The Hydrogen Making Process: Key Production Techniques
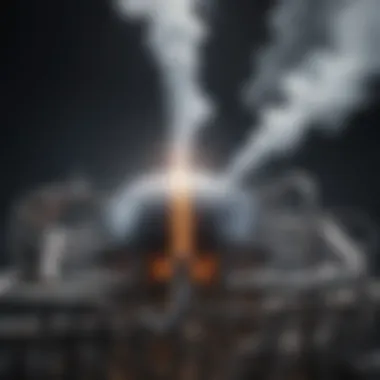
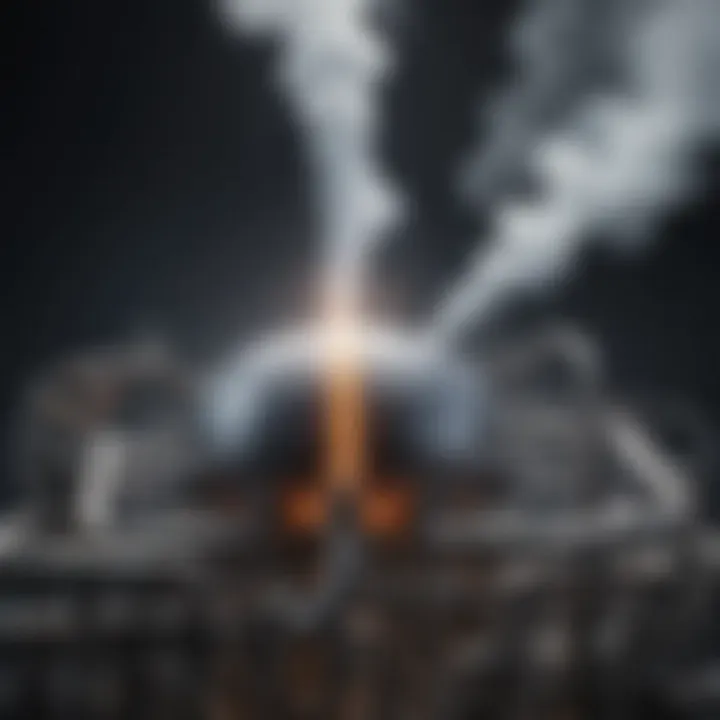
Intro
Hydrogen is gaining attention in today’s energy discussions due to its potential to transform energy systems globally. The methods of hydrogen production are numerous, each with its own unique advantages and drawbacks. As energy needs evolve, understanding these methods becomes essential.
This article presents a detailed examination of hydrogen production methods, aiming to elucidate the scientific processes behind them. By exploring steam methane reforming, electrolysis, and biomass gasification, it offers insights into the implications of hydrogen as an energy resource.
Research Overview
Summary of Key Findings
The landscape of hydrogen production is continually evolving. Here are key findings:
- Steam Methane Reforming (SMR) is the most common method today, converting natural gas into hydrogen and carbon dioxide. Its efficiency makes it widely used, but it is carbon-intensive.
- Electrolysis involves splitting water into hydrogen and oxygen using electricity. This method can be cleaner if powered by renewable energy, suggesting a more sustainable future for hydrogen production.
- Biomass Gasification offers another route by converting organic materials into hydrogen-rich gas. This method can contribute to a circular economy but faces challenges such as feedstock availability.
Importance of the Research
Understanding hydrogen production is not just an academic exercise. It has practical implications for industries aiming to reduce carbon footprints. Researchers, educators, and professionals must grasp these processes to innovate effectively in energy solutions. Enhanced hydrogen production methods could play a critical role in mitigating climate change.
Methodology
Study Design
This article utilizes a comprehensive review of current literature on hydrogen production. It analyzes peer-reviewed studies, industry reports, and regulatory frameworks. By compiling data from multiple sources, it forms a holistic view of the hydrogen production landscape.
Data Collection Techniques
Data was collected through:
- Reviewing academic journals like International Journal of Hydrogen Energy.
- Exploring reports from organizations such as the International Energy Agency.
- Analyzing recent advancements in hydrogen technologies via platforms like ResearchGate and Google Scholar.
By employing these techniques, the research highlights significant advancements and existing challenges, facilitating an enriched understanding of hydrogen as an emergent energy source.
Prolusion to Hydrogen Production
The production of hydrogen holds significant relevance in today’s energy landscape due to its versatility and potential for sustainability. As global efforts toward reducing carbon emissions gather pace, hydrogen emerges as a cornerstone in the transition to cleaner fuels. This section seeks to elucidate the importance of hydrogen production, accentuating its role in various sectors and the environmental benefits it offers.
The Importance of Hydrogen
Hydrogen is the most abundant element in the universe and possesses unique attributes that make it an appealing candidate for energy solutions. First, it can be generated from a variety of sources, including natural gas, water, and biomass. This flexibility allows for diverse approaches in production methods. Furthermore, when used as a fuel, hydrogen produces only water vapor as a byproduct, thus mitigating harmful emissions that are prevalent with fossil fuels. The quest for a sustainable energy future necessitates a robust system for hydrogen production that can adapt to rapidly evolving technological landscapes.
Hydrogen’s potential lies not only in its capacity to generate energy but also in its ability to store energy from renewable sources.
Applications of Hydrogen
Hydrogen has numerous applications across various industries, reflecting its adaptability and essential role in the energy transition.
- Fuel Cells: Hydrogen is a critical component in fuel cell technology, which converts hydrogen and oxygen into electricity. This application is especially crucial in the transportation sector, where zero-emission vehicles are gaining traction.
- Industrial Uses: Hydrogen serves as a feedstock in chemical processes, notably in ammonia production for fertilizers, which is vital for global agriculture. It also finds applications in refining gasoline and as a reducing agent for metals.
- Energy Storage: As energy storage mechanisms evolve, hydrogen is increasingly recognized for its ability to store excess energy generated from renewable sources. This properties enables a more reliable energy supply, even when production fluctuations occur.
The importance and wide-ranging applications of hydrogen underscore the necessity of understanding the processes behind its production. Through systematic exploration of various production methods, the implications for energy systems and environmental sustainability become increasingly clear, thus laying the groundwork for future advancements.
Overview of Hydrogen Properties
Understanding the properties of hydrogen is fundamental to comprehending its role in energy systems and production methods. Hydrogen, with its unique characteristics, is becoming increasingly pivotal in discussions surrounding energy sustainability and innovative technologies. Several aspects of hydrogen need to be taken into account, such as its chemical and physical properties, and its eventual integration into various energy systems. This section provides a foundational overview of those properties, which ultimately enhances our grasp of hydrogen production and its far-reaching implications.
Chemical and Physical Properties
Hydrogen is known for its simplicity as the lightest and most abundant element in the universe. It exists primarily as a diatomic molecule (H₂), which is a stable form under normal conditions. This diatomic nature leads to various significant properties:
- Low Density: Hydrogen is the least dense gas, which influences storage and transport strategies.
- High Energy Content: When combusted, hydrogen yields a substantial amount of energy, approximately 120 MJ/kg. This makes it an attractive fuel alternative.
- Non-Toxicity: Hydrogen is non-toxic, making it safer compared to traditional fuels, which often release harmful pollutants.
- Flammability: Although flammable, hydrogen's combustion does not produce carbon emissions, which is crucial for reducing greenhouse gases.
These features present both advantages and considerations in the innovation and implementation of hydrogen production processes. Understanding these properties helps in engineering effective production methods and provides insight into safety standards required in its handling.
Role in Energy Systems
The role of hydrogen in energy systems cannot be understated. As the world strives to transition from fossil fuels to more sustainable energy sources, hydrogen emerges as a crucial component. It has various functions within energy systems:
- Energy Carrier: Hydrogen can store energy produced from renewable sources. It can be converted back to electricity when needed through fuel cells, bridging the gap between production and consumption.
- Decarbonization Agent: As industries look to decarbonize, hydrogen provides a pathway. It can replace fossil fuels in processes such as steel manufacturing, where carbon emissions are traditionally high.
- Grid Stability: Hydrogen can help enhance grid stability by acting as a buffer for excess electricity from renewable sources, thus addressing intermittency challenges.
In summary, the characteristics and roles of hydrogen lay the groundwork for its potential as a vital part of a sustainable energy future. The focus on these properties is essential for researchers and industry professionals who are looking to explore new avenues in hydrogen production and application.
"Hydrogen has the potential to transform the energy landscape, serving both as a clean energy carrier and a crucial material for significant industrial processes." - Industry Experts
As we move forward in this analysis of hydrogen production methods, the insights provided in this section will serve to elevate our understanding and present a well-rounded view of hydrogen's essential attributes.
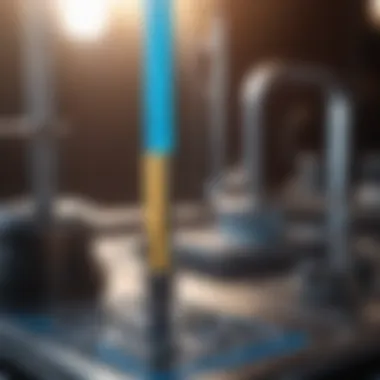
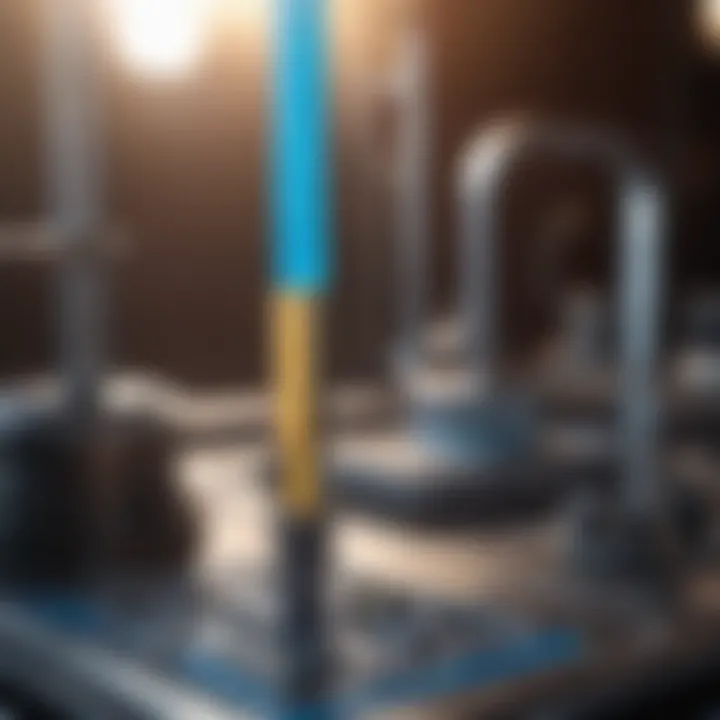
Methods of Hydrogen Production
Methods of hydrogen production are central to understanding how this element fits into the current energy landscape. The methods vary widely, each with unique features that influence efficiency, sustainability, and economic viability. Evaluating these methods entails more than just technical feasibility; it involves considering their implications for energy policy, environmental health, and technological advancement.
The choice of production method is influenced by the available resources, economic factors, and environmental regulations. Therefore, comprehending these methods helps in making informed decisions about hydrogen's future role.
Steam Methane Reforming
Process Description
Steam Methane Reforming (SMR) is the most prevalent method for hydrogen production. It involves a chemical reaction between natural gas and water vapor, producing hydrogen and carbon monoxide. The primary reaction typically occurs at high temperatures, around 700 to 1,000 degrees Celsius, using a catalyst.
This method is favored due to its established infrastructure and ability to produce large quantities of hydrogen. It forms a significant part of the current hydrogen economy, hence its inclusion in this article.
One unique characteristic of SMR is its ability to be combined with carbon capture and storage technologies, which can mitigate its greenhouse gas emissions.
Advantages and Disadvantages
The main advantage of SMR is its efficiency and relatively low cost. This method can produce hydrogen at large scales, making it economically viable, especially in industries that depend heavily on hydrogen. Furthermore, the existing infrastructure supports the method, facilitating wider adoption.
However, notable disadvantages exist as well. The process emits carbon dioxide, a significant contributor to climate change. As the global focus shifts towards sustainable practices, there is a growing concern regarding its environmental impact, which need addressing in future energy strategies.
Environmental Impact
Steam Methane Reforming has a profound environmental impact. While it is often seen as a transitional technology in the shift to clean hydrogen fuel, its reliance on natural gas leads to substantial CO2 emissions. The emissions are particularly concerning due to their contribution to global warming.
However, the application of carbon capture and storage technologies can mitigate some of these effects. Still, these technologies are not universally applied, limiting SMR's overall sustainability appeal.
Electrolysis
Process Description
Electrolysis is a versatile method that uses electricity to split water into hydrogen and oxygen. When powered by renewable sources, it produces green hydrogen, making it a popular choice for sustainability advocates. The technology is characterized by its simplicity and its ability to integrate with renewable energy systems, thus minimizing emissions.
The significance of electrolysis lies in its potential to drive the transition toward a hydrogen economy centered on clean energy. Its role in this article underscores innovative methods of production to meet climate goals.
Types of Electrolyzers
Different types of electrolyzers exist, including alkaline, proton exchange membrane (PEM), and solid oxide electrolyzers. Each type has distinct characteristics, making them suitable for different applications.
- Alkaline Electrolyzers: Established and low-cost but less efficient.
- PEM Electrolyzers: Higher efficiency and faster response times, ideal for fluctuating renewable energy.
- Solid Oxide Electrolyzers: Suitable for high-temperature applications but still in experimental stages.
Understanding these distinctions assists industries in determining which technology aligns best with their operational goals.
Renewable Energy Sources for Electrolysis
The coupling of electrolysis with renewable energy sources is where its potential truly shines. Utilizing solar, wind, or hydro energy, the process becomes carbon-neutral. The value of this methodology lies not just in its production capabilities but in how it harmonizes with global energy systems focused on sustainability.
By tapping into renewable electricity, we can transition to a more sustainable hydrogen production model. However, the variability in renewable energy generation poses challenges to ensuring a consistent supply for electrolysis.
Biomass Gasification
Process Description
Biomass gasification converts organic materials into hydrogen-rich gas by heating them in a low-oxygen environment. This process showcases how biomass can be transformed into a valuable energy source. It can utilize various feedstocks, including agricultural residues and forestry waste.
The importance of biomass gasification lies in its potential to utilize waste, creating a circular economy model. Its relevance to sustainable practices makes it worthy of exploration in this article.
Feedstock Considerations
Choosing appropriate feedstock is crucial in biomass gasification. Factors such as availability, cost, and energy content impact the process's efficiency and economic feasibility.
Nevertheless, competition for feedstock with food supply and across industries may create barriers to biomass utilization. Balancing economic viability and sustainability is essential in the decision-making process.
Technical Challenges
While biomass gasification presents a valuable opportunity, it faces several technical challenges. These include the need for advanced gas cleaning, managing tar production, and ensuring consistent gas quality.
Innovations in technology can help address these issues, thus supporting the further development of biomass gasification in the hydrogen sector.
Thermochemical Water Splitting
Process Overview
Thermochemical water splitting utilizes heat to drive chemical reactions that separate water into hydrogen and oxygen. The process often relies on high temperatures typically achieved through solar concentrators or nuclear reactors. This method represents a novel means of producing hydrogen without relying on fossil fuels.
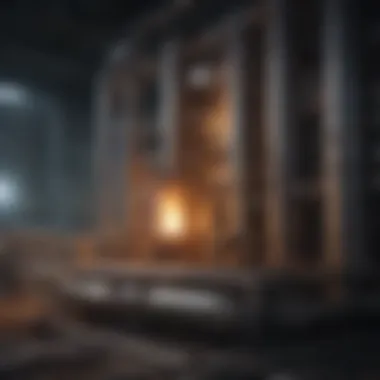
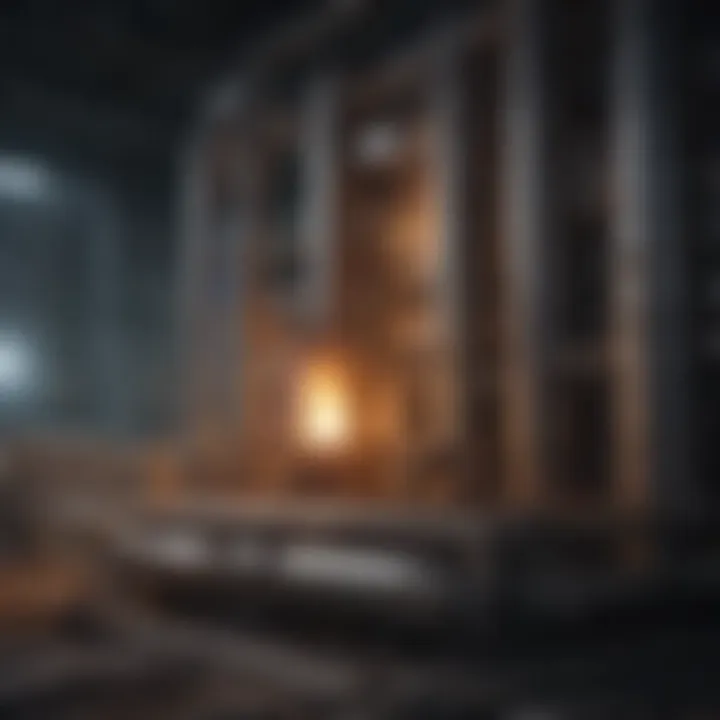
Its potential contribution to hydrogen production ties back to addressing overall energy needs sustainably, which makes it significant in this article.
Material Requirements
Thermochemical processes require materials that can withstand extreme conditions. This necessitates the development of advanced materials that can tolerate high temperatures and corrosive environments. The innovation in materials science is crucial to the implementation of these technologies.
Potential Advantages
The potential advantages of thermochemical water splitting include lower CO2 emissions compared to traditional methods and the ability to utilize abundant thermal energy sources. While still in developmental stages, it promises substantial long-term benefits for hydrogen production.
Photoelectrochemical Water Splitting
Mechanism
Photoelectrochemical water splitting leverages solar energy to directly split water into hydrogen and oxygen. The process uses semiconductor materials that absorb sunlight and create electronic holes and electrons, facilitating water splitting.
Its ability to harness solar energy makes it a compelling alternative in the hydrogen production discourse, particularly in this article.
Current Research Trends
Research in this field aims at improving the efficiency and stability of photoelectrochemical systems. Understanding material composition, enhancing light absorption, and integrating with existing systems are focal points. Continued innovation can propel this method into mainstream use.
Challenges in Implementation
Despite the potential, challenges exist. The high cost of materials, efficiency issues, and scalability remain barriers to widespread implementation. Addressing these hurdles is critical to unlocking the full potential of photoelectrochemical water splitting in hydrogen production.
Comparative Analysis of Production Methods
The evaluation of hydrogen production methods is crucial for identifying the most effective pathways to meet current and future energy needs. By comparing various techniques, we can uncover strengths and weaknesses that will influence decisions in energy policy, industrial investment, and environmental sustainability. Comprehensively analyzing efficiency, costs, and environmental implications helps stakeholders understand which methods align with their goals in fostering a hydrogen economy.
Efficiency and Yield
Efficiency refers to how effectively a given production method converts raw materials into hydrogen. Yield denotes the amount of hydrogen produced per unit. For instance, steam methane reforming typically offers high yields but has lower efficiency when considering the fossil fuel inputs. In contrast, electrolysis can achieve high efficiency under optimal conditions, especially when powered by renewable energy sources.
It's important to recognize that advancements in technology continuously shift these metrics. For example, new catalysts and optimized operational parameters can significantly enhance yields in methods like biomass gasification or photoelectrochemical water splitting. Efficient production not only increases the available supply but can also reduce costs associated with hydrogen production.
Cost Considerations
The financial aspect of hydrogen production remains a central factor in its commercial viability. Each method incurs different capital and operational expenditures. Steam methane reforming, while currently cheap due to established infrastructure, can be affected by fluctuations in natural gas prices.
On the other hand, technologies like electrolysis require substantial initial investments, primarily due to electrolyzer costs and supporting renewable energy infrastructure. Recent trends suggest that as renewable energy becomes cheaper and more prevalent, electrolytic hydrogen may see decreased costs in the long term. Furthermore, governments are increasingly providing incentives for clean hydrogen technologies, which may alter cost structures positively.
Critical factors that influence cost include:
- Source of materials
- Energy input and operational efficiency
- Research and developmental breakthroughs
- Regulatory policies and tax incentives
Environmental Implications
The environmental impact of hydrogen production methods cannot be overlooked. Each technique presents a unique set of challenges and benefits.
- Steam methane reforming, while economically feasible, generates significant CO2 emissions, compounding climate change issues.
- On the contrary, electrolysis and biomass gasification can potentially provide low-carbon or carbon-neutral hydrogen, depending on the energy sources used and carbon capture technologies employed.
- Methods such as thermochemical water splitting hold the promise of utilizing solar or nuclear power with minimal emissions, making them particularly attractive.
Assessing environmental implications necessitates a life-cycle analysis. This examination requires consideration of greenhouse gas emissions, resource depletion, and other ecological factors. Ultimately, selecting a hydrogen production method has wide-ranging implications for both energy systems and climate goals.
"Hydrogen energy represents both an opportunity and a challenge. Understanding production methods helps us to harness its full potential while mitigating negative impacts."
Innovations and Future Directions
The landscape of hydrogen production is undergoing rapid transformation due to advancements in technology and changing energy dynamics. Innovations in this sector are crucial for optimizing hydrogen production processes and making them more sustainable. These innovations not only aim to increase efficiency but also address environmental concerns and economic feasibility. Here, we explore several key areas that are shaping the future of hydrogen production.
Advances in Catalytic Processes
Catalysis plays a vital role in enhancing the efficiency of hydrogen production. Traditional methods, like steam methane reforming, rely heavily on specific catalysts that often require high temperatures or pressures. New catalytic processes, however, are emerging that promise to increase hydrogen yield while minimizing energy input and cost.
Recent developments in nanotechnology have provided new opportunities for improving catalysts. Nanostructured materials have shown higher activity levels due to increased surface area. This means that less material can achieve better performance. Moreover, research has focused on developing catalysts that can operate effectively at lower temperatures. This not only saves energy but also reduces carbon emissions associated with hydrogen production. Key players in the field are experimenting with new metal alloys and oxide-based catalysts to push the boundaries of current methods.
Integration with Renewable Energy
The integration of hydrogen production with renewable energy sources is essential for creating a sustainable hydrogen economy. Electrolysis, which splits water into hydrogen and oxygen, is particularly relevant in this context. By harnessing energy from sources like solar panels or wind turbines, hydrogen can be produced without the carbon emissions associated with fossil fuels.
This synergy enhances the appeal of hydrogen as an energy carrier. During times of excess renewable energy production, electrolysis can be ramped up to generate hydrogen, which can be stored or used later. This method not only curtails waste but also stabilizes energy supply. Continued investments and innovations in this area could potentially lead to a more flexible and resilient energy infrastructure.
Policy and Economic Considerations
The future of hydrogen production also depends significantly on the policy landscape and economic incentives. Governments worldwide are beginning to recognize hydrogen's potential in the transition to a low-carbon economy. Therefore, supportive policies such as grants, subsidies, and research funding are becoming increasingly vital for advancing hydrogen technologies.
Economic considerations include the costs associated with new technologies and infrastructure. While advancements in production methods can lower costs, the initial investment in infrastructure remains a barrier. Policymakers need to create frameworks that encourage public-private partnerships to foster innovation and improve economics.
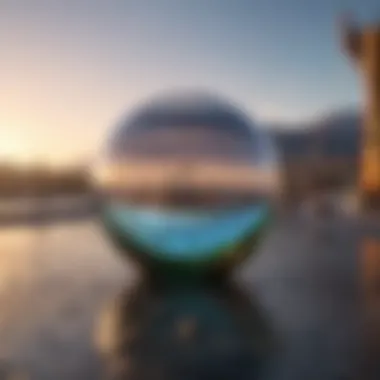
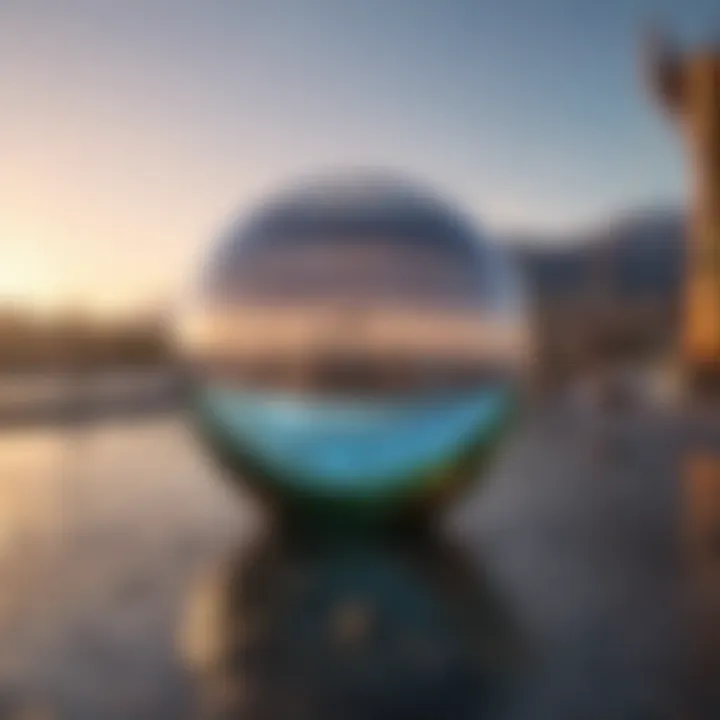
The effective collaboration between governments, researchers, and industries will determine how quickly we can scale hydrogen production and integrate it into existing energy systems.
In summary, advancements in catalytic processes, integration with renewable energy, and supportive policies are pivotal in shaping the future of hydrogen production. These innovations not only promise to enhance efficiency and sustainability but also position hydrogen as a key player in the energy transition.
Challenges in Scaling Hydrogen Production
Scaling hydrogen production is critical for its future role in the global energy landscape. The various methods of hydrogen production possess unique advantages, yet they also present challenges that need addressing. Issues like infrastructure limitations, market dynamics, and technological barriers must be understood to fully realize hydrogen's potential as an energy source. Identifying and overcoming these challenges can enhance hydrogen's viability and drive significant progress toward a sustainable future.
Infrastructure Limitations
Infrastructure is a key component in the hydrogen production and distribution process. Currently, the existing network for natural gas and electricity does not adequately support large-scale hydrogen production or its distribution. This situation presents both logistical and economic hurdles.
To build the necessary infrastructure for hydrogen, significant investments are required. This includes pipelines, refueling stations, and storage facilities specifically designed for hydrogen. Traditional facilities may not be suitable due to hydrogen's unique properties, such as its low density and small molecular size, which present challenges related to leakage and material compatibility.
- Hydrogen pipelines: Existing natural gas pipelines can be adapted, but this requires careful consideration of material compatibility and safety measures.
- Refueling stations: Expansion of hydrogen refueling stations is essential to promote the use of hydrogen fuel cell vehicles.
- Reforming facilities: New facilities could support hydrogen production from renewable sources, though their establishment involves regulatory approvals and infrastructure planning.
The lack of a comprehensive hydrogen infrastructure can limit market growth and technology adoption.
Market Demand and Supply
The market dynamics surrounding hydrogen production are influenced by demand and supply. While the interest in hydrogen as a clean energy source is increasing, actual market demand remains uncertain. Several factors contribute to this scenario:
- Consumer Awareness: Limited understanding of hydrogen's benefits may hinder its adoption in sectors like transportation and power generation.
- Investment in Technology: Industries may hesitate to invest in hydrogen technologies without clear market signals. As a result, scaling up production becomes difficult.
- Competing Technologies: Alternatives such as battery electric vehicles and other renewable technologies also vie for attention and investment, complicating hydrogen's position.
For hydrogen production to scale effectively, significant market demand must be established. This could be achieved through government policies supportive of hydrogen initiatives.
Technological Barriers
Technological advancements are essential for overcoming the barriers to hydrogen production. Despite various methods being available, efficiency and scalability remain concerns. Some notable issues include:
- Production Costs: The high costs associated with methods like electrolysis limit their current application.
- Energy Efficiency: Some technologies do not yet provide adequate energy conversion efficiencies compared to fossil fuels, making them less appealing economically.
- Research and Development: Continued innovation is required to improve existing technologies and develop new ones that are both cost-effective and environmentally friendly.
Overall, tackling these technological barriers is vital for scaling hydrogen production effectively. Promoting research initiatives and partnerships can enhance innovation in this sector.
"Investments in advanced research are critical for addressing the technological barriers that currently limit hydrogen production efficiency and commercial viability."
Impact on the Energy Landscape
The emergence of hydrogen as a critical energy carrier has reshaped traditional perspectives on energy systems. Understanding the impact of hydrogen on the energy landscape reveals its potential to contribute significantly to the global energy transition. By integrating hydrogen into various sectors, we can address pressing challenges such as climate change and energy security.
Hydrogen economy potential refers to the concept of a system where hydrogen serves as a key energy carrier in multiple applications. Hydrogen can be produced using different methods, enabling its versatility across industries. For instance, it can be utilized in fuel cells to generate electricity without producing harmful emissions. Additionally, hydrogen serves as a high-energy-density fuel for transportation and industrial processes, thereby fulfilling diverse energy needs efficiently.
- Versatility: Hydrogen can complement or replace fossil fuels in many applications.
- Storage: It can store excess renewable energy, helping to balance supply and demand.
- Transport: Hydrogen infrastructure can facilitate global energy trade.
Hydrogen Economy Potential
The hydrogen economy potential is vast. By harnessing hydrogen, countries can enhance their energy independence and diversify their energy mix. Several nations are investing heavily in hydrogen infrastructure to realize this potential.
One of the critical benefits of a hydrogen economy is its ability to support renewable energy sources. Hydrogen can be created from renewable energy through electrolysis, ensuring that energy production is sustainable and carbon-neutral. This synergy allows renewable sources, like wind and solar, to generate hydrogen when production exceeds demand.
Efficient hydrogen production methods are crucial for scaling this economy. Policymakers and industry leaders must collaborate to develop technologies that enhance production efficiency while reducing costs. The importance of a supportive regulatory framework cannot be overlooked either. Incentives for sustainability-driven projects will accelerate growth in this sector.
Role in Decarbonization
Hydrogen plays a pivotal role in decarbonization efforts worldwide. As countries strive to meet climate goals, hydrogen emerges as a viable solution to reduce greenhouse gas emissions. It can displace carbon-intensive fossil fuels, particularly in hard-to-abate sectors, such as heavy industry and freight transport.
- Industrial Applications: Hydrogen can be utilized in steel and cement production, reducing reliance on coal and significantly cutting emissions.
- Transportation: Hydrogen fuel cell vehicles produce no tailpipe emissions, thereby contributing to cleaner urban air.
Recent studies emphasize hydrogen's ability to facilitate a broader transition to a sustainable energy future. Integrating hydrogen into existing systems offers a pathway to achieve net-zero emissions without compromising economic growth. For this transformation to be effective, investment in infrastructure and technology development remains paramount. Significant strides in research and innovations will bolster hydrogen applications further.
Hydrogen represents a crucial component of the strategy for achieving greenhouse gas reduction targets. Its role in supporting a sustainable future is set to expand as technology advances and infrastructure improves.
Epilogue and Future Prospects
The importance of evaluating the conclusion and future prospects of hydrogen production cannot be understated. The significance of hydrogen as an energy source is growing rapidly, with various sectors increasingly adopting it. The transition to hydrogen has implications for energy policy, environmental sustainability, and economic growth. As we analyze the findings of this article, it becomes clear that the path forward for hydrogen will be shaped by advancements in production methods, regulatory frameworks, and market acceptance.
Summary of Findings
The examination of hydrogen production methods exposes several critical elements:
- Variety of Production Techniques: The article explored different methods such as steam methane reforming, electrolysis, biomass gasification, and thermochemical processes. Each technique has unique advantages, challenges, and environmental implications.
- Comparative Analysis: It detailed efficiency, yield, cost, and environmental impact, providing insights into how these factors play a role in the adoption of each method in different industries and applications.
- Innovations and Future Directions: Advances in technology and integration with renewable energy sources have shown promise in enhancing the sustainability and economic viability of hydrogen production.
- Challenges in Scaling: Infrastructure, market demand, and technological barriers pose significant challenges. Addressing these obstacles is crucial for realizing a hydrogen economy.
This summary highlights that the future of hydrogen as a key energy source depends on technological innovation and strategic policy decisions.
Final Thoughts
Looking ahead, the role of hydrogen in the global energy landscape is both promising and complex. With its potential to contribute to decarbonization and energy security, the push towards hydrogen must be driven by a clear vision of its integration into existing systems. Continued research and investment are vital to unlock its full potential.
As industries and policymakers navigate these waters, they must prioritize sustainable practices and innovative solutions. In doing so, they can ensure that hydrogen not only supports economic growth but also aligns with the global agenda for a cleaner and more sustainable future.
"Hydrogen presents an opportunity not only as a fuel source but also as a means of addressing climate change, provided we address the associated challenges effectively."