This approach is beneficial when experimentation or variability in production is desired.
In contrast, continuous production systems allow for the uninterrupted synthesis of polymers. Materials feed into the system continuously, producing a steady stream of output. This method is advantageous for large-scale production and has several benefits:
- Increased efficiency and reduced production costs per unit.
- Consistent product quality due to stable conditions.
- Real-time monitoring and adjustments to optimize output.
However, continuous systems are less flexible. Changing the polymer type or formulation can involve significant downtime, which may not be suitable for producers with fluctuating customer demands. Many industries weigh these factors carefully to determine the right balance based on their specific needs.
Emerging Technologies in Polymer Production
The field of polymer production is continuously evolving. Emerging technologies are crucial for enhancing production efficiency and reducing environmental impact. Several noteworthy advancements include:
- Microfluidics enables precise control over polymerization processes at a microscale, allowing for better product uniformity.
- 3D printing of polymers provides new ways to fabricate complex structures with custom designs, reducing waste.
- Biotechnology plays a significant role, especially in developing biodegradable polymers. Using bio-based feedstocks can help address sustainability concerns.
Additionally, automation and artificial intelligence are transforming production lines. These technologies improve monitoring, predictive maintenance, and process optimization, which can significantly enhance output quality and operational efficiency.
Overall, the adoption of these technologies represents a prominent shift in polymer production practices, positioning industries to better respond to market dynamics and environmental challenges. As research progresses, these methods will likely become more refined and integrated into standard production protocols, shaping the future landscape of polymer materials.
Applications of Polymers
Polymers have become an integral part of various industries and everyday life. This section examines the significance of polymers in real-world applications, highlighting their unique properties that contribute to advancements in different fields. Understanding these applications gives insight into how polymers enhance functionality, efficiency, and sustainability.
Packaging Industry
The packaging industry heavily relies on polymers due to their versatility and ability to be molded into various shapes. Materials like polyethylene and polypropylene are commonly used, providing durability and flexibility. These polymers protect products from contamination and damage while extending shelf life.
The benefits of polymer packaging include:
- Lightweight construction: Reducing transportation costs.
- Barrier properties: Protecting contents from moisture and oxygen.
- Customization: Allowing for design flexibility in branding and functionality.
The move towards sustainable packaging has led to the rise of biodegradable polymers. These materials are designed to break down more quickly than traditional plastics, reducing environmental impact. Innovations in bioplastics provide greener alternatives, meeting consumer demand for sustainability.
Biomedical Applications
In the biomedical field, polymers are essential for creating medical devices, drug delivery systems, and tissue engineering scaffolds. Biocompatible polymers, like polylactic acid and polycaprolactone, are widely used due to their compatibility with biological systems.
Key areas of application include:
- Implants: Used in stents and orthopedic devices.
- Controlled drug release: Enhancing the effectiveness of therapies.
- Tissue scaffolds: Supporting cell growth for regenerative medicine.
These applications show how polymers aid in improving patient outcomes and advancing medical technology. Research in this domain continues to explore new polymer types that can further enhance efficacy and reduce risks.
Electronics and Conductive Polymers
Polymers are increasingly being used in electronics, particularly conductive polymers. These materials possess unique electrical properties that make them ideal for various applications, such as sensors, organic light-emitting diodes, and photovoltaic cells.
Notable benefits of using conductive polymers include:
- Flexibility: Allowing for the design of lightweight and bendable devices.
- Cost-effectiveness: Cheaper manufacturing compared to traditional metal components.
- Enhanced performance: Offering high conductivity and efficiency.
As electronics continue to evolve, the role of conductive polymers is expanding. Innovations in this field could lead to solutions for challenges in energy storage and smart materials.
The applications of polymers span numerous industries, showcasing their adaptability and importance. As research continues, the potential for new uses and improvements will likely pave the way for further developments in polymer science.
Understanding the role of polymers in various applications informs future innovation and environmentally sustainable practices.
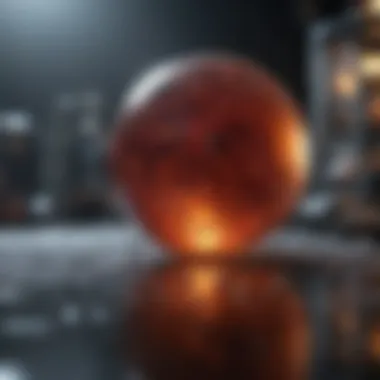
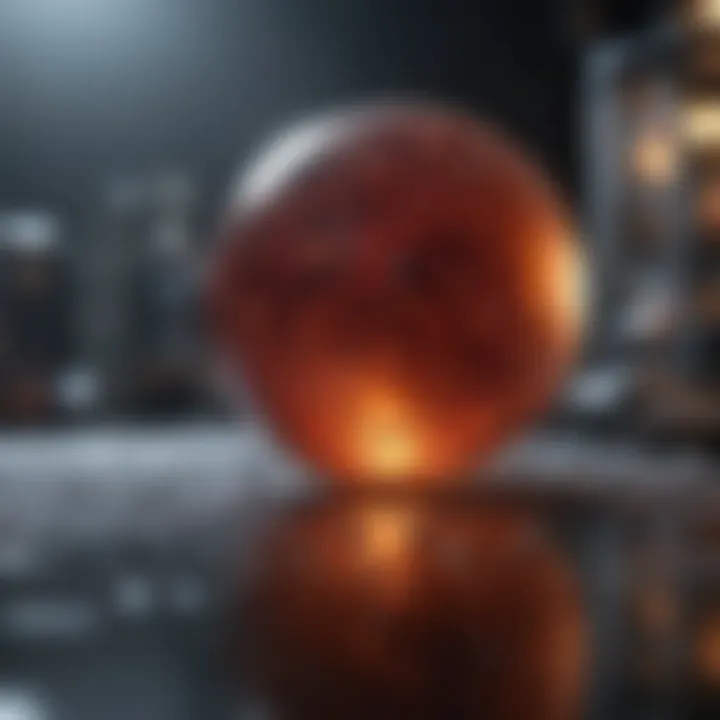
Environmental Considerations
Environmental considerations in polymer production have become increasingly important as the world grapples with the consequences of plastic pollution and climate change. The production of polymers can significantly impact ecosystems and human health through emissions, resource extraction, and waste generation. Addressing these impacts allows for more responsible practices that are essential for a sustainable future.
Sustainability in Polymer Production
Sustainability aims to meet the needs of the present without compromising the ability of future generations to meet their own needs. In polymer production, this involves minimizing resource use, reducing energy consumption, and ensuring that the life cycle of materials results in minimal environmental damage.
One key approach is the use of renewable resources in the creation of polymers. Materials like corn starch and sugar can serve as alternatives to traditional petroleum-based monomers. Another important aspect is improving production processes. For example, optimizing reaction conditions can reduce energy inputs and waste outputs.
Additionally, companies are increasingly adopting circular economy principles. This means designing polymers with end-of-life considerations. Materials can be created to be recyclable or reusable, which helps in reducing landfill waste. Initiatives such as closed-loop recycling can further diminish the demand for new raw materials.
Biodegradable Polymers
Biodegradable polymers offer a promising solution to the challenges posed by plastic waste. These materials are designed to break down more quickly than conventional plastics, typically through microbial processes. They help to mitigate the long-lasting impact of traditional plastics that can remain in the environment for hundreds of years.
Common biodegradable polymers include polylactic acid (PLA) and polyhydroxyalkanoates (PHA). These materials can serve a variety of applications in packaging, agriculture, and even textiles. However, the efficacy of biodegradable polymers relies heavily on specific conditions such as temperature, moisture, and microbial activity.
It is important to note that not all biodegradable polymers are created equal. Their production and end-of-life behavior must be optimized to ensure true environmental benefits. Thus, ongoing research and development are crucial for improving performance and reducing the ecological footprint of biodegradable polymers.
"Transitioning to sustainable and biodegradable polymers is not just an environmental choice, it's an economic necessity for future industries."
Future Trends in Polymer Production
The landscape of polymer production is under constant evolution. Future trends indicate a shift towards more sustainable practices, advanced technologies, and innovative materials. This section delves into significant elements of future trends in polymer production, emphasizing their benefits and considerations.
Advancements in Polymer Research
The study of polymers is witnessing rapid advancements. Researchers are increasingly focused on developing new synthesis methods. These methods aim to enhance the efficiency and reduce the environmental footprint of production processes. For instance, nanotechnology is playing a crucial role in polymer research. The incorporation of nanoparticles can lead to stronger and lighter materials. This is particularly beneficial in industries like aerospace and automotive.
Moreover, biopolymer research is gaining traction. Scientists are exploring substances derived from renewable resources. These materials present a viable alternative to conventional, fossil fuel-based polymers. They can decrease carbon emissions and pollution associated with traditional methods.
Another notable trend is the exploration of smart polymers. These materials can respond to environmental changes, offering functionality in various applications. For instance, some polymers change shape or color with temperature shifts, which could revolutionize medical devices and textiles.
Regulatory Changes and Industry Impact
Regulatory frameworks around polymer production are evolving. Governments worldwide are recognizing the need for stricter environmental regulations. This shift impacts how companies operate in the polymer sector. Regulatory changes often promote sustainable practices, such as using biodegradable materials and minimizing waste.
Companies need to adapt their strategies in response to these changes. Compliance can lead to higher production costs, but it also opens up new markets. Sustainable products have a growing demand among consumers aware of environmental issues. Firms that embrace these regulations can position themselves favorably in this competitive landscape.
To summarize, the future of polymer production is likely to be shaped by advancements in research and regulatory adjustments. Companies that prioritize innovation and sustainability may find themselves leading the market.
"Sustainability in production is not just a trend; it is becoming a necessity for survival in the polymer industry."
With these advancements and changes on the horizon, the polymer industry has the potential to transform in ways that enhance product performance while reducing environmental impact.
Epilogue
The importance of the conclusion in this article cannot be overstated. This final section encapsulates the primary insights and knowledge derived from the comprehensive analysis of polymer production. It serves as a synthesis of the intricate details discussed throughout the previous sections, highlighting key methodologies, materials, and technological advances that shape the polymer industry today.
One notable benefit of the conclusion is that it consolidates essential information for readers, allowing them to retain critical aspects of polymer production. It offers clarity on how various polymer types are developed and their applications in diverse fields, which can be particularly beneficial for students, researchers, educators, and professionals engaged in this domain.
Considering the global push towards sustainability, the conclusion also prompts readers to think about the environmental implications discussed in the article. This reflective thought is crucial as the industry undergoes transformation in response to ecological challenges. Furthermore, a strong conclusion emphasizes the necessity for continued exploration and innovation in polymer research, suggesting that progress in this field is indispensable for future applications.
Summary of Key Points
- Diverse Polymer Types: Different classes of polymers serve varying industries, each with its unique synthesis process and application.
- Production Techniques: Both batch and continuous production methods play pivotal roles in ensuring efficiency and quality.
- Environmental Impact: The discussion on sustainability highlights the pressing need for biodegradable options in the polymer landscape.
- Future Innovations: Advances in polymer research indicate a promising horizon for new materials and sustainable practices.
Implications for Future Research
The insights presented in this article open various avenues for future research, particularly in polymer synthesis and application. Researchers should focus on:
- Sustainable Materials: Identifying and creating polymers that are both effective in performance and environmentally friendly could significantly contribute to reducing pollution.
- Clinical Applications: Exploring innovative uses of polymers in biomedical fields can lead to breakthroughs in drug delivery systems and medical devices.
- Adaptation to Regulations: As environmental regulations become more stringent, research into compliance alongside innovation will be critical for industry growth.
- Interdisciplinary Studies: Enhanced cooperation between chemical engineering, materials science, and environmental science can yield revolutionary advancements in polymer production.
In summary, the conclusion of this article not only solidifies the information presented but also encourages further inquiry and innovation in polymer studies, crucial for meeting future demands and challenges.