Exploring 16S Sequencing in Microbiome Research
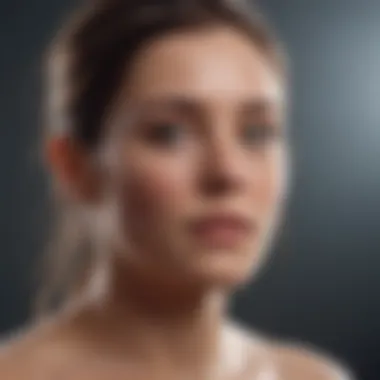
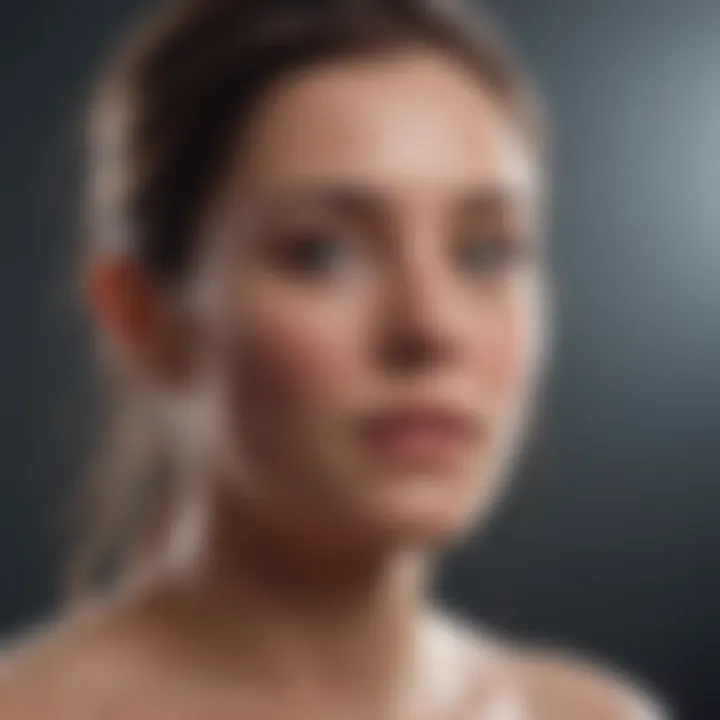
Intro
The study of microbiomes has gained significant attention in recent years. Microbiomes are complex communities of microorganisms that thrive in various environments, including the human body. Understanding these communities can provide insights into health, disease, and ecological balance. One of the pivotal techniques for microbiome research is 16S rRNA sequencing, a method that allows scientists to identify and characterize microbial species based on genetic information.
This article aims to delve into the nuances of 16S rRNA sequencing, exploring its methodologies, applications, and implications for various fields. The discussion will address both the technical aspects and practical applications of sequencing, aiding in the comprehension of microbial diversity and functionality.
Research Overview
Summary of Key Findings
16S rRNA sequencing offers a robust framework for understanding complex microbial communities. Key findings in microbiome research indicate that bacteria play an essential role in human health, nutrition, and disease. Particularly in digestive health, studies have shown that the composition of gut microbiota can impact metabolic processes and immune responses. The significance of this method lies in its ability to reveal the taxonomic diversity and functional potentials of these microbial communities.
Importance of the Research
The relevance of researching microbiomes extends across various disciplines, including health and environmental science. In clinical settings, pinpointing the microbial dysbiosis—an imbalance of microbial populations—can inform treatment strategies for conditions like obesity, diabetes, and autoimmune diseases. In environmental studies, 16S rRNA sequencing helps assess ecosystem health by tracking changes in microbial diversity in response to pollutants or climate change. This breadth of application highlights the need for continued innovation and understanding of 16S rRNA sequencing.
Methodology
Study Design
A typical study using 16S rRNA sequencing begins with the selection of samples, which may be derived from human subjects, soil, or water, depending on the research goals. The study design is often observational, aiming to correlate microbial characteristics with health outcomes or environmental factors. Random sampling is a common practice to ensure the representativeness of collected data, leading to more reliable conclusions.
Data Collection Techniques
Data collection involves several critical steps:
- Sample Preparation: This includes DNA extraction from the collected microbial samples, ensuring that the resultant DNA is of sufficient quality and quantity for sequencing.
- PCR Amplification: Polymerase Chain Reaction (PCR) is used to amplify the 16S rRNA gene, allowing for sufficient material to analyze the different microbial taxa present.
- Sequencing: Various platforms may be employed, such as Illumina or PacBio, each offering unique advantages in terms of read length and accuracy.
- Bioinformatics Analysis: Once the sequencing is completed, robust bioinformatics tools will analyze the data. Tools like QIIME or Mothur help classify and characterize the microorganisms based on their genetic sequences. This step is vital for synthesizing the information collected and for drawing meaningful conclusions from the data.
"The integration of advanced sequencing technologies with comprehensive bioinformatics tools enables researchers to paint a clearer picture of microbial ecosystems."
Through these methodologies, 16S rRNA sequencing continues to advance our understanding of microbial communities, setting a foundation for future exploration and application of microbiome research.
Prelude to 16S Sequencing
16S sequencing is a fundamental technique in the study of microbiomes, providing insights into microbial diversity and functionality. Its ability to profile complex microbial communities has made it essential in microbiology and biotechnology. Understanding the core principles of 16S rRNA sequencing helps researchers access myriad applications ranging from human health to environmental studies.
Overview of 16S rRNA
16S rRNA is a component of the ribosomal RNA that is universally present across all bacteria. This gene is particularly valuable due to its slow evolutionary rate, which makes it suitable for elucidating phylogenetic relationships among bacteria. In microbiome research, scientists leverage 16S rRNA for taxonomic identification. By amplifying and sequencing this specific gene, detailed catalogs of microbial communities can be created. These catalogs serve as a foundation for understanding how different microbial species interact with each other and their environments.
The primer selection for 16S rRNA is crucial, targeting conserved regions across different bacterial taxa. This specificity increases the sensitivity and resolution of the sequencing results. Given its conserved nature, 16S rRNA allows for the amplification of a wide variety of bacterial species, making it an indispensable tool in microbial ecology.
Historical Context and Development
The advent of 16S sequencing can be traced back to the early 1980s, when researchers first acknowledged the importance of ribosomal RNA in phylogenetics. Carl Woese pioneered the use of 16S rRNA for bacterial classification, laying the groundwork for modern microbiome research. The method has evolved over decades, transitioning from Sanger sequencing to next-generation sequencing technologies, which facilitate high-throughput data collection.
Initially, 16S sequencing was limited by available technologies and analytical methods. As sequencing platforms such as Illumina and PacBio emerged, the ability to analyze vast amounts of microbial DNA became feasible. This shift significantly enhanced researchers' understanding of microbial diversity in various environments, from human gut microbiomes to complex ecosystems. Today, 16S sequencing is a standard procedure in microbiome studies, continuously adapting to advancements in science and technology.
"16S rRNA gene sequencing has revolutionized our understanding of microbial communities, enabling studies that were once thought impossible."
The Technical Framework of 16S Sequencing
The technical framework of 16S sequencing is foundational in microbiome research. It encompasses crucial methodologies that facilitate the analysis of microbial communities. Understanding this framework allows researchers to ensure the accuracy and reliability of their findings. The technical details have direct implications for data quality and interpretation.
Sample Collection and Preparation
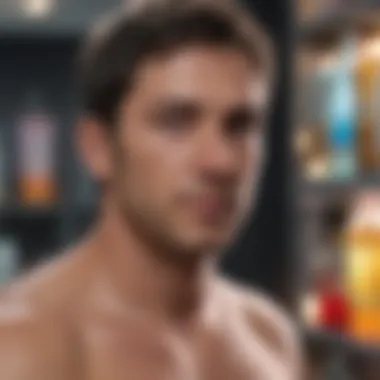
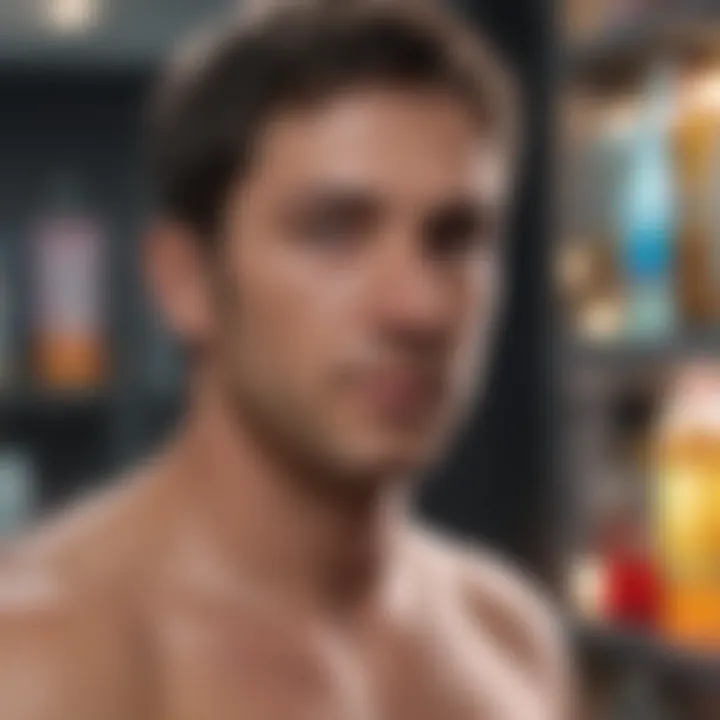
Types of samples
The types of samples collected are critical for 16S sequencing studies. Common choices include stool samples, saliva, skin swabs, and environmental samples. Each type provides unique insights into microbial diversity. Stool samples, for instance, are widely used for gut microbiome studies due to their richness in microbial DNA.
Utilizing these samples helps researchers gather data that are representative of the microbial community in question. It is essential to choose appropriate samples that reflect the area of study. However, each sample type has its limitations. For example, stool samples may not account for all microbial members present in gastrointestinal environments.
Preservation methods
Preservation methods play a significant role in maintaining sample integrity. These methods include refrigeration, freezing, and the use of preservatives such as RNAlater. One key characteristic of these methods is their ability to inhibit microbial degradation. Proper preservation ensures that DNA remains stable until analysis occurs.
Using appropriate preservation techniques enhances the reliability of sequencing results. Yet, it is essential to consider that some methods may alter the microbial community structure. For instance, freezing could lead to cell lysis, affecting the final analysis.
PCR Amplification Techniques
Primer selection
Primer selection is crucial in PCR amplification for 16S sequencing. Primers need to be specific to the 16S rRNA gene to ensure accurate amplification of the target regions. A key characteristic of effective primers is their uniqueness to microbial groups of interest. This specificity supports successful downstream analysis of microbial diversity.
Choosing the right primers can directly influence the results. Poor primer design can lead to biases in sequencing outputs. This can misrepresent the actual microbial community present.
Amplification challenges
Amplification challenges arise during PCR procedures. These challenges include nonspecific binding, which results in unwanted products. Efficient amplification is necessary for generating sufficient DNA for sequencing. One key characteristic of these challenges is the variability in amplification efficiency among different microbial taxa.
This phenomenon can lead to underrepresentation or overrepresentation of certain species. Thus, addressing these amplification challenges is critical for accurate microbiome profile representation in 16S sequencing.
Sequencing Platforms and Technologies
Overview of sequencing technologies
The overview of sequencing technologies reveals the diversity of platforms used in 16S sequencing. Technologies include Sanger sequencing, Illumina sequencing, and Oxford Nanopore. Each technology has distinct features that serve different research needs. Sanger sequencing is often favored for its accuracy but is less efficient for large-scale studies. In contrast, Illumina sequencing offers high throughput, suitable for expansive microbiome analyses.
The choice of technology can impact the quality and depth of the sequencing data obtained. Knowing the strengths and weaknesses of each platform is essential for researchers.
Comparison of platforms
Comparing sequencing platforms sheds light on their respective advantages and disadvantages. Illumina sequencing, for instance, is cost-effective for large datasets but may have issues with read length, making it challenging for resolving closely related species.
On the other hand, Oxford Nanopore provides long-read capabilities but can have higher error rates. Understanding these differences allows researchers to select the most appropriate platform for their study objectives, promoting reliable and interpretable results.
Data Analysis in 16S Sequencing
Data analysis is a fundamental aspect of 16S sequencing that translates raw sequence data into meaningful biological interpretations. This process is not merely about obtaining results; it embodies a complex interplay of bioinformatics, statistical evaluation, and interpretation of microbial ecology. As microbiome research expands, robust data analysis becomes crucial for understanding microbial communities and their roles in various ecosystems, including human health and the environment.
Bioinformatics Pipelines
Data preprocessing
Data preprocessing refers to the initial step of preparing raw sequencing data for subsequent analysis. This phase is vital for ensuring the quality and reliability of the data. Poor-quality reads can lead to erroneous conclusions, making preprocessing a necessary focus. Key characteristics of data preprocessing include filtering out low-quality sequences, trimming off adapter sequences, and removing chimeras. These steps are beneficial because they enhance the clarity of the results by maintaining only high-quality data.
One unique feature of preprocessing is that it can significantly affect the diversity outcomes in microbial studies. By reducing noise, researchers can more accurately represent microbial communities. However, it can also introduce biases if not performed carefully. For instance, overly aggressive filtering may remove legitimate sequences, leading to incomplete datasets. Therefore, researchers must strike a balance to ensure useful yet comprehensive datasets are produced.
OTU clustering
Operational Taxonomic Unit (OTU) clustering is a method used to classify similar sequences into groups, providing a means to analyze biodiversity. This process simplifies datasets by grouping reads that share common characteristics. The main advantage of OTU clustering is that it allows researchers to describe complex communities using a more manageable dataset, thus shedding light on overall composition and diversity.
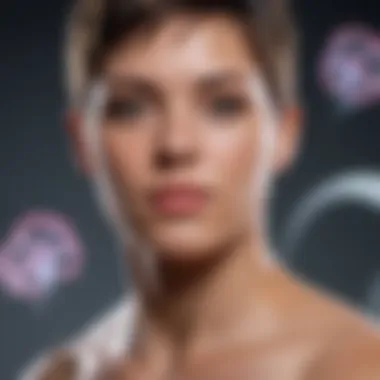
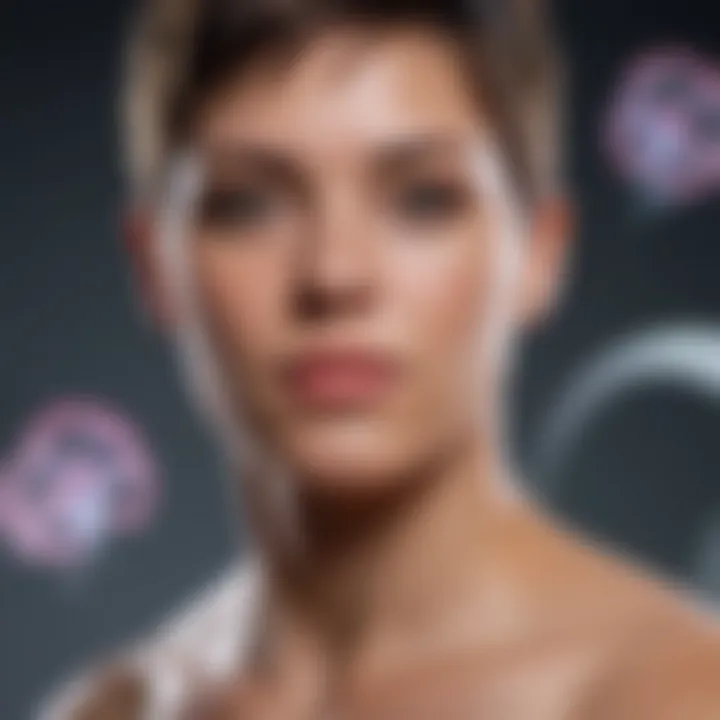
A unique aspect of OTU clustering is its reliance on a defined similarity threshold, often set at 97%. This threshold helps categorize sequences into OTUs effectively. However, this practice presents challenges as different clustering methods can produce various results. The choice of method could affect the resulting ecological significance attributed to different OTUs, leading to potential misinterpretations if the wrong method is applied.
Statistical Methods in Microbiome Studies
Diversity metrics
Diversity metrics quantify how many different types of organisms are present in a given sample and their relative abundances. These metrics are essential in comparing microbial richness and evenness across various environments or conditions, contributing significantly to our understanding of microbiomes. The most commonly used metrics include Shannon diversity index and Simpson's index, which focus on evenness and richness respectively.
The key characteristic of diversity metrics is their ability to summarize complex biological data into interpretable formats. They are beneficial in microbiome research as they can reveal shifts in community structures due to environmental changes or health status. However, a major disadvantage is their reliance on accurate taxonomic classifications. Poor classification accuracy may lead to misleading conclusions about community diversity.
Statistical tests
Statistical tests are essential for determining the significance of observed differences in microbial communities. These tests help validate hypotheses and identify specific factors or conditions influencing microbial composition. Common statistical tests used in microbiome studies include ANOVA, t-tests, and non-parametric tests such as Kruskal-Wallis.
The key characteristic of these statistical tests lies in their ability to differentiate between groups of data. This approach is particularly beneficial for understanding how different variables impact microbial communities. However, the interpretation of results must be done carefully; incorrect assumptions about data distribution or sample size can lead to invalid conclusions. Ensuring proper statistical rigor is therefore necessary in drawing credible insights from microbial analysis.
In microbiome studies, the integrity of the data analysis process contributes significantly toward the accuracy of ecological interpretations and practical applications.
Applications of 16S Sequencing
The applications of 16S sequencing are vast and varied, reflecting its integral role in advancing our understanding of microbiomes across different domains. Notably, this technique enables researchers to delve into both human health and environmental ecosystems, providing critical insights that were previously unattainable. These applications shed light on the complex interactions within microbial communities and their impacts on health and ecology.
Human Health and Disease
Microbiome and gut health
Gut health is increasingly recognized as a crucial aspect of overall well-being. The microbiome inhabiting our gastrointestinal tract plays a pivotal role in digestion, metabolism, and immune system function. The 16S rRNA sequencing allows for the characterization of this complex microbiota, revealing diversity and community structure. This method's key characteristic is its ability to identify and quantify specific bacterial populations. Such insights make it a valuable approach in understanding how microbiome composition affects human health.
By analyzing gut microbiome data, researchers can identify patterns relating to various health outcomes. For instance, a healthy gut microbiome is often associated with a higher diversity of beneficial bacteria, while dysbiosis—a microbial imbalance—has been linked with conditions like obesity, diabetes, and inflammatory diseases. The specific advantage of 16S sequencing is its non-invasive nature, making it suitable for studies involving dietary interventions or health status assessments. However, it does have limitations, such as its inability to identify functional capabilities of the microbiota or detect non-bacterial microorganisms.
Association with diseases
The associations between microbiota profiles and diseases have been the subject of extensive research. Understanding these relationships is essential in our fight against various chronic illnesses. The significant advantage of 16S sequencing lies in its ability to connect microbial communities with disease states. For example, shifts in microbiome composition can serve as biomarkers for diseases such as irritable bowel syndrome or certain cancers.
Key characteristic of this approach is the comprehensive overview it provides of microbial biodiversity in diseased states compared to healthy controls. However, the interpretation of these associations presents challenges. Correlation does not imply causation, and further studies are needed to establish whether these microbiome changes are a cause or an effect of disease. This creates an opportunity for subsequent follow-up studies that aim to confirm and elucidate the role of specific microbiota in various health conditions.
Environmental Microbiology
Microbial ecology
Microbial ecology is crucial for understanding how microorganisms interact with each other and their environment. This field explores diversity, distribution, and activities of microorganisms in various ecosystems. 16S sequencing has become a standard method for elucidating microbial communities in soil, water, and even extreme environments, such as hot springs or deep-sea vents. The technique's ability to provide detailed taxonomic information allows researchers to assess ecological relationships and adaptability among different microbial species.
The advantage of focusing on microbial ecology with 16S sequencing is the insight it provides into how microbial populations respond to environmental stressors. Understanding these dynamics can inform conservation efforts and ecosystem management. However, one limitation is the difficulty in correlating microbial data with ecological functions without combining it with functional sequencing approaches like metagenomics.
Pollution monitoring
Monitoring pollution levels and their effects on microbial communities is another crucial application of 16S sequencing. This technique can help identify shifts in microbial community structures in response to pollutants such as heavy metals or organic waste. The ease of conducting large-scale surveys facilitates the tracking of microbial responses to environmental changes over time.
A critical characteristic of using 16S sequencing for pollution monitoring is its capacity to provide early warning indicators of ecosystem changes. The disadvantage, however, is the need for extensive database comparisons to accurately assess changes in populations attributed to pollution versus natural fluctuations. As ecological and microbial dynamics are complex, these distinctions can sometimes be challenging.
In summary, the applications of 16S sequencing span across human health and environmental monitoring. By understanding the microbiome's role in our bodies and ecosystems, researchers can pave the way for innovative approaches in medicine and environmental science.
Challenges and Limitations of 16S Sequencing
Understanding the challenges and limitations of 16S sequencing is crucial for researchers and practitioners in microbiome studies. While this technique has significantly advanced our comprehension of microbial communities, several drawbacks must be acknowledged. Addressing these challenges is essential to improve the reliability and interpretability of data obtained from 16S sequencing.
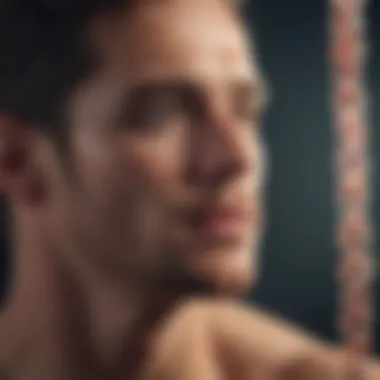
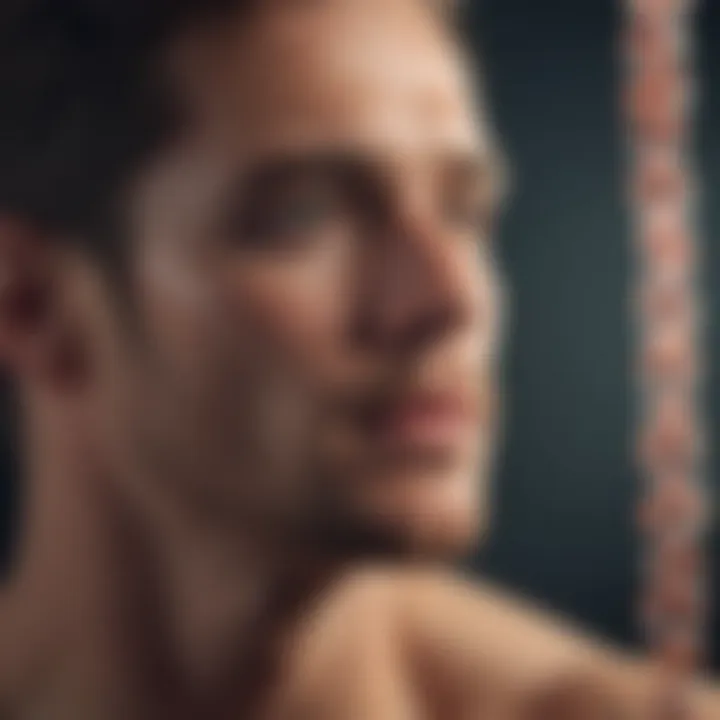
Technical Limitations
Bias introduced by PCR
PCR, or polymerase chain reaction, is a cornerstone technique in amplifying DNA for 16S sequencing. However, bias introduced by PCR can skew results. During amplification, some DNA fragments amplify better than others. This results in a disproportionate representation of certain taxa. Such bias may lead to misleading conclusions about microbial diversity.
One key characteristic of this bias is its dependent nature on primer specificity. Certain primers preferentially target specific sequences, which can result in overrepresentation of certain taxa while ignoring others. This aspect makes understanding the limitations of primer choice vital for researchers aiming for accurate representation of microbial communities.
While PCR bias is a common issue, it is not entirely negative. The amplification process is essential for detecting low-abundance microbes. However, the potential for skewed data must be carefully considered when interpreting results from 16S studies.
Resolution issues in taxonomic classification
Resolution issues often arise during taxonomic classification when using 16S sequencing. The fact is that 16S rRNA genes are conserved and share high similarity across various microbial taxa. This can pose challenges in accurately delineating closely related species.
One notable feature of these resolution issues is the difficulty in distinguishing between isolates at the species level. This is especially prevalent in highly diverse environments such as the gut. The inability to resolve such closely related taxa can lead to an incomplete understanding of microbial functionality and ecosystem dynamics.
The advantages of using 16S sequencing lie in its ability to provide a broad overview of microbial communities. However, researchers must be cautious about misinterpretations that stem from this resolution limitation. Thus, supplementary methods such as whole-genome sequencing may enhance taxonomic resolution but add further complexity to analysis.
Interpretative Challenges
Ecological significance of findings
Interpreting the ecological significance of findings derived from 16S sequencing is often fraught with difficulties. While this method successfully catalogs microbial diversity, linking these findings to ecological function remains challenging. Ecological significance often depends on contextual factors such as environment and interaction dynamics among microbes.
The contextual nature of ecological data makes it imperative to consider the broader ecological frameworks when interpreting results. This means researchers need to not only focus on microbial identity but also on environmental factors that shape their interactions.
Another aspect worth mentioning is the role of metabolic functions that these microbes perform, which are not always reflected in the 16S sequences. In many instances, the presence of a taxon does not straightforwardly imply a specific ecological function, creating a gap in understanding how microbial communities contribute to their ecosystem.
Limitations of correlation studies
Correlation studies provide valuable insights but also come with limitations. These studies often examine relationships between microbial communities and specific health or environmental outcomes. However, correlation does not equate to causation.
One major characteristic of these limitations is that confounding variables can heavily influence the observed relationships. For instance, multiple external factors may simultaneously impact microbial community composition and health outcomes, making it difficult to establish direct causal links.
Despite these challenges, correlational analyses can still guide further investigations. By highlighting association patterns, they can direct researchers toward potential causal mechanisms to explore further in controlled settings. Nonetheless, the interpretative limitations of correlation studies must always be kept in mind to avoid overgeneralization and misinterpretation of data.
Future Directions in 16S Sequencing and Microbiome Research
The future of 16S sequencing and microbiome research is a crucial area of study. As techniques evolve, so does our understanding of microbial ecosystems. The emphasis on advancing methodologies, while integrating new technologies, shows the path forward for the field. Researchers are keen to enhance accuracy and resolution in sequencing, which could significantly affect the interpretation of microbiome data.
Emerging Technologies and Innovations
Next-generation sequencing advancements
Next-generation sequencing (NGS) has transformed microbiome research by allowing for higher throughput and lower costs. This technology enables researchers to process multiple samples simultaneously, drastically speeding up data acquisition. A key characteristic of NGS is its ability to produce vast amounts of sequence data quickly, making it an efficient choice for large-scale studies. One unique feature of NGS is its reliance on massively parallel sequencing, allowing for detailed insights into microbial diversity at a scale previously unattainable. Despite its advantages, such as cost-effectiveness and speed, NGS can still introduce complexity in data interpretation.
Single-cell sequencing
Single-cell sequencing offers the ability to analyze the genetic material of individual cells. This technology provides insights into the heterogeneity of microbial populations, which is essential for understanding microbiome functionality. A notable characteristic of single-cell sequencing is its capacity to capture genomic information from rare or uncultured organisms. This makes it a valuable method in microbiome research, as many microbes are difficult to isolate in culture. However, the main disadvantage lies in its lower throughput compared to bulk sequencing methods, often making it more time-consuming and costly to analyze.
Integrating Multi-Omics Approaches
Metabolomics
Metabolomics plays a significant role in understanding the functional outputs of the microbiome. By analyzing metabolites produced by microbial communities, researchers can better interpret the biochemical interactions at play. A key aspect of metabolomics is its ability to link microbial activity with host health or disease status. This integration allows for a comprehensive view of how microbiomes influence physiological systems. However, metabolomics studies can face challenges in standardization and reproducibility, which may complicate conclusions drawn from diverse populations.
Proteomics
Proteomics involves the large-scale study of proteins and can provide valuable insights into microbial functions. Through this approach, researchers can identify active proteins in microbiomes under various conditions. The key characteristic of proteomics is its ability to reflect real-time biological activities, which is essential for understanding dynamic microbiome interactions. While proteomics offers advantages in terms of functional analysis, it can be technically challenging and expensive. This might limit its frequent use in microbiome research when compared to other approaches such as genomics or 16S sequencing.
As the field of microbiome research continues to advance, the integration of multiple omics approaches will likely provide richer insights into the complex interactions within microbial communities.