Exploring the Microwave Transmitter: Structure and Functionality
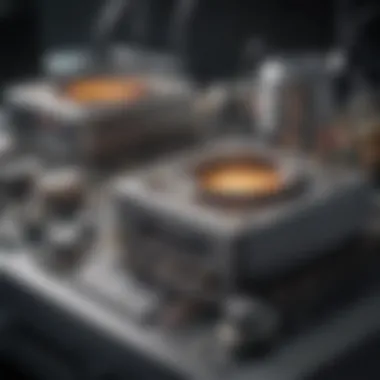
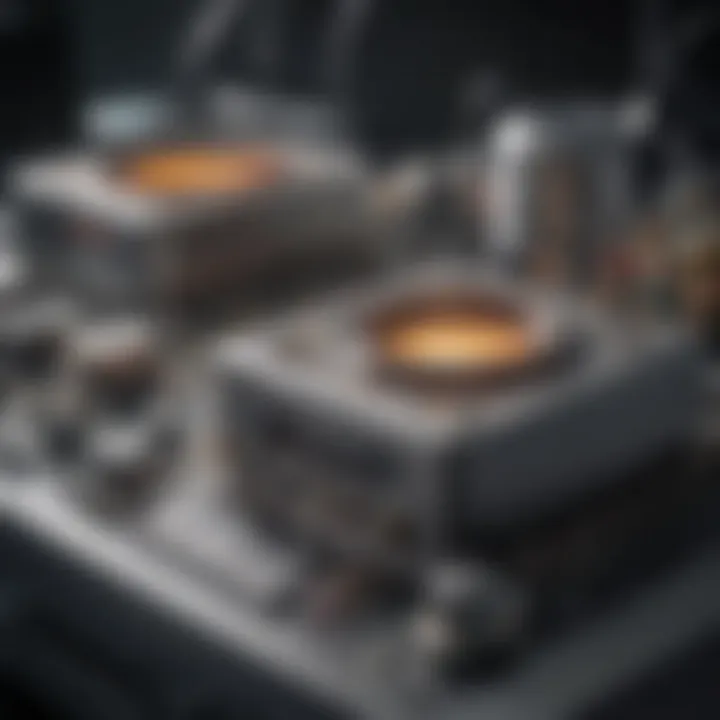
Intro
Microwave transmitters serve as critical components in the landscape of modern communication and scientific applications. These devices operate at microwave frequencies, typically ranging from 1 GHz to 300 GHz, allowing them to facilitate the transmission of data, voice, and video with remarkable efficiency. Understanding the structure and functionality of microwave transmitters can unlock insights into their role across various industries, from telecommunications to radar systems.
Herein, we explore the components that constitute these transmitters, their operational principles, and the diverse applications that leverage their capabilities. Knowledge about microwave transmitters not only enhances our comprehension of contemporary technologies but also provides a foundation for future innovations and advancements.
Research Overview
Summary of Key Findings
This analysis reveals several noteworthy aspects of microwave transmitters:
- Core Components: The primary components of microwave transmitters include the oscillator, modulator, amplifier, and antenna. Each part plays a vital role in signal generation, modulation, amplification, and dispersion.
- Operational Principles: Microwave transmitters operate through the conversion of electrical signals into microwave signals, which are subsequently transmitted over a chosen medium. The modulation techniques employed significantly influence the communication efficacy.
- Applications: These devices find their applications in numerous fields: telecommunications, satellite communications, radar technology, and even in medical imaging systems, showcasing versatility and importance.
Importance of the Research
Understanding microwave transmitters is crucial for professionals and students in fields such as telecommunications, engineering, and applied physics. By delving into their operational structures and potential advancements, researchers can contribute to improving communication technologies and enhancing scientific research methodologies.
"The significance of microwave transmitters extends beyond mere technology; they embody the foundation of modern communications and scientific innovations."
Methodology
Study Design
The exploration involves a comprehensive review of existing literature, technological specifications, and application scenarios surrounding microwave transmitters. This study design integrates theoretical frameworks with practical insights, allowing for a holistic learning experience.
Data Collection Techniques
Data was gathered from multiple sources:
- Academic Journals: Articles from peer-reviewed journals provided in-depth analysis and current trends in technology.
- Industry Reports: Documentation from industry leaders offered valuable perspectives on applications and market demands.
- Online Resources: Websites such as Wikipedia and Britannica revealed historical contexts and definitions that support the subject matter, assisting in building a well-rounded understanding of microwave transmitters.
As we progress through this article, we will scrutinize each key component of microwave transmitters in detail, elucidating their interconnections and role within the broader communication system.
Intro to Microwave Technology
The realm of microwave technology embodies a fascinating intersection of physics, engineering, and practical application. This article aims to shed light on the intricacies of microwave transmitters, which serve as pivotal components in various modern systems. Understanding the foundations of this technology is imperative since it influences diverse fields, including telecommunications, scientific research, and industrial processes. With microwave transmitters, information can be transmitted efficiently over long distances, making them indispensable in today's connected world.
Microwave technology utilizes electromagnetic waves with frequencies in the range of 300 MHz to 300 GHz. This particular frequency band enables high data rates while minimizing interference. As we explore the components and functionality of microwave transmitters, we will uncover their significance in enabling reliable communication systems. Overall, this exploration is not just technical detail; it represents key mechanisms that enhance our capacity to share information swiftly and accurately.
What is a Microwave Transmitter?
A microwave transmitter is a device that converts electrical signals into microwave signals. These devices generate and deliver high-frequency electromagnetic waves, which carry information over vast distances. The essential purpose of a microwave transmitter is to send data, be it voice, video, or any other form, across communication networks. Their operation typically involves creating an oscillating signal, amplifying it, and transmitting it through an antenna.
In practical terms, microwave transmitters are found in various applications, from satellite communications to radar systems. They serve distinct functions that are vital for ensuring effective data transmission in differing environments.
Historical Development
The historical evolution of microwave technology is marked by significant breakthroughs and discoveries that have shaped its current form. The journey began in the early 20th century when researchers first explored the properties of electromagnetic waves. Notably, the development of radar during World War II accelerated microwave technologies' progress. As military applications expanded, the understanding and design of microwave transmitters advanced significantly.
In the decades that followed, the commercialization of microwave technologies surged. With the advent of the transistor in the late 1940s, power efficiency improved notably. The introduction of solid-state devices further revolutionized the design and deployment of microwave transmitters. Today, they are integral to the functioning of many essential systems, influencing how we communicate and access information.
In summary, recognizing both the current functionality and historical context of microwave transmitters contributes to a deeper appreciation of their pivotal role in modern technology.
Components of a Microwave Transmitter
Microwave transmitters are complex systems with several critical components working in concert. Understanding these components is paramount for appreciating how microwave transmitters function and their applications in various fields. Each part plays a distinctive role, influencing the efficiency and effectiveness of the entire system. Here, we explore the key elements that contribute to the functionality of a microwave transmitter, while also considering their benefits and practical aspects.
Microwave Oscillator
The microwave oscillator is a fundamental element of any microwave transmitter. It generates the initial signal that will be amplified and transmitted. Typically, oscillators are designed to operate at specific frequencies, essential for meeting communication standards and requirements. The most common types of oscillators used in microwave applications include Gunn diodes, YIG oscillators, and local oscillators.
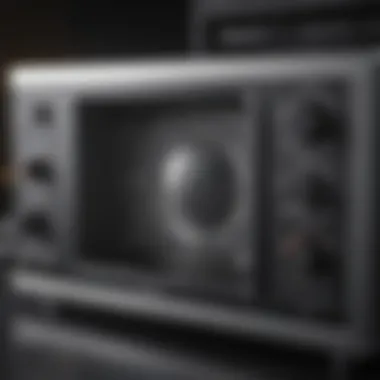
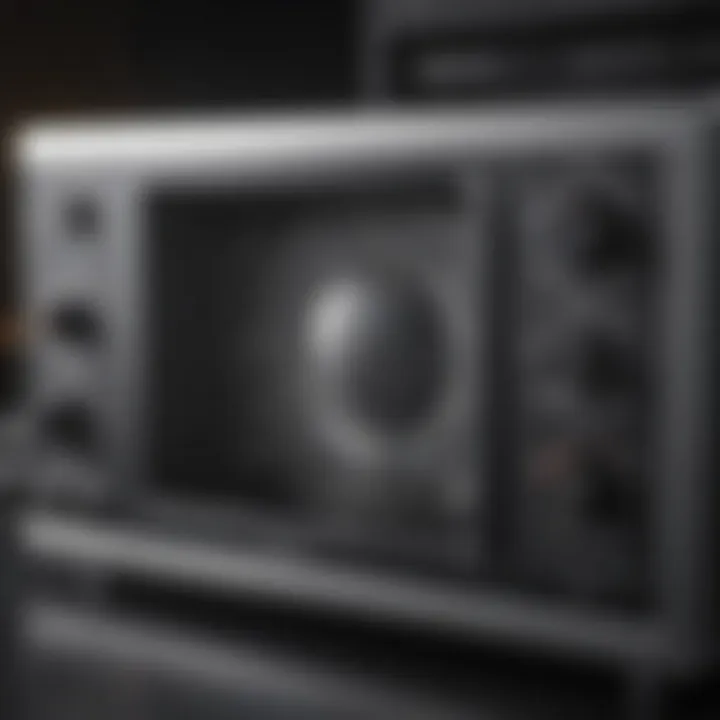
The precision of the microwave oscillator directly affects the performance of the transmitter. A stable oscillator ensures minimal drift in frequency, which is crucial for maintaining signal integrity over distance. Local oscillators help in the frequency conversion process, thereby extending the range of communication while improving overall efficiency.
Amplifier Modules
Amplifier modules are also vital in microwave transmitters. These components take the weak signal generated by the oscillator and boost it to the needed power level for transmission. The choice of amplifier is critical, as it impacts the overall performance and quality of the signal sent out. Common amplifier types include solid-state amplifiers and traveling wave tube amplifiers.
Solid-state amplifiers are generally favored for their compact size and high efficiency. They provide excellent linearity and thermal stability, making them suitable for many applications. On the other hand, traveling wave tube amplifiers excel at high power output, which is essential for applications such as satellite transmission.
Choosing the right amplifier module depends on the specific application requirements, including power, bandwidth, and operational environment.
Antenna Systems
Antenna systems are the final piece in the microwave transmission chain. They convert the electrical signals from the amplifier into electromagnetic waves, transmitting them through space. Antennas come in diverse designs, such as parabolic dishes, horn antennas, and phased array systems. Each design serves different purposes and has unique advantages.
For example, parabolic antennas are ideal for long-distance communication due to their high gain and narrow beamwidth. In contrast, phased array antennas allow for rapid beam steering and are beneficial in radar applications. Understanding antenna characteristics is crucial for optimizing transmission range and quality.
Power Supply Considerations
Finally, power supply considerations are crucial for the reliable operation of microwave transmitters. The power supply must provide adequate voltage and current to each component, particularly during peak transmission times. A well-designed power supply system ensures system stability and improves the longevity of components.
Factors such as power efficiency and backup systems should be considered. Uninterrupted power supply systems are often implemented in critical applications to prevent signal loss or degradation. This aspect is essential for applications in telecommunications and scientific research.
Principles of Operation
Understanding the principles of operation of microwave transmitters is crucial as they form the foundation of how these systems communicate and interact within various applications. The effectiveness of microwave technology largely hinges on the generation of microwave signals, the modulation techniques employed, and the subsequent transmission and reception processes. Each of these components not only works in tandem but also addresses specific challenges and requirements inherent in modern communication systems.
Generation of Microwave Signals
Microwave signals are the lifeblood of microwave transmitters. These signals are typically generated using devices known as oscillators. An oscillator produces regular waveforms, which are essential for establishing the high frequency needed for microwave communication. Common types of oscillators used include stabilized oscillators and local oscillators, each serving different functions. The formation of these signals involves complex electromagnetic principles, necessitating precise control over frequency and phase.
- Importance: Generating consistent microwave signals ensures high-quality transmission, reducing the risk of interference and signal loss.
- Frequency Range: Typically, microwaves operate within the frequency range of 300 MHz to 300 GHz. Higher frequencies often result in better transmission capabilities but also require more sophisticated technology to manage.
Accurate generation of these signals ultimately dictates the reliability and clarity of communication systems.
Signal Modulation Techniques
Modulation refers to the process of altering a carrier signal, allowing it to carry information. There are various modulation techniques used in microwave transmitters, including amplitude modulation (AM), frequency modulation (FM), and phase modulation (PM). Each method has its advantages and is selected based on the specific requirements of the communication system.
- Amplitude Modulation: In this technique, the amplitude of the carrier wave is varied according to the information signal. It is generally simpler but more susceptible to noise.
- Frequency Modulation: Here, the frequency of the carrier wave is varied, making it more robust against interference, often used in applications requiring higher fidelity.
- Phase Modulation: This involves altering the phase of the carrier wave and is essential in advanced digital communications.
These modulation methods enable accurate data transmission over long distances, maintaining the integrity of the information conveyed.
Transmission and Reception Process
The transmission process begins once the microwave signals have been generated and modulated. The modulated signals are sent through antenna systems, converting electronic signals into electromagnetic waves that can travel through the air.
Upon reaching the target receiver, the process must be reversed. The receiver's antenna captures the electromagnetic waves and converts them back into electrical signals. This back-and-forth exchange is critical for enabling clear communication.
- Important Aspects: The polarization of antennas, signal strength, and surrounding environment greatly influence transmission efficiency.
- Challenges: Factors like atmospheric conditions, physical obstructions, and interference from other signals can degrade the quality of reception, emphasizing the need for careful design and materials.
To maximize the effectiveness of microwave transmitters, understanding these principles provides invaluable insights into their operation and enhances applications across varied fields, from telecommunications to scientific research.
Understanding how signals are generated, modulated, and transmitted is essential for grasping the functionality of microwave transmitters.
Types of Microwave Transmitters
Understanding the types of microwave transmitters is crucial for comprehending the nuances of microwave technology. Each type serves distinct purposes and brings certain advantages to the table. Their functionalities are tailored for specific applications, which highlights why they are considered significant across various domains such as communications, scientific research, and industry. Essentially, knowing the types aids in the optimal selection for particular requirements.
Continuous Wave Transmitters
Continuous wave (CW) transmitters produce a constant signal without interruptions. Their ability to emit a steady and unmodulated frequency makes them suitable for various applications. One key feature of CW transmitters is their simplicity in design and operation. They can easily generate signals that remain stable over time.
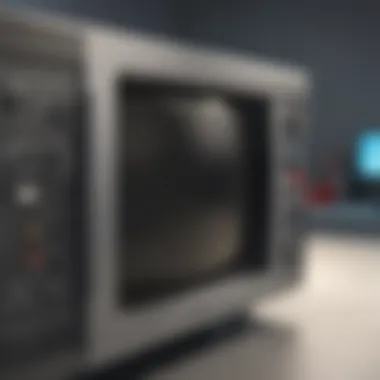
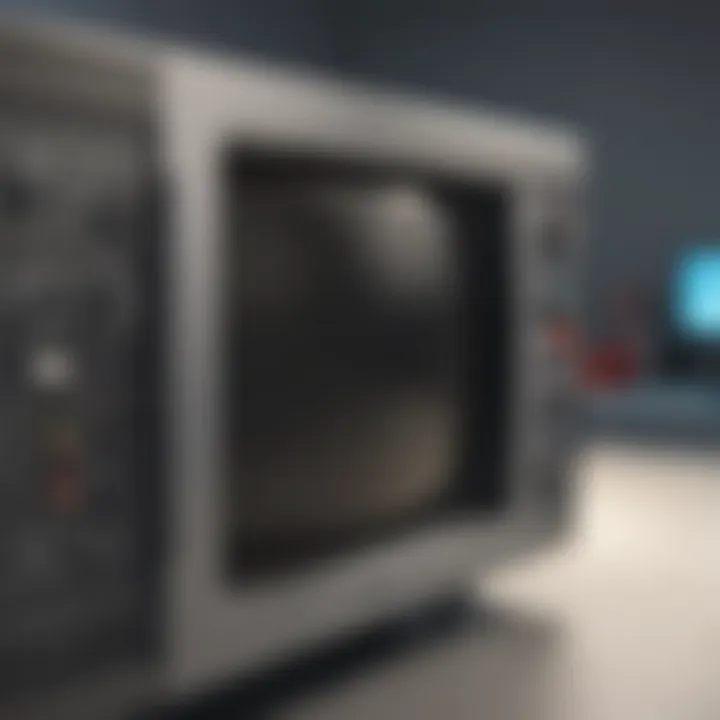
CW transmitters are often used in radar systems and other sequential measurement technologies. In radar, they allow for the effective measurement of distance and speed through frequency shifts. This functionality is possible due to the Doppler effect, where changes in frequency occur based on the relative motion of objects.
The continuous nature of this signal enables enhanced detection capabilities in radar applications.
In communications, they are sometimes favored for short-range transmissions where signal integrity is paramount. The challenge with continuous wave transmitters includes susceptibility to noise and interference that can distort the signal, leading to potential issues in long-range applications.
Pulsed Transmitters
Pulsed transmitters, as the name implies, emit microwave signals in short bursts or pulses. This method allows for effective use of power and provides advantages in various applications. The intermittent nature of the output facilitates easier handling of signal processing requirements.
Pulsed transmitters are widely used in radar and communication systems where precise distance measurement is needed. They can significantly enhance target detection capabilities through time-of-flight calculations. Thanks to pulse compression, these transmitters also maximize resolution in imaging systems, making them beneficial for remote sensing applications.
However, the increased complexity in generating and processing pulses can lead to challenges. Advanced circuitry is often needed for efficient operations, and this may raise overall system costs.
Solid-State vs. Vacuum Tube
The debate between solid-state and vacuum tube transmitters is perennial in the microwave technology field. Each technology possesses distinct characteristics that cater to specific requirements.
Solid-state transmitters are known for their compact size, energy efficiency, and durability. They can operate at room temperature and are less susceptible to physical shock. These devices typically utilize semiconductor technologies and are preferred for applications like satellite communications where reliability and performance over long periods is critical.
In contrast, vacuum tube transmitters, which include magnetrons and klystrons, are often chosen for their high power output capabilities. They can handle high-frequency operations, making them well-suited for many radar applications. However, they are larger, require more power, and can be less reliable in terms of lifespan.
Understanding the different types of microwave transmitters helps in addressing specific industry requirements with suitable technology.
Applications of Microwave Transmitters
Microwave transmitters play a crucial role in various fields. Their unique ability to handle high-frequency signals enables a wide array of applications. From communication systems to scientific research, the benefits are extensive. Moreover, these applications not only streamline processes but also enhance the efficiency and reliability of systems across different industries.
Communication Systems
Telecommunications
In telecommunications, microwave transmitters enable high-speed data transfer. They thrive on their ability to transmit signals over long distances with minimal interference. This characteristic makes them indispensable in modern communication networks.
The unique aspect of telecommunications lies in its versatility. Microwave transmitters can adapt to various frequencies and modulations, ensuring reliable communication. This adaptability is a significant reason why they are preferred in this field. However, one should consider the limited bandwidth in some microwave systems, which could affect the overall performance in densely populated areas.
Satellite Communications
Satellite communications utilize microwave transmitters to relay information across vast distances. The specific aspect of this application is its capability to provide coverage even in remote locations. Such functionality is vital for global connectivity.
The key characteristic of satellite communications is its resilience against geographical barriers. This feature makes it a popular choice for regions where terrestrial communication is challenging. One unique advantage is the minimal delay in signal transmission, although it can be hampered by atmospheric conditions, which may affect reliability.
Scientific Research
Remote Sensing
Remote sensing relies on microwave transmitters to gather data about the Earth's surface. This application is fundamental in environmental monitoring and resource management. The importance of remote sensing lies in its ability to collect information over large areas without physical presence.
A key characteristic of this technology is its capacity to penetrate clouds and darkness, providing crucial data for research. It is favored for its accuracy and noninvasive nature. However, the analysis of data from remote sensing can be complex and often demands advanced computational techniques.
Material Analysis
Material analysis benefits from the precision of microwave transmitters. They are used to investigate the properties of various substrates in diverse fields, such as manufacturing and construction. The crucial role of this application is observed in quality control and testing processes.
Material analysis stands out due to its ability to provide real-time data. This advantage is critical for industries where product integrity is paramount. However, a challenge involves the costly equipment required for such detailed analysis, which may limit accessibility for smaller organizations.
Industrial Applications
Manufacturing Processes
In manufacturing processes, microwave transmitters are applied for various tasks, including monitoring and controlling equipment. The specific aspect of using microwave technology here lies in improving efficiency and safety in production.
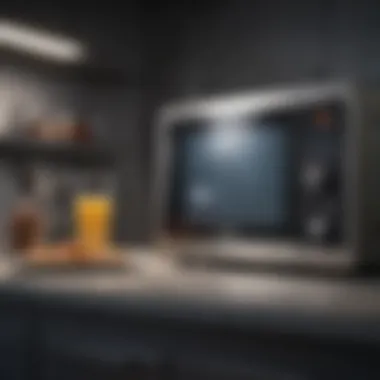
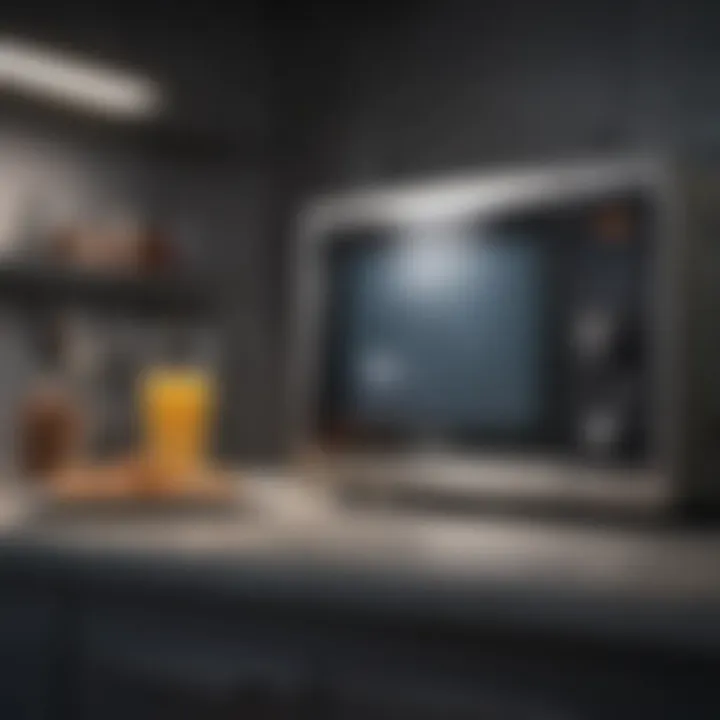
One key characteristic of this application is its capability for non-contact sensing. This feature allows for immediate adjustments during production, which can reduce waste and increase product quality. However, implementing these systems can require a steep learning curve.
Food Processing
In the food processing industry, microwave transmitters enable efficient cooking and drying processes. This technology is becoming increasingly popular as it significantly reduces cooking times while preserving nutrients.
The unique feature of microwave food processing is its even heating capabilities, which prevent hot and cold spots. This leads to better quality control in food production. Yet, the equipment can be expensive, and some processes may affect food texture if not closely managed.
Challenges and Limitations
Understanding the challenges and limitations in microwave transmitter technology is crucial. These factors can impact the effectiveness of transmitters in various applications. From technical issues to environmental impacts and regulatory constraints, each aspect plays a significant role in shaping the future of microwave technology.
Technical Challenges
Technical challenges involve a range of issues. One primary concern is the development of efficient amplifiers. Amplifier modules must provide sufficient gain while minimizing distortions that can interfere with signal quality. Furthermore, the design of antennas needs special attention. Antenna systems must be capable of directing signals effectively, ensuring good transmission range without significant loss.
Another technical hurdle is the frequency stability of the microwave signals. Maintaining specific frequencies during operation is essential for accurate data transmission. Therefore, engineers invest in oscillators that are reliable and stable, but achieving that is a continuous battle. Lastly, integration of new materials into the design may introduce unexpected complications, which further complicates the production of reliable microwave transmitters.
Environmental Impact
The environmental impact of microwave transmitters is an important consideration. These devices contribute to electromagnetic pollution, which can affect both wildlife and human health. Examining the long-term effects of exposure to microwave radiation remains a complex challenge for scientists. Additionally, the manufacturing process of transmitters poses its own environmental issues. The materials used often require significant energy and resources, contributing to waste and carbon emissions.
Mitigating the environmental impact demands innovative solutions. This might include using sustainable materials or improving energy efficiency. Industry stakeholders must find a balance between performance and environmental responsibility.
Regulatory Issues
Regulatory issues surround microwave transmitters as governments impose guidelines for their use. Compliance with regulations ensures that devices operate safely and do not interfere with other communication systems. Different regions might have varying standards, making it a challenge for manufacturers to align products with international norms.
Moreover, the approval process for new equipment can be lengthy. Companies often face delays when trying to bring innovations to market. This regulatory landscape requires careful navigation, prompting manufacturers to stay informed about changes in laws and standards.
"Navigating the regulatory framework is as crucial as the technical performance of the equipment itself"
Future Trends in Microwave Technology
The landscape of microwave technology is constantly evolving. Future trends hold significant importance for various sectors, from communication to science and industry. Understanding these trends is critical for professionals who rely on microwave transmitters. This knowledge allows for the optimization of existing systems and the development of new applications. As new materials and technologies emerge, they will transform how microwave systems are designed and utilized.
Advancements in Materials
Recent advancements in materials science are pivotal to microwave technology. New materials with enhanced properties can significantly improve performance. For instance, advancements in dielectric materials lead to better signal propagation and reduce loss. The introduction of materials like low-loss ceramics and advanced polymers is revolutionizing antenna system designs.
Another area of interest is the development of metamaterials. These engineered materials can manipulate electromagnetic waves in novel ways. This allows for more compact and efficient antenna designs. Enhanced materials can also improve thermal management in microwave systems, thus increasing reliability and lifespan.
Additionally, the use of nanotechnology in the development of microwave components shows promise. Nanostructured materials offer improved electromagnetic properties, which can enhance the sensitivity and spectral response of microwave sensors.
In summary, advancements in materials are critical for driving improvements in microwave transmitter functionality and efficiency.
Integration with Emerging Technologies
The integration of microwave technology with emerging technologies is another significant trend. One example is the collaboration with Internet of Things (IoT) devices. Smart devices require reliable wireless communication, and microwave transmitters can play a crucial role. The high-frequency bands used in microwave communications can support the data-intensive requirements of IoT applications.
Moreover, the rise of 5G technology demands new microwave solutions. As telecommunication networks upgrade, microwave transmitters will need to adapt to higher frequencies and wider bandwidths. This shift necessitates the development of advanced modulation techniques and improved transmitter designs.
In the realm of scientific applications, integrating microwave technology with artificial intelligence is gaining traction. AI can optimize signal processing, enabling quicker analysis and more accurate data interpretation in applications such as remote sensing or material analysis.
Collaboration with software technologies is also important. It can streamline control systems, improve user interfaces, and enhance the overall functionality of microwave systems.
Overall, the integration of microwave technology with these emerging trends will create more sophisticated and efficient systems, broadening their applications across different sectors.
Epilogue
Microwave transmitters play a pivotal role in todayβs technological landscape, bridging the communications gap and facilitating advancements across various domains. Their intricate design and functionality enable efficient transmission of data, making them indispensable in modern infrastructure.
The exploration into the structure and operational principles of microwave transmitters reveals their complexity and efficiency. The components such as microwave oscillators, amplifiers, and antenna systems work synchronously to convert electrical signals into microwave signals, ensuring reliable data transfer in diverse applications. Without these devices, the seamless communication we experience today would be significantly hindered.
Key benefits of understanding microwave transmitters include better insight into telecommunications, industrial processes, and scientific research applications. As we delve deeper into the challenges and limitations, it becomes evident that while microwave technology is robust, it faces hurdles such as technical challenges and regulatory considerations that must be addressed as the technology evolves.
Moreover, as outlined in the previous sections, the future is promising with advancements in materials and the integration of emerging technologies, hinting at more efficient and sustainable solutions. This comprehensive overview fosters a more profound appreciation for the technology that underpins our connected world. The journey through the mechanics and relevance of microwave transmitters underscores their critical position in shaping future innovations.
Understanding the nuances of this technology not only equips students and professionals with essential knowledge, but it also emphasizes the significance of continued research and development in this field.