Understanding mRNA's Role and Importance in Cells
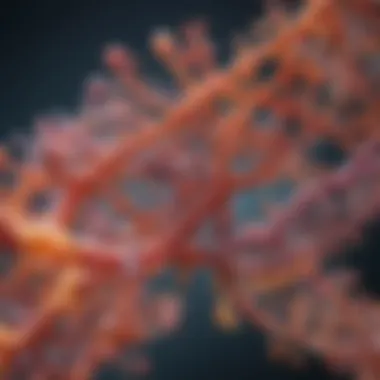
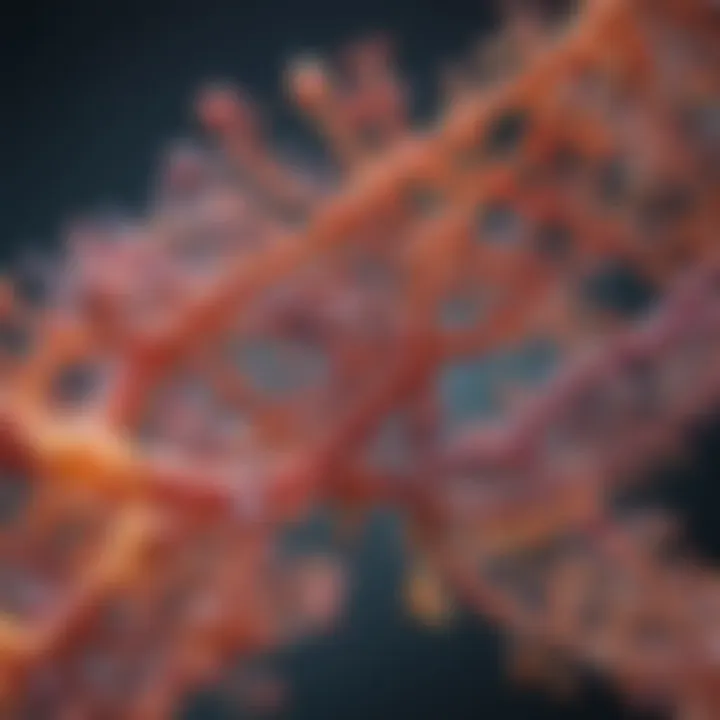
Intro
The significance of messenger RNA (mRNA) has been thrust into the spotlight, particularly with the rapid advancements in biotechnology and medicine. Understanding mRNA's role is essential for grasping how genetic information translates into proteins that drive cellular processes. This exploration reveals the intricacies of mRNA's function within cells, highlighting both its fundamental biological purposes and emerging applications. Through the lens of current research and innovation, we’ll uncover how this molecule not only serves as a bridge between DNA and protein synthesis but also paves the way for groundbreaking therapies in modern medicine.
Research Overview
Summary of Key Findings
Messenger RNA plays a crucial role in the intricate web of cellular functions, bridging the gap between genes and the proteins they encode. Key findings in this field indicate that mRNA is not just a passive messenger. Instead, it actively participates in regulating gene expression and responding to environmental stimuli. The advancements in understanding mRNA dynamics shed light on how cells adapt to varying conditions and engage in processes such as growth, differentiation, and reaction to stress.
"The discovery of mRNA's role extends beyond traditional understandings of protein synthesis; it now encompasses its influence on cellular regulation and therapeutic interventions."
Importance of the Research
The implications of studying mRNA extend far beyond academia. With the recent breakthroughs in mRNA vaccine development, particularly during the COVID-19 pandemic, the practical benefits of understanding this molecule have become glaringly apparent. Such research not only contributes to our grasp of fundamental biological processes but also propels innovations in vaccine technology, cancer treatments, and personalized medicine. As scientists continue to unveil the complexities of mRNA, its significance in enhancing human health becomes more profound, making it a focal point for future biological research and clinical application.
Methodology
Study Design
Research regarding mRNA functions often relies on a mix of experimental and computational approaches. By utilizing techniques such as gene editing (CRISPR/Cas9) and high-throughput sequencing, researchers can analyze mRNA expression profiles in various tissues under different conditions. This blend of methodologies allows for a comprehensive understanding of mRNA's roles in cellular mechanics.
Data Collection Techniques
Data collection in mRNA research typically includes:
- RNA-Sequencing (RNA-seq): Provides detailed insights into the transcriptome, including mRNA abundance and splicing variants.
- Quantitative PCR (qPCR): Measures mRNA levels to assess gene expression changes in response to stimuli.
- Fluorescence In Situ Hybridization (FISH): Visualizes mRNA within cells, linking expression patterns to cellular processes.
These techniques not only enrich the understanding of mRNA’s functional landscape but also lay the groundwork for applying this knowledge in biotechnology and therapeutic strategies.
Preamble to mRNA
Messenger RNA, or mRNA, serves as a fundamental molecule in the cellular machinery, guiding the synthesis of proteins crucial for life's myriad processes. Understanding mRNA is not merely an academic exercise; it’s an exploration into the heart of biology. This section dives into the essence of mRNA, why it holds significance in various cellular mechanisms, and how it shapes our understanding of molecular biology today.
Throughout history, scientists have unveiled how mRNA translates genetic blueprints into the proteins that perform a vast array of functions within cells. From muscle contraction to enzymatic activity, the influence of mRNA echoes through nearly every biochemical pathway. By grasping its role, one can appreciate how disruptions in mRNA functions can lead to diseases, particularly as the links between mRNA and health continue to unfold.
The study of mRNA positions itself not just in the realm of theoretical knowledge but also in practical applications, especially in therapeutic strategies such as mRNA vaccines, which have gained enormous attention. This exploration serves both as a foundation and a launchpad into the complexities of cellular processes, helping students, researchers, educators, and professionals uncover the interdependencies of life at a molecular level.
Defining mRNA
mRNA acts as an intermediary between DNA and proteins. Essentially, it carries the coded information from DNA in the nucleus to ribosomes in the cytoplasm, where proteins are synthesized. To put it simply, think of mRNA as the delivery worker bringing the genetic message from the DNA's "blueprint" to the cellular factories.
In its structure, mRNA is a single-stranded molecule that comprises nucleotide sequences, each made of a sugar, a phosphate group, and a nitrogenous base. The sequence and composition of these bases—adenine, guanine, cytosine, and uracil—dictate the order in which amino acids are assembled into a protein. The uniqueness of mRNA lies in its ability to be translated into distinct proteins, which further underscores its crucial role in defining cellular function.
Historical Context
The journey to comprehend mRNA's function began in earnest in the early 20th century, when researchers started piecing together the genetic code. One of the landmark moments came from the work of Francis Crick and James Watson, who in 1953 unveiled the double-helix structure of DNA. This revelation paved the way for further discoveries, leading to the identification of RNA as a key player in protein synthesis.
By the 1960s, scientists like Marshall Nirenberg were exploring how sequences of nucleotides correspond to specific amino acids, essentially cracking the genetic code. This foundational work laid the groundwork for understanding mRNA's role in biology. As research progressed, it became evident that mRNA is not just a passive messenger but an active participant in gene expression regulation, revealing layers of complexity in cellular processes.
In the decades since, our knowledge has expanded exponentially. With advancements in genetic technology and the advent of tools like CRISPR, the exploration of mRNA and its many roles continues to evolve. Today, understanding mRNA has implications far beyond academia; it affects medicine, biotechnology, and our fundamental understanding of life itself.
"mRNA is not a mere messenger; it is a dynamic player integral to the orchestration of cellular symphony."
As we delve deeper into the subsequent sections, the transformative impact of mRNA will unfold further, touching upon its key functions in protein synthesis, the intricacies of gene expression, and its remarkable applications in the realm of biotechnology.
The Role of mRNA in Protein Synthesis
The role of messenger RNA (mRNA) in protein synthesis is critical to understanding cellular processes and the intricate mechanisms of life. Essentially, mRNA serves as a blueprint for the synthesis of proteins, which are pivotal for the structure and function of cells. Without mRNA, the information encoded in DNA would remain dormant, unable to translate into the proteins necessary for cellular functions and organismal survival. In this section, we will explore the transcription process, translation mechanism, and the specific roles of each component involved in protein synthesis.
Transcription Process
Transcription is the first step in the flow of genetic information from DNA to RNA. Here, DNA serves as a template, and RNA polymerase is the enzyme that synthesizes mRNA by unwinding the double helix and complementary pairing. This process is tightly regulated and ensures the right gene expression at the right time.
Initiation of Transcription
The initiation of transcription is where the magic begins. This phase involves the binding of RNA polymerase to a specific region of DNA known as the promoter. The promoter's unique sequence characteristics are crucial because they dictate when and where transcription starts.
A notable aspect of this initiation is the presence of various transcription factors that facilitate RNA polymerase attachment. They function almost like traffic signals, controlling the flow of transcription. The ability of these factors to determine the timing is a significant benefit, enabling precise regulation of gene expression, which can lead to advantages in adapting to environmental changes.
While initiation is essential, it isn’t without challenges; misregulation can lead to inappropriate gene expression, potentially resulting in diseases such as cancer. This highlights the balance needed during the initiation phase.
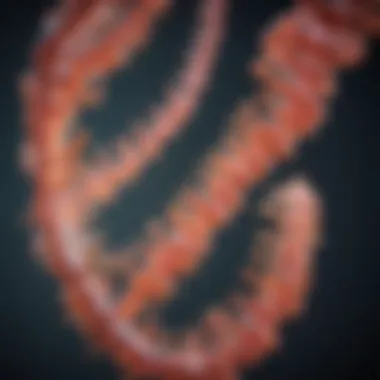
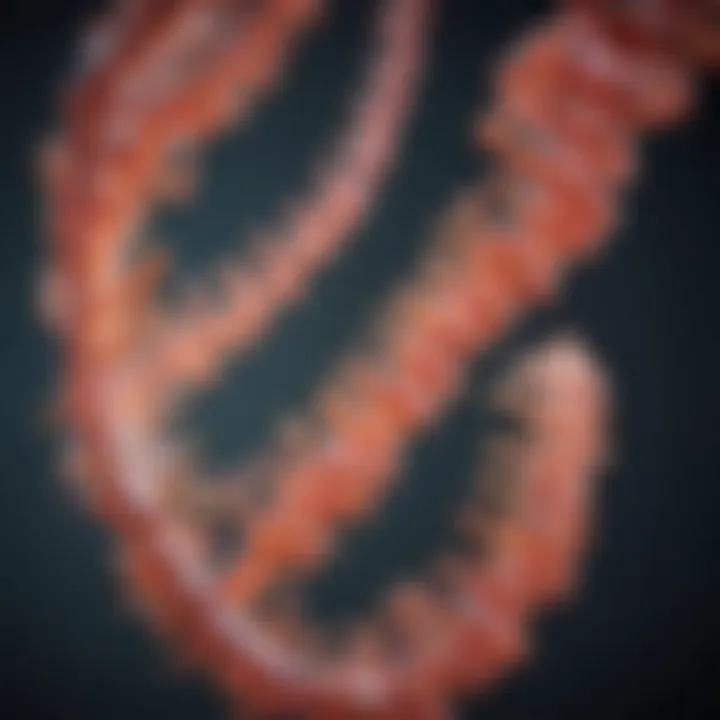
Elongation and Termination
Once transcription is initiated, we move into the elongation phase, where RNA polymerase synthesizes the mRNA strand by reading the DNA template. It’s like a conveyor belt, continuously adding nucleotides, making mRNA grow longer and longer.
Termination is equally crucial as it signals the end of transcription. When RNA polymerase encounters a specific sequence known as a terminator, it facilitates the release of the newly synthesized mRNA strand. The beauty of this process lies in its efficiency. It ensures that only the necessary portions of DNA are transcribed, avoiding any unnecessary or erroneous sequence elongation.
A unique feature of termination is how it affects mRNA stability. The way the mRNA is capped and the poly-A tail adds a layer of protection against degradation, a popular consideration for ensuring mRNA remains intact for translation. However, if this process fails, it can lead to mRNA molecules that are short-lived, affecting protein synthesis.
Translation Mechanism
After mRNA synthesis, the next step is translation, which takes place in the ribosomes—the cellular factories for protein synthesis. The translation mechanism converts the sequence of mRNA into a specific amino acid chain, ultimately forming proteins essential for various cellular functions.
Ribosome Structure
Ribosomes are fascinating structures made of ribosomal RNA and proteins, forming two subunits that come together during translation. Their composition and arrangement are pivotal. They provide a platform for the mRNA to bind, ensuring that the genetic code can be read correctly.
A unique aspect of ribosome structure is its ability to facilitate the binding of tRNA, connecting the mRNA codons with corresponding amino acids. This feature underscores the ribosome’s role as a mediator between mRNA and protein synthesis. The ribosome’s efficiency is a significant benefit, allowing for the rapid production of proteins, a must in a dynamic cellular environment.
However, their complex nature can also lead to misreading of the mRNA code, which may result in incorrect protein synthesis. This is a risk that can have severe biological consequences, emphasizing the importance of ribosomal fidelity.
tRNA Functions
Transfer RNA (tRNA) plays a crucial role in translation as the adapter molecule that connects mRNA to amino acids. Each tRNA molecule carries a specific amino acid and recognizes the corresponding codon on the mRNA through its anticodon. This function is essential for ensuring the correct sequence of amino acids is added to the growing protein chain.
The beauty of tRNA lies in its versatility; there are many tRNA molecules for the various amino acids, which allows a robust and flexible system for protein synthesis. The unique feature of tRNA to bring the right amino acids directly to the ribosome aids in efficiency. A key characteristic of tRNA function is its ability to ensure a tight coupling between mRNA sequence and protein structure, ultimately determining protein function.
However, tRNA also faces its own challenges. Misalignment can lead to the incorporation of incorrect amino acids, which may affect the stability and functionality of the resulting protein. Thus, maintaining tRNA integrity is crucial for the overall accuracy of protein synthesis.
mRNA is not just a catalyst for protein synthesis; it is a fundamental player in determining cellular fate and function.
Types of mRNA
Understanding the different types of mRNA is essential in grasping the broader implications of molecular biology, especially in the context of cellular processes. Each type offers unique roles and insights into gene regulation and expression. The primary categories include coding mRNA and non-coding mRNA. Their significance reflects not just on their functions, but also on how they interact with various cellular mechanisms, shaping the landscape of molecular biology.
Coding mRNA
Coding mRNA, often represented as mRNA, serves a pivotal function in the synthesis of proteins. This class of mRNA consists of sequences that are translated into amino acids, which ultimately form proteins — the building blocks of life. The coding sequence from mRNA contains the information that determines the structure and function of proteins, making it highly significant in biological processes.
For instance, consider the mRNA translated from the gene that encodes insulin. The precise sequence of nucleotides in the coding mRNA dictates how insulin is formed and, consequently, how glucose is regulated in the body. This exemplifies the direct impact that coding mRNA has on health, metabolism, and, in broader terms, homeostasis.
Furthermore, coding mRNA is crucial during the process of gene expression. It undergoes transcription from DNA to mRNA, followed by translation to protein. The fidelity of this process is vital, as errors can lead to diseases like cancer. Therefore, a profound understanding of coding mRNA not only accentuates its intrinsic biological importance but also its applications in medical research and therapeutic interventions.
Non-Coding mRNA
Non-coding mRNA, contrary to its name, plays a vital role in cellular function, even though it does not code for proteins. Instead, this type of mRNA contributes to various regulatory processes, primarily through its involvement in gene expression and RNA modulation.
Functions and Significance
The diverse roles of non-coding mRNA, including microRNA (miRNA) and long non-coding RNA (lncRNA), highlight its importance in the regulation of gene expression. Non-coding mRNAs can influence processes such as chromatin remodeling, RNA processing, and the maintenance of cellular identity.
- Key Characteristic: A standout feature of non-coding mRNAs is their ability to regulate gene expression post-transcriptionally, which is paramount in fine-tuning cellular responses to environmental stimuli.
- Unique Feature: Their interactions tend to be complex, involving multiple pathways that can inhibit or promote the availability of coding mRNA. For example, miRNAs can bind to coding mRNAs, leading to degradation or translation repression. This reflects a sophisticated level of control distinct from that of coding mRNA.
- Advantages/Disadvantages: While these regulatory mechanisms are beneficial for preventing diseases such as cancer or genetic disorders through precise control, they also pose challenges in terms of therapeutic interventions. Targeting non-coding mRNAs can be tricky, given their intricate networks of interactions within the cell.
In summary, both coding and non-coding mRNAs are integral to cellular processes, with distinct yet complementary roles in gene expression and regulation. Understanding these types lays the groundwork for further exploration into how mRNA-based therapies could revolutionize treatment strategies in modern medicine.
Gene Expression Regulation
The regulation of gene expression is a pivotal aspect driving countless cellular processes. By fine-tuning which genes are switched on or off, cells can adapt to various stimuli and demands of their environment. This regulation helps ensure that the right proteins are synthesized in the right amounts at the right times—a necessity for maintaining homeostasis and responding to challenges like environmental changes or developmental cues.
In the context of mRNA, gene expression regulation plays a crucial role. This regulation not only influences the initial transcription of mRNA but also governs its stability, processing, and translation. Through specific mechanisms, cells leverage these regulatory elements to manage diverse functions, from responding to stress to facilitating normal growth and development.
Transcriptional Control
Transcriptional control is fundamental to gene expression regulation. This process determines the ability of RNA polymerase to bind to the DNA and synthesize mRNA, affecting everything that follows in the gene expression chain.
Promoters and Enhancers
Promoters and enhancers are essential components of the transcriptional regulatory landscape. Promoters are sequences located just upstream of the genes they regulate and serve as the docking station for RNA polymerase. On the other hand, enhancers can be situated far away from the genes and interact with promoters in a three-dimensional space to boost transcription rates.
- Key Characteristics: One significant characteristic of both promoters and enhancers is their ability to control transcription initiation effectively. This is the primary reason they are crucial in this article. Without these regulatory elements, gene expression could be disorganized, leading to potential issues in cellular function.
- Unique Features: Promoters possess core elements that are universally recognized by RNA polymerase, ensuring that transcription starts correctly. Enhancers, however, can interact with multiple promoters and can sometimes even influence distantly located genes, giving them a unique advantage in multifaceted gene regulation.
But there are disadvantages too. Enhancers might become overactive, leading to excessive gene expression, which is linked to conditions like cancer. The delicate balance they maintain is vital for cellular health.
Repressors and Activators
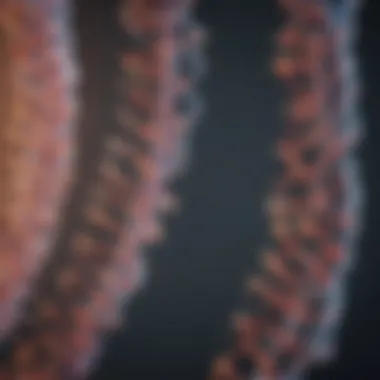
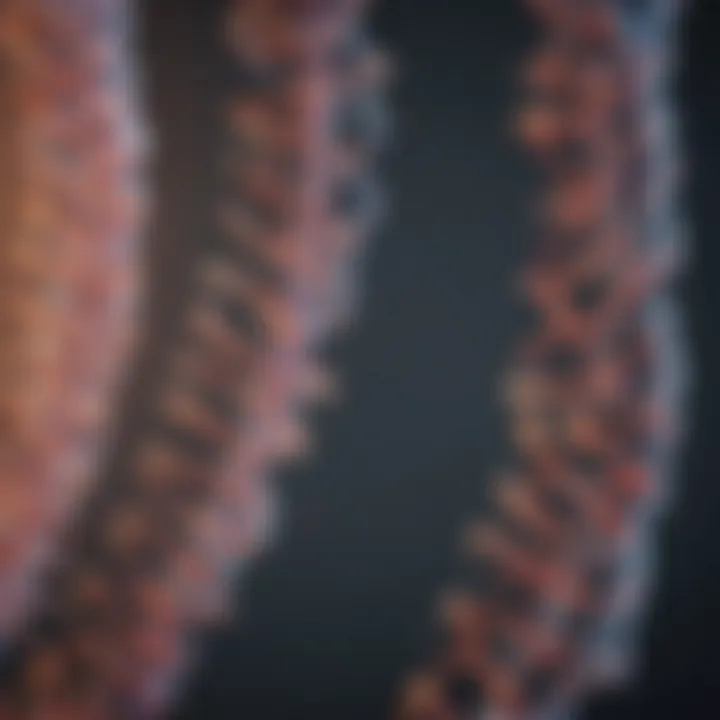
Repressors and activators contribute to the nuanced control of gene expression. Activators are proteins that bind to enhancers, facilitating the recruitment of RNA polymerase, thus promoting transcription. Conversely, repressors are proteins that bind either to promoters or enhancers and obstruct the transcription machinery.
- Key Characteristics: The primary characteristic of activators is their ability to enhance transcription efficiency, which is pivotal for gene expression, making them a beneficial aspect discussed here. Repressors play an equally important role by putting the brakes on transcription, allowing selective gene expression.
- Unique Features: Apart from lowering or increasing transcription rates, repressors can prevent the formation of transcription complexes, while activators can attract necessary cofactors. This interaction can create regulatory networks that help in fine-tuning the gene expression in response to cellular signals.
However, an excessive amount of repression from these proteins can result in inadequate expression of essential genes, impacting growth or disease resistance.
Post-Transcriptional Modifications
Post-transcriptional modifications further influence gene expression after transcript has been synthesized. These modifications can alter the stability, localization, and translation of mRNA, critically impacting protein synthesis.
Splicing
Splicing is the process by which non-coding regions (introns) are removed from the pre-mRNA, while coding regions (exons) are joined together. This process is vital for generating mature mRNA that can be translated into proteins.
- Key Characteristics: A key characteristic of splicing is its specificity; incorrect splicing can lead to non-functional or even harmful proteins. This property makes splicing a focus of interest in this article since it ensures that only the right sequences give rise to functional mRNA.
- Unique Features: Intriguingly, splicing can generate multiple mRNA variants from a single gene through alternative splicing. This unique feature allows for greater diversity in protein production, expanding the functional repertoire without increasing the number of genes.
While alternative splicing offers remarkable versatility, it can also lead to complexities that may result in misregulation, contributing to diseases such as cancer.
Polyadenylation
Polyadenylation involves adding a poly(A) tail to the 3' end of mRNA, which is crucial for mRNA stability and transport out of the nucleus.
- Key Characteristics: It increases the lifespan of mRNA by protecting it from degradation, ensuring that the mRNA is suitably available for translation. This aspect is beneficial, allowing for precise control over protein synthesis and making it a key element in this article.
- Unique Features: Poly(A) tails also facilitate the translation process by helping ribosomes recognize the mRNA. This unique feature creates an interface between mRNA stability and translational efficiency.
However, if polyadenylation occurs incorrectly, it can lead to the destabilization of mRNA, causing inadequate protein synthesis, which can have dire consequences for the cell's health.
Understanding these complex interactions and modifications is fundamental to grasping how mRNA functions in cellular processes, highlighting the intricate balance of expression that keeps cellular machinery running smoothly.
Role of mRNA in Disease Mechanisms
The significance of mRNA in disease mechanisms cannot be overstated. Within the complex web of cellular functions, messenger RNA serves as a pivotal player. Its role extends beyond mere transmission of genetic information; it strongly influences how cells respond to internal and external stimuli. This section will delve into two vital areas where mRNA displays its influence: cancer and viral infections.
mRNA and Cancer
Cancer represents a major health threat globally, and mRNA plays a multifaceted role in this ailment. One of the noteworthy aspects is that mRNA is often abnormally expressed in malignant cells compared to normal cells. This aberrant expression can contribute to oncogenesis—the process of tumor formation. For example, certain oncogenes—genes that, when mutated or overexpressed, promote the growth of cancer—are translated from mRNAs that are expressed at elevated levels.
In precision medicine, targeting specific mRNA molecules has become a viable strategy. Antisense oligonucleotides and RNA interference are just two examples of approaches that aim to inhibit the translation of harmful mRNAs, potentially leading to tumor reduction.
- Key Aspects of mRNA in Cancer:
- Altered expression patterns in oncogenes and tumor suppressor genes
- Utilization in targeted therapies
- Potential as biomarkers for diagnosis and prognosis
Insights and Considerations
Understanding the interaction between mRNA and cancer pathways can pave the way for innovative treatment options. Not only does it aid diagnostics, but it also fosters the development of new therapeutics. This newfound insight reveals mRNA's dual nature, acting both as a culprit in disease and a target for intervention.
mRNA in Viral Infections
Viral infections exploit the host's cellular machinery to replicate, and central to this process is the manipulation of mRNA. Viruses utilize their own mRNA to hijack a host cell’s resources, forcing it to produce viral components instead of normal cellular products. The infamous SARS-CoV-2 virus, for instance, has its own mRNA that instructs host cells to synthesize chains of viral proteins, aiding in viral assembly and replication.
Moreover, the host immune response is also linked to mRNA. In an attempt to resist infection, host cells generate specific mRNAs that encode interferons, proteins crucial in the immune response to viruses. This cat-and-mouse game highlights how mRNA is a battleground where host defenses and viral strategies collide.
- Key Points in Viral Infections:
- Viral mRNA manipulation of host cell machinery
- Host responses mediated by mRNA production
- Emerging mRNA vaccine technology targeting viral diseases
An understanding of how viruses manipulate mRNA paints a clearer picture of infection dynamics. This knowledge is crucial for developing vaccines and antiviral drugs.
In summary, the interplay between mRNA and disease mechanisms, especially in cancer and viral infections, shows a world of opportunities for researchers and clinicians. Recognizing the nuances of how mRNA functions lays the groundwork for enhanced treatment modalities and a deeper grasp of disease pathology.
Biotechnological Applications of mRNA
The implications of mRNA technology have surged in recent years, marking a transformative epoch in biotechnology and medicine. It has given rise to innovative approaches that enhance healthcare solutions. This section unwraps how mRNA fits into biotechnological advancements, shedding light on its applications and overshadowing its potential.
mRNA Vaccines
Mechanism of Action
The core of mRNA vaccines lies in their ability to instruct cells to produce specific proteins that mimic those present in pathogens. By introducing a synthesized strand of mRNA into the body, it allows cells to craft these proteins, which in turn triggers an immune response. The notable aspect of this method is its speed; once the mRNA is inside, the body can start creating the protective proteins almost immediately. This feature makes mRNA vaccines astonishingly efficient and is one reason they gained traction so quickly during the pandemic.
However, while the perks are apparent, there are some drawbacks worth noting. The stability of mRNA can be somewhat unpredictable, requiring careful handling and sophisticated storage conditions. It does raise questions about scalability and accessibility, especially in regions with limited resources. Nevertheless, the potential of mRNA in vaccine technology cannot be overstated as it opens doors to combating various infectious diseases, potentially saving countless lives globally.
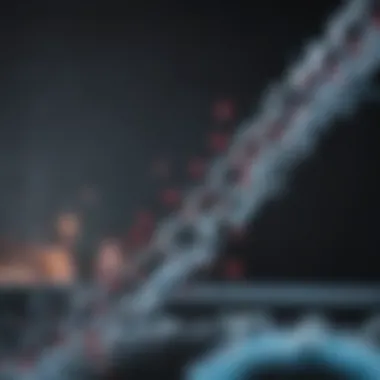
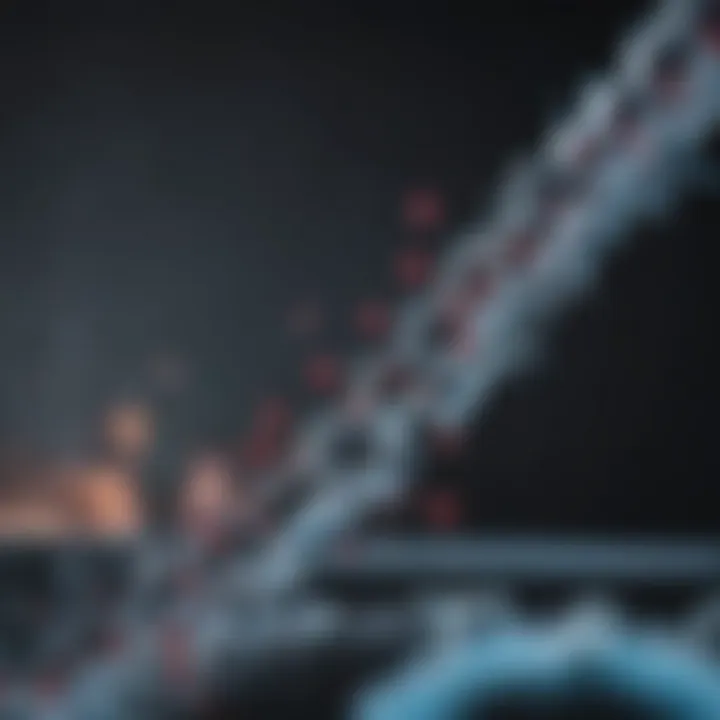
Clinical Impact
When considering the clinical impact of mRNA vaccines, efficacy becomes the talk of the town. Trials and studies have indicated their remarkable ability to generate immunity against specific viruses like SARS-CoV-2, offering a robust defense mechanism. This platform has empowered researchers to adapt quickly to emerging diseases, demonstrating mRNA's agility as a significant player in modern medicine. The swift adaptation in response to variants is one of its standout features.
Moreover, these vaccines have set a precedent for future innovations in vaccine development. They pave the way for mRNA technology to be tailored for other diseases, including cancers and auto-immune disorders. However, one must consider the rare adverse reactions some individuals experience, adding layers to the conversation on safety that the medical community must continue to address.
Therapeutic Developments
Gene Therapy Approaches
In gene therapy, mRNA technology ushers in a novel way to correct or compensate for defective genes. The aim is to deliver healthy gene copies to tissues at the cellular level, which can lead to significant advancements in treating genetic disorders. The defining trait of this approach is its targeted nature. By honing in on specific genetic anomalies, it stands apart from traditional methods that may involve broader treatments featuring more general interventions.
However, challenges remain. The delivery system for mRNA needs to be exceptionally efficient for this therapy to succeed. Factors such as immune responses and cell uptake play a critical role in the effectiveness. Yet, the potential for treating previously incurable genetic conditions stands as a testament to the revolutionary nature of mRNA.
Protein Replacement Therapy
On the front of protein replacement therapy, mRNA serves the purpose of delivering instructions essential for producing therapeutic proteins that may be missing or malfunctioning in certain diseases. This area showcases mRNA's flexibility; as it's designed to produce a variety of proteins tailored for individual conditions. A standout feature of this treatment is its ability to resolve protein deficiencies, potentially offering quality of life improvements to numerous patients.
Nonetheless, the practicality of this approach hinges on ensuring that the mRNA reaches the right tissues and that the proteins behave as expected in the body. There’s inherent uncertainty, but the possibilities shine bright, warranting further exploration into optimizing this therapy for real-world applications.
"The remarkable potential of mRNA technology reshapes our understanding of health and disease, fostering unprecedented opportunities in treatment strategies."
Through diligent research and thoughtful innovation, the future of biotechnological applications of mRNA appears bright. It could catalyze a paradigm shift in how we approach medicine and genetic engineering, showcasing the dynamism and adaptability of this essential molecule.
Future Perspectives on mRNA Research
The realm of mRNA research is blossoming, holding the promise of revolutionizing not just how we understand biological processes, but also how we treat diseases. With the advent of technologies like mRNA vaccines, there's a palpable excitement in the scientific community. This section delves into the future trajectory of mRNA studies, considering both innovations on the horizon and the challenges that lurk behind them.
Innovations on the Horizon
Emerging technologies are set to further enhance our comprehension of mRNA functionality. A few noteworthy advancements include:
- Next-Generation Sequencing: This technology enables more precise mapping of mRNA molecules, allowing researchers to detect low-abundance transcripts essential for cellular processes. It acts as a magnifying glass that lets us peek deeper into the complexities of gene expression.
- CRISPR Evolution: CRISPR has taken the field by storm, especially when paired with mRNA technologies. This pairing makes gene editing more efficient, putting the power of precise modification into the hands of scientists and potentially leading to cures for genetic diseases.
- Novel Delivery Systems: Innovations in how mRNA is introduced into cells, such as lipid nanoparticles, promise more effective therapeutic approaches, minimizing side effects and maximizing target specificity.
These breakthroughs could pave the way for exciting applications in areas such as personalized medicine, regenerative therapies, and cancer treatment, thereby reshaping our understanding and interaction with biological systems.
Challenges and Considerations
Though the future looks bright, several challenges need to be acknowledged in the burgeoning field of mRNA research. Let's examine two critical aspects: stability issues and ethical implications.
Stability Issues
Stability of mRNA molecules in biological systems is undeniably a pivotal aspect. mRNA is inherently unstable and can be degraded quickly by cellular enzymes, posing challenges for therapeutic applications. The key characteristic of this instability is its relatively short half-life, which limits the duration of its action.
To tackle this, researchers explore different modifications such as:
- 5’ Caps and Poly(A) Tails: These modifications protect the mRNA from degradation, providing it a fighting chance against nucleases.
- Synthesized Nucleotides: Using modified nucleotides in mRNA synthesis helps enhance stability without compromising translation efficiency.
Despite these challenges, the persistent research in stabilizing mRNA equips it with the potential for extended therapeutic use, thus making it a popular choice in biotechnological advances.
Ethical Implications
As we venture further into the landscape of mRNA research, ethical considerations must not be cast aside. Key characteristics of ethical implications surrounding mRNA technology include:
- Informed Consent: Ensuring that patients are fully aware of the biotechnological agents introduced into their bodies remains a cornerstone of ethical medical practice.
- Accessibility Issues: As mRNA therapies gain popularity, concerns arise regarding who benefits from these innovations and whether they are equitably distributed across populations.
Unique features tied to ethical considerations often focus on the balance between potential benefits and risks. The advantages here lie in fostering responsible research, but the disadvantages could lead to societal divides if not addressed.
"The conversation around ethical implications is just as important as the science itself; after all, the ultimate goal is to benefit humanity as a whole."
In summary, the future of mRNA research holds extraordinary promise. Yet, it is imperative to traverse this path cautiously, paying heed to both innovations that lie ahead and the challenges that may thwart progress.
Closure
In wrapping up our exploration into the multifaceted roles of messenger RNA (mRNA), it's evident that mRNA stands as a pivotal player in the complex landscape of cellular biology. Its significance cannot be overstated, as it facilitates the crucial process of protein synthesis, acting as the messenger between DNA and ribosomes, where proteins are synthesized. This role is not merely functional; it underscores the very fabric of life, guiding how organisms grow, respond, and adapt in diverse environments.
Summary of Key Points
To summarize, we have touched upon several key elements throughout this article:
- mRNA’s Central Role: mRNA is indispensable for the translation of genetic information into functional proteins.
- Diversity of mRNA Types: Different types of mRNA exist, each serving distinct functions, whether coding or non-coding.
- Gene Regulation: The intricate mechanisms of gene expression regulation, both at transcriptional and post-transcriptional levels, further underscore the complexity of mRNA’s role in cellular dynamics.
- Clinical Relevance: The link between mRNA and various diseases, especially in the contexts of cancer and viral infections, highlights the importance of mRNA research in developing therapeutic strategies.
- Biotechnological Innovations: Advances like mRNA vaccines represent groundbreaking shifts in how we approach disease prevention and treatment.
- Future Directions: As we gaze into the future of mRNA research, the potential for new discoveries continues to expand, hinting at the capability for mRNA technologies to revolutionize medicine and biotechnology.
The Future of mRNA in Science
Looking ahead, the future of mRNA within the scientific community appears bright, yet filled with challenges. While the advent of mRNA vaccines has demonstrated the technology's immense potential, various factors must be navigated carefully:
- Stability Concerns: mRNA molecules are inherently unstable, which limits their effectiveness in some applications. Continued research is vital to enhance their longevity and reliability in therapeutic contexts.
- Ethical Considerations: As with any rapidly evolving technology, ethical questions regarding the manipulation of genetic materials loom large. Transparency, coupled with comprehensive regulatory frameworks, will be crucial in addressing these concerns.
- Innovative Applications: New methods of using mRNA, such as in cancer treatments or personalized medicine, are under exploration. These applications promise to tailor interventions to individual patients, moving away from a one-size-fits-all approach.
As we venture further, the interplay of mRNA research with genomic studies will likely unearth insights that refine our understanding and open new therapeutic pathways. Clearly, mRNA is not just a molecular messenger but a dynamic player in the orchestrated symphony of life, shaping the future of science in profound ways.