Thermally Conductive Materials: Properties and Innovations
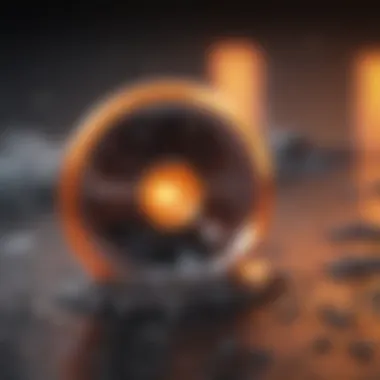
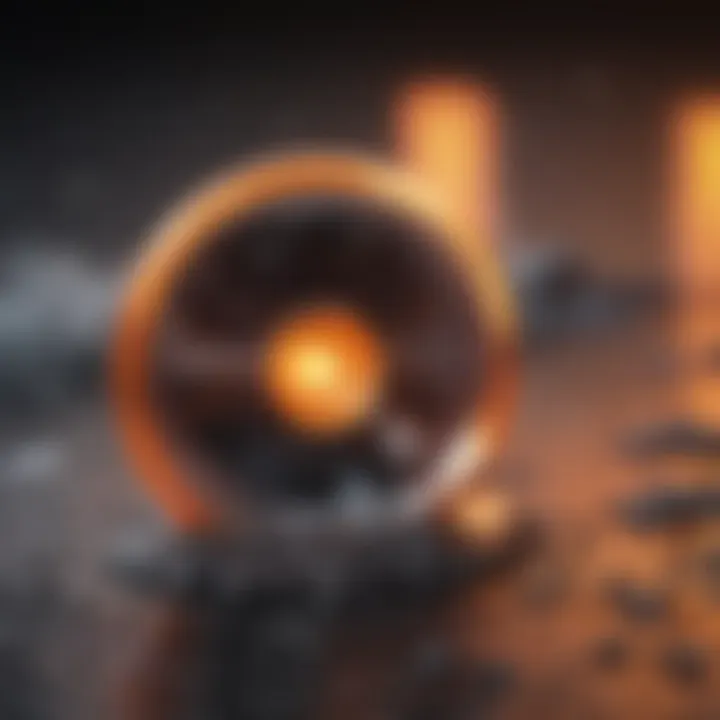
Research Overview
Understanding thermal conductivity is fundamental to a variety of fields, from electronics to construction. Thermal conductivity dictates how efficiently heat can be transferred through a material. Materials with high thermal conductivity, like copper and aluminum, are prized in numerous industries for their ability to disperse heat quickly, while those with low conductivity, such as silicone foams or fiberglass, serve as effective insulators.
Summary of Key Findings
The research focused on several pivotal aspects of thermal conductive materials:
- Material Types: A diverse range of materials including metals, ceramics, composites, and polymers were reviewed for their thermal properties.
- Innovation in Applications: Technologies such as thermal interface materials and heat sinks are highlighted, demonstrating how innovations are addressing the challenges of thermal management.
- Emerging Trends: New synthetic materials, like graphene and carbon nanotubes, are emerging as frontrunners in thermal applications due to their exceptional properties.
"The role of thermal conductivity in advancing technology cannot be understated; it's the glue that binds innovation to functionality."
Importance of the Research
The significance of thermal conductivity in materials cannot be overstated. In electronics, the heat generated by components can lead to failure if not properly managed. Thus, materials with high thermal conductivity are essential for efficient device performance. In construction, understanding how heat moves through materials impacts energy efficiency and comfort in buildings.
Methodology
An in-depth examination of the properties and applications of thermally conductive materials requires a systematic approach to research and data collection.
Study Design
The research employed a mixed-methods design that includes both qualitative and quantitative analyses. It blended laboratory experiments with real-world application assessments to ensure a comprehensive understanding of material behaviors under various conditions.
Data Collection Techniques
Data were collected using a variety of techniques:
- Experimental Testing: In controlled environments, thermal conductivity tests were conducted using techniques such as the laser flash analysis.
- Surveys and Interviews: Engaged with industry professionals to ascertain practical challenges faced with current materials and desired properties for future developments.
- Literature Review: Analyzed existing academic research and patents to understand the evolution of thermally conductive materials and their applications.
Through these methodologies, insights into the properties and innovations of thermal conductive materials were gleaned, shaping our understanding and paving the way for future advancements in the field.
Prolusion to Thermal Conductivity
Thermal conductivity plays a significant role in both our everyday lives and advanced technological applications. Understanding how heat transfers through different materials can lead to innovations in multiple fields, from electronics to environmental science. Without grasping the core concepts of thermal conductivity, individuals may miss the broader implications of material selection and utilization, potentially leading to inefficiencies or failures in various applications. This section serves to lay the foundation for comprehending thermal conductivity, its historical evolution, and how it shapes our modern world.
Definition of Thermal Conductivity
Thermal conductivity is essentially a measure of a material's ability to conduct heat. It is quantified as the amount of heat that passes through a unit thickness of the material over a certain time period under a given temperature gradient. Typically, materials with high thermal conductivity, like copper or aluminum, facilitate rapid heat transfer. Conversely, low conductivity materials, such as rubber or wood, impede heat flow. This property is measured in watts per meter-kelvin (W/m·K).
To put it simply, if you imagine a metal rod heated on one end, the speed at which the heat travels to the other end gives insight into its thermal conductivity. The higher the thermal conductivity, the quicker the heat travels. This aspect is integral across industries, informing product design, safety standards, and energy efficiency.
Historical Context and Development
The journey of understanding thermal conductivity can be traced back to the late 19th century. One notable figure is Jean-Baptiste Joseph Fourier, whose theories on heat conduction laid groundwork for modern thermal analysis. Fourier's law states that the rate of heat transfer through a material is directly proportional to the area and the temperature difference across it. His work provided essential framework that encouraged further research and development.
As the field of thermodynamics evolved, the importance of thermal conductivity in practical applications became clearer. For example, during the industrial evolution, engineers sparked interest in materials that could withstand high temperatures, particularly in steam engines and, later, internal combustion engines. This led researchers to explore metals, ceramics, and advanced composites, each bringing unique properties to the table.
Today, rigorous studies continue to advance our understanding of heat transfer. Innovations like nanotechnology have opened new avenues for enhancing thermal conductivity, challenging existing notions of material performance. As a result, industries have reevaluated how they approach heat management, impacting everything from energy systems to consumer electronics.
In short, without the historical context of thermal conductivity, many of the advancements we see today in materials science and engineering would not be possible. Its evolution highlights the synergy between theoretical inquiry and practical application, helping define the way we use thermally conductive materials in various aspects of life.
Fundamentals of Heat Transfer
Understanding the fundamentals of heat transfer is critical when exploring thermally conductive materials. It sets a foundation for appreciating how different materials interact with heat and explains why certain materials excel in specific applications. The various mechanisms of heat transfer—conduction, convection, and radiation—play pivotal roles in the performance and utility of these materials in real-world scenarios.
Conduction, Convection, and Radiation
Heat transfer can occur in several ways, notably through conduction, convection, and radiation. Each method has unique characteristics:
- Conduction involves heat flowing through a material without any movement of the material itself. This process is dictated by the material's thermal conductivity. Metals, for instance, are renowned for their ability to conduct heat due to tightly packed atoms that facilitate efficient energy transfer.
- Convection refers to heat transfer via the movement of fluids—both liquids and gases. Fluids absorb heat at one point and convey it to another through currents. Think of how hot air rises and cooler air sinks; this natural cycle is a prime example of convection in action, particularly in heating systems or cooling fans.
- Radiation, on the other hand, occurs when heat is transferred through electromagnetic waves. Unlike conduction and convection, radiation does not require a medium. For example, sunlight heating your skin is radiation at work. Every material emits infrared radiation, which can be harnessed for various applications.
All of these processes reveal the complexity of managing heat in different environments, showcasing why understanding these fundamentals is essential for the effective development and use of thermally conductive materials.
The Role of Temperature Gradients
Temperature gradients are vital in the study of heat transfer. A gradient refers to the difference in temperature between two areas, and it's this difference that drives the flow of heat. The greater the temperature difference, the more rapid the heat transfer. This principle is crucial in designing systems that rely on efficient thermal management.
For instance, in electronic devices, maintaining proper temperature gradients can prevent overheating. Engineers must consider the materials' thermal conductivity to ensure heat dissipates effectively, allowing components to operate within safe limits.
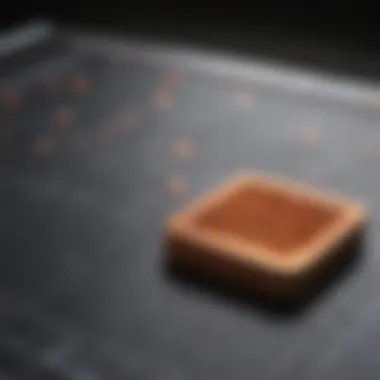
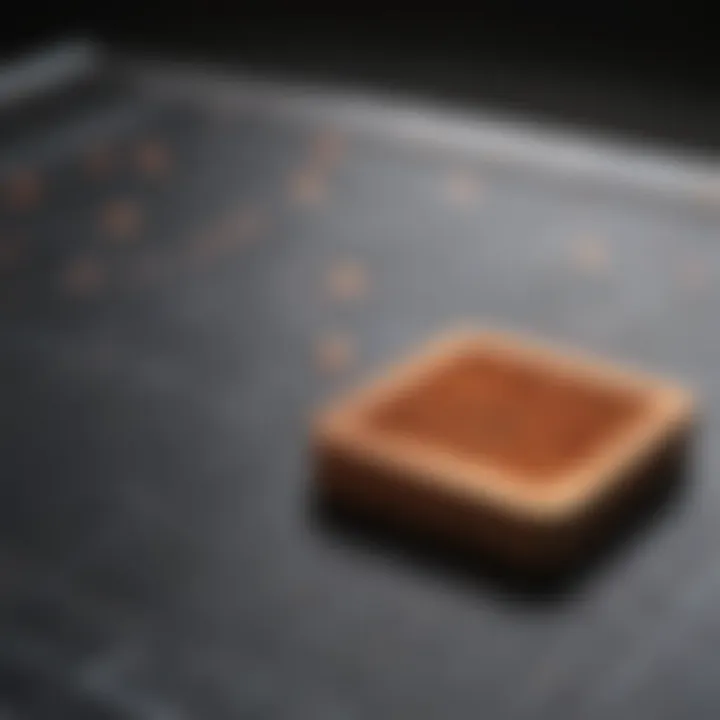
Understanding these gradients also plays a role in natural systems. In ecology, the interaction of air and water temperatures impacts climate zones, affecting both local flora and fauna.
In summary, mastering the fundamentals of heat transfer not only aids in selecting the right thermally conductive materials, but also equips researchers and professionals to tackle various challenges in heat management. These concepts directly inform innovations that could transform industries as diverse as electronics, construction, and aerospace.
"Thermal management is not just about using the right materials; it's understanding the science behind how heat moves to create efficient, effective solutions."
Grasping these fundamentals empowers professionals to make informed decisions that leverage thermal conductivity in diverse applications.
Types of Thermally Conductive Materials
Understanding the diverse range of thermally conductive materials is crucial for anyone diving into the realm of thermal management. These materials serve distinct purposes across various sectors, and their unique properties can determine the efficiency of heat transfer in applications ranging from electronics to construction. Knowing the different types also allows for more informed choices when designing systems involving heat dissipation or insulation. Think of it like choosing the right tool for the job; having the right material can mean the difference between success and failure in thermal performance.
Metals and Their Thermal Properties
Metals are often the go-to materials for thermal conduction, and it's not hard to see why. Copper and aluminum stand out prominently due to their exceptional thermal conductivity. Copper pulls ahead with a conductivity of about 401 W/m·K, making it a favorite for applications such as heat sinks and electrical wiring. On the other hand, aluminum, offering about 237 W/m·K, is widely used in applications where weight is a factor, like in aerospace structures.
Metals, in general, exhibit high thermal conductivity because they possess free electrons. This characteristic allows them to transfer heat rapidly and evenly. However, it's worth noting that not all metals are created equal; some, like stainless steel, possess lower thermal conductivity, which is advantageous when insulation is necessary.
Ceramics as Conductive Materials
When you think of ceramics, you might picture fragile pottery. However, some advanced ceramics defy this stereotype by exhibiting significant thermal conductivity. Materials like alumina and silicon carbide are notable examples. These ceramics can handle high temperatures, making them suitable for applications in electronics and as heat shields in aerospace. They provide durability and stability in extreme conditions, which is a huge advantage in many scenarios.
But, there's a trade-off. While they are resistant to thermal shock and boast impressive structural integrity, ceramics can be brittle. Thus, their applications often require careful consideration regarding wear and tear.
Polymers with Enhanced Thermal Conductivity
Polymers typically aren't known for their thermal conductivity, but recent innovations in this area are quite remarkable. Conventional polymers have poor thermal conductivity, usually below 0.5 W/m·K. However, modifications such as incorporating conductive fillers can dramatically improve their properties. Filled with materials like carbon black or graphite, these polymers can achieve thermal conductivities surpassing 10 W/m·K. This feature makes them appealing for uses ranging from thermal interface materials in electronic devices to lightweight components in automotive applications.
While these enhanced polymers provide functional benefits, one should also consider factors such as cost and the potential impact on material flexibility. It’s always a balancing act, but when executed well, enhanced polymers represent a sweet spot in thermal application design.
Novel Nanomaterials
The field of nanotechnology has introduced innovative materials that change the landscape of thermal conductivity. Graphene, for instance, is one of the most prominent nanomaterials known for its unbelievable thermal conductivity, often exceeding that of metals. Because it consists of a single layer of carbon atoms arranged in a two-dimensional lattice, this material can facilitate an efficient heat transfer process that can revolutionize thermal management strategies.
Additionally, nanowires and carbon nanotubes are gaining traction for their unique properties. These materials can also be embedded in traditional matrices to create composites with tailored thermal characteristics. Furthermore, the ability to manipulate their structure at the nanoscale opens up a world of possibilities for engineers and developers looking to enhance thermal performance.
In summary, navigating the variety of thermally conductive materials propels understanding and innovation in heat management solutions. From traditional metals to groundbreaking nanomaterials, each type has distinct advantages and applications that cater to a plethora of industrial needs.
"Choosing the right thermally conductive material is paramount to optimizing performance in even the smallest of applications."
By paying attention to specific characteristics, properties, and contextual needs, one can utilize these materials efficiently in real-world scenarios.
Measuring Thermal Conductivity
Understanding how to measure thermal conductivity is crucial in both theoretical and practical contexts. This measurement not only helps researchers characterize materials but also informs engineers and manufacturers regarding the suitability of materials for various applications. Accurate measurement enables designers to select the right materials based on thermal performance for tasks ranging from heat dissipation in electronics to insulation in building structures. The significance of precise measurements extends into energy efficiency; it guides the decisions that affect the overall thermal management of systems in diverse fields.
Methods of Measurement
Thermal conductivity can be assessed utilizing a variety of methods. Each method comes with its own advantages and limitations, determining the choice based on the specific circumstances and material types. Here are some key methods:
- Guarded Hot Plate Method: This is a classic approach for solids and measures thermal conductivity by creating a steady-state condition. The method uses a hot plate and a cold plate to maintain a temperature difference and quantify heat flow through the sample.
- Heat Flow Meter: This apparatus measures the heat flow through a specimen placed between two temperature-controlled plates. It’s especially beneficial for insulation materials, providing rapid results in a non-destructive manner.
- Transient Method (e.g., Laser Flash Analysis): This technique involves heating a sample for a short period and analyzing how long it takes for the heat propagation to be detected. It’s effective for small samples and is suitable for various materials, including those that are difficult to measure with traditional methods.
- Oxide Thermal Conductivity Analyzers: A newer technology, it uses a non-contact method to assess thermal properties, allowing for a broader range of applications without altering the sample significantly.
Each of these methodologies is tailored to fit particular industry requirements, from building materials to advanced composites. Therefore, understanding the nuances of each is paramount.
Understanding Thermal Conductivity Values
Thermal conductivity values are a key indicator of how efficiently a material can conduct heat. These values are expressed in watts per meter-kelvin (W/m·K). The interpretation of these numbers can vary depending on the material and its intended use. Understanding these values helps engineers and scientists predict the behavior of materials in real-world applications.
Generally, the higher the thermal conductivity, the better the material is at conducting heat. For instance, metals like copper can have thermal conductivity values well over 400 W/m·K, making them ideal for electrical components or heat sinks. In contrast, materials like polystyrene can have values as low as 0.03 W/m·K, making them suitable for insulation purposes.
"Materials with high thermal conductivity are critical in applications where efficient heat transfer is necessary, while low conductivity materials offer benefits in thermal insulation."
Culmination of Measurement Importance
Applications Across Different Industries
The impact of thermally conductive materials stretches far and wide across multiple sectors. Their role is more than just technical; it lays the groundwork for innovations that influence daily life. In fields ranging from electronics to aerospace, these materials are not merely supplements; they are essential stakeholders in improving efficiency and safety.
In this discussion, we will dive into the role and implications of thermally conductive materials in various industries, highlighting specific applications and benefits while touching on the considerations professionals must keep in mind.
Electronics and Thermal Management
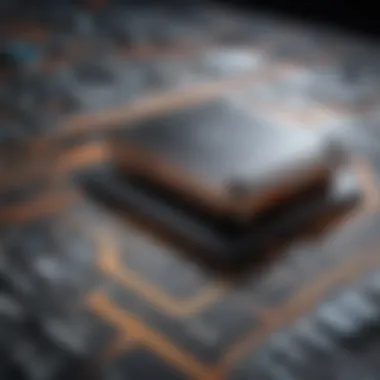
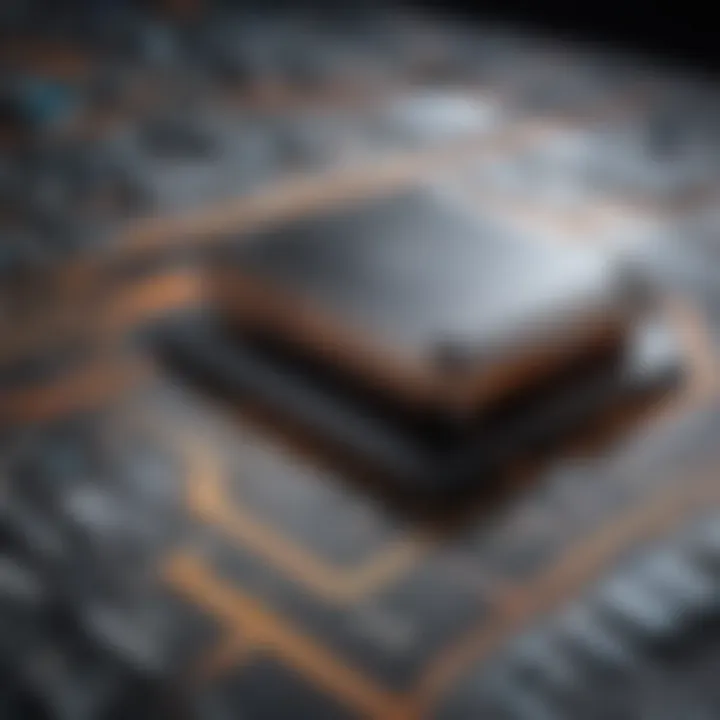
In the realm of electronics, effective thermal management is overwhelmingly paramount. As devices become smaller and more powerful, the heat generated increases, necessitating materials that can efficiently dissipate this heat. High thermal conductors, such as copper and aluminum, are commonly used for heat sinks and thermal pads. These materials pull heat away from critical components, ensuring systems operate within safe temperatures, reducing failures and extending component lifespan. In addition, innovative thermal interface materials, like thermally conductive adhesives, are emerging as game changers, providing both structural integrity and heat flow.
Key benefits in electronics include:
- Enhanced Performance: Reduced heating leads to improved function in processors and other critical components.
- Safety: Effective dissipation minimizes fire risks associated with overheating.
- Longevity: Devices last longer when thermal issues are managed well, reducing waste in the long run.
Balancing cost and performance, however, remains a challenge in this sector. Selectively using thermally conductive materials where most needed can be a smart approach, rather than blanket applications.
Building Materials and Energy Efficiency
The construction industry is another arena where thermally conductive materials hold significant promise. Incorporating these materials can enhance energy efficiency, an increasingly crucial factor as energy prices rise and sustainability takes center stage. For example, materials like aerogels offer excellent thermal insulation while allowing some degree of thermal conductivity, assuring buildings remain warm in winter and cool in summer.
- Heat Management: Smart materials can help regulate indoor temperatures, significantly reducing heating and cooling expenses.
- Sustainability: The use of energy-efficient materials aligns with current trends toward green building practices.
- Regulations Compliance: Many regions have strict building codes regarding energy efficiency, making thermally conductive solutions not just beneficial but necessary.
While the switch towards better thermal performance in buildings brings promising benefits, stakeholders often grapple with the initial costs of these materials and their integration into existing designs.
Automotive Applications
As cars evolve, so does the demand for advanced thermal management. Thermally conductive materials are playing a vital role in improving battery performance and cooling systems in electric and hybrid vehicles. Materials like silicone elastomers and specialized thermal compounds are engineered to facilitate heat transfer away from hot spots, thereby increasing overall efficiency and safety.
Considerations in the automotive world include:
- Battery Life Enhancement: Effective heat management can prolong battery life, which is essential for maintaining range in electric vehicles.
- Component Longevity: Appropriate material choices reduce wear on components by managing thermal stress, a major failure point in automotive systems.
- Regulatory Requirements: Meeting safety and performance standards is key in the automotive industry, making thermally conductive materials instrumental.
Moreover, auto manufacturers are continuously looking for innovative materials to maintain a competitive edge, driving research into next-gen solutions.
Aerospace Engineering
In aerospace, the stakes could not be higher. With the intensity of conditions, thermally conductive materials are integral to ensuring safety and performance. High-performance ceramics and composites are routinely used in parts exposed to extreme temperatures, like engine components and thermal shields.
Points of note in aerospace applications include:
- Weight Reduction: Efficient thermal management often leads to lighter materials, a critical consideration in aviation where every gram counts.
- High-Temperature Resistance: Materials must perform under extreme conditions without degrading, necessitating advanced development and testing.
- Reliability: The aerospace sector demands that every component operates flawlessly, making thermal stability in material selection a top priority.
In summary, the application of thermally conductive materials spans diverse industries, each benefiting uniquely from innovative solutions that enhance performance, energy efficiency, and safety. As the demand for these materials grows, it becomes evident that their role is not just supportive but critical to the evolution of modern technologies and structures.
Innovations in Thermally Conductive Materials
The landscape of thermally conductive materials is ever-evolving. With the demand for superior thermal management in various industries skyrocketing, innovations in this domain are paramount. These advancements not only enhance existing materials but also pave the way for entirely new applications, reshaping how industries approach thermal management challenges. In this section, we'll dive into some of the groundbreaking developments that promise to revolutionize thermal conductivity and explore why they are considered crucial in today’s technological environment.
Advanced Composite Materials
Advanced composite materials are a significant leap forward in enhancing thermal performance. These materials combine different components—each contributing unique characteristics to the final product. For instance, a common approach is to blend polymers with thermally conductive fillers like aluminum oxide or boron nitride.
This combination results in materials that are lightweight yet possess significant thermal conductivity, making them suitable for applications in electronics where both weight and heat dissipation are critical factors.
- Key Advantages:
- Enhanced thermal conductivity without a substantial increase in weight.
- Greater flexibility in design, allowing for tailored solutions across sectors, especially in automotive and aerospace.
- Increased durability and resistance to thermal fatigue.
Incorporating advanced composite materials into thermal management systems helps in creating more efficient devices and structures that can operate at higher temperatures without compromising performance.
Graphene and Its Implications
Graphene is often hailed as a revolutionary material in the world of thermally conductive materials. Its unique properties stem from its atomic structure, which consists of a single layer of carbon atoms arranged in a 2D honeycomb lattice. This pristine arrangement leads to exceptional thermal conductivity, surpassing most traditional materials.
The implications of using graphene are broad:
- Applications in Electronics:
- Potential in Energy Solutions:
- The lightweight nature and superior conductivity make graphene suitable for heat spreaders in electronic devices. Its ability to effectively dissipate heat can drastically improve performance, especially in high-power LED applications.
- Its conductivity can potentially enhance the efficiency of solar cells and improve thermal storage solutions.
- Graphene’s integration into thermal interface materials can shorten the heat transfer path, thus improving overall system efficiency.
"Graphene is more than a material; it’s a potential game changer in how we approach thermal management in our technologies."
3D Printing of Conductive Materials
The advent of 3D printing has opened up novel avenues for producing thermally conductive materials. With additive manufacturing, it's possible to fabricate complex geometries that were previously unimaginable using traditional manufacturing methods.
Materials for 3D printing can now be engineered to exhibit enhanced thermal properties, creating customized solutions for specific applications.
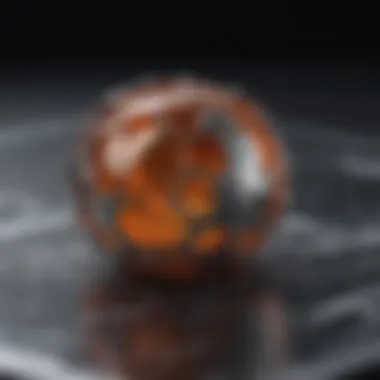
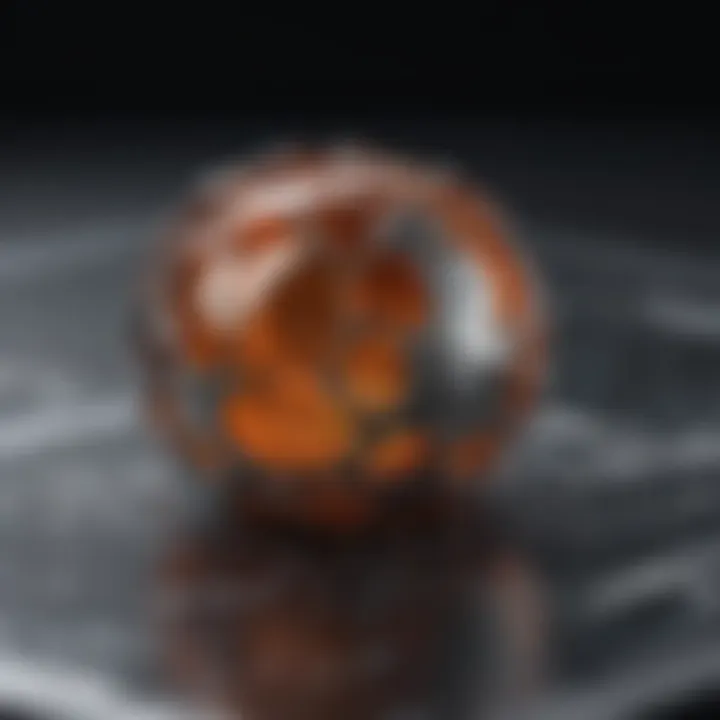
- Benefits of 3D Printing:
- Allows for the creation of intricate designs that optimize heat flow in targeted areas.
- Customizable thermal conductivity tailored for specific applications, ranging from consumer electronics to industrial settings.
- Reduces waste compared to traditional methods, as materials are added layer by layer.
The future of thermally conductive materials will likely see an increased integration of 3D printing technologies, enabling innovative designs that challenge the limitations of conventional manufacturing.
As industries continue to push the boundaries of thermal management, staying updated with these innovations is essential for any professional or researcher dedicated to advancing thermal conductivity applications in their respective fields.
Challenges in Thermally Conductive Material Development
When it comes to thermally conductive materials, understanding the difficulties in developing these substances is just as vital as knowing their applications and properties. These challenges have far-reaching implications, influencing not only advancements in material science but also impacting the practicalities of various industries. In a world that demands efficiency, especially in thermal management, recognizing these challenges can spark innovation and lead us toward better solutions.
Material Stability and Performance
The stability of thermally conductive materials over time is a serious concern. Many materials may exhibit excellent thermal conductivity initially but could degrade under thermal stress or environmental conditions. For instance, polymers often lose their conductive properties as they undergo thermal cycling, essentially a series of continual heating and cooling. This can be an Achilles' heel for applications where consistent performance is crucial, such as electronics cooling systems or aerospace components.
So, what’s the crux? Balancing thermal conductivity with material stability can be tricky. Researchers continuously explore composite materials, mixing different compounds to enhance thermal performance while ensuring robustness. This requires a keen understanding of the underlying mechanisms that could lead to material failure over time.
Important aspects to consider include:
- Thermal Cycling Resistance: Ability to withstand repeated temperature changes without losing functionality.
- Chemical Stability: Resistance to degradation when exposed to other substances.
- Mechanical Strength: Maintaining structural integrity under stress.
Utilizing advanced characterizations and simulations, scientists are trying to predict how materials will behave over time, aiming for options that won’t just perform well at the start but will also last long-term. The trade-offs between different properties need careful evaluation, as enhancing one aspect may come at the expense of another.
Cost and Scalability Issues
Cost and scalability represent another delicious slice of the pie, but what a tricky slice it is! Designing cutting-edge thermally conductive materials often involves expensive raw materials and specialized processes. For example, graphene emerges as a superstar in thermal conductivity but manufacturing it cost-effectively remains a significant hurdle. The high price could deter its widespread adoption in industries that operate with tight margins.
Additionally, scaling up production from lab-scale experiments to broad market applications introduces its own set of complications. The process may not only be cumbersome but can also inflate costs significantly. Companies hoping to offer thermally conductive solutions at competitive prices must navigate through a labryinth of engineering challenges. Some crucial points here are:
- Raw Material Availability: Sourcing quality materials at scale can be a roadblock.
- Manufacturing Techniques: Adapting the production methods to be more efficient without compromising quality is essential.
- Market Demand: Fluctuations in what industries need can complicate long-term planning.
The need for innovation here is clear. Researchers and manufacturers are focusing on finding cheaper alternatives or improving existing methods, such as developing new composite materials that balance performance and cost. While the hurdles appear substantial, they often stimulate creativity and forward-thinking solutions that can ultimately pave the way for revolutionary advancements in the field of thermally conductive materials.
"Navigating the path of material development means embracing the challenges as opportunities for innovation."
Future Directions in Research
The exploration of thermally conductive materials is entering a new chapter marked by rapid advancements and fresh perspectives. Future directions in research not only showcase the ever-evolving nature of this field but also emphasize the necessity for sustainable practices and innovative technologies. As industries strive to integrate more efficient materials, understanding these new trends becomes essential for both researchers and practitioners alike.
The Role of Artificial Intelligence in Material Analysis
Artificial Intelligence is making waves across various sectors, and material science is no exception. In the context of thermally conductive materials, AI can catalyze breakthroughs in both the design and analysis stages. Imagine employing machine learning algorithms to predict the thermal performance of a new composite before it's even synthesized. That's where the beauty lies.
One significant aspect of utilizing AI is its capacity for sifting through vast datasets. Conducting experiments on thermally conductive materials often involves extensive trial and error. With AI, researchers can analyze previous experiments and datasets, spotting patterns that might not be obvious to the human eye. For example, neural networks can identify the most efficient compositions for polymers or nanomaterials that deliver improved conductivity.
Moreover, AI can assist in optimizing production processes. By applying predictive analytics, industries can foretell the behavior of materials under different conditions. This information is crucial when developing novel thermally conductive materials that meet specific criteria, enhancing reliability without breaking the bank.
Sustainable and Eco-Friendly Solutions
As the world grapples with environmental challenges, the spotlight increasingly turns toward sustainability. This shift creates a pressing need for thermally conductive materials that not only perform well but also do so with minimal ecological impact. The future of material research must prioritize eco-friendly solutions.
Investing in sustainable sourcing of raw materials can significantly alter the landscape of thermal conductivity applications. For instance, biodegradable polymers are emerging as promising candidates. These materials, derived from organic compounds, may offer adequate thermal properties while reducing reliance on fossil fuels.
Additionally, professionals in the field need to consider recycling and material life-cycle assessments. Thermally conductive materials should not just be designed for efficiency, but also with an eye toward their end-of-life scenario. This means developing strategies for reusing or repurposing materials, thus crafting a circular economy around thermal management technologies.
"Sustainability isn’t just a trend; it’s a responsibility. Research must pave the way for thermally conductive materials that our planet can afford to utilize."
Concluding Thoughts
Thermally conductive materials are at the crux of multiple industries, driving innovation and efficiency in a variety of applications. Their significance stretches from fundamental scientific research to actionable solutions in everyday life, underlining how thermal conductivity plays a pivotal role in optimizing performance across various sectors.
Summary of Key Findings
In reviewing the landscape of thermal conductivity, several key points emerge. Firstly, the diversity in thermal conductive materials — from metals to advanced composites — illustrates the evolving nature of these substances. Each material type exhibits specific properties affecting their effective utilization in applications such as electronics, construction, and automotive engineering.
- Metals, like copper and aluminum, remain the benchmark for thermal performance, prized for their reliability and efficiency.
- Ceramics offer options that withstand extreme temperatures, making them indispensable in aerospace contexts.
- Polymers with enhanced thermal conductivity expand the horizons of material applications by providing lighter alternatives without compromising on performance.
- Nanomaterials like graphene introduce new potentials, enabling groundbreaking advances due to their superior thermal properties.
This broad understanding enables stakeholders to select the right material for specific applications, potentially leading to more energy-efficient designs and improved operational capabilities.
Implications for Future Research and Industry
The landscape of thermally conductive materials holds an exciting promise for future exploration. As industries lean towards sustainability, the drive for eco-friendly materials becomes imperative. Researchers are increasingly looking to natural composites or bio-inspired materials that maintain high thermal efficiency while minimizing environmental impact. The integration of artificial intelligence in material analysis can also streamline research processes, allowing for quicker identification of suitable candidates when developing new materials.
Furthermore, the potential of 3D printing in fabricating thermally conductive components may revolutionize how products are conceived and produced. This innovation grants designers the flexibility to create custom geometries, optimizing thermal management in unique applications.
As the demand for high-performance materials escalates with advancements in technology, maintaining a keen focus on thermal management, efficiency, and sustainability will shape the future dynamics of this field. The interplay of academic research and industrial application is bound to lead to innovative solutions, demonstrating that understanding thermal conductivity cannot be understated in the path towards smarter and eco-friendlier technology.