Exploring Innovations in Digital Polymerase Chain Reaction
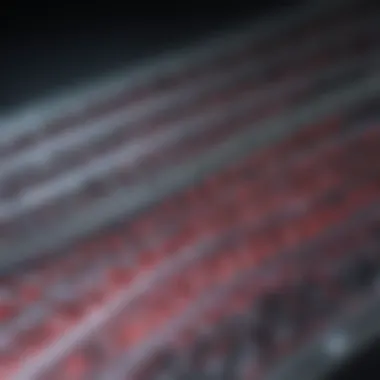
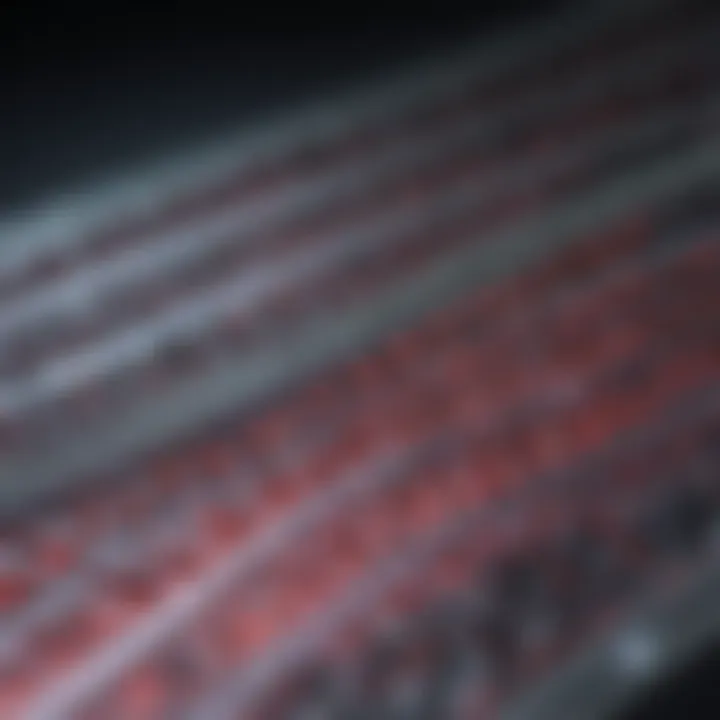
Intro
Digital polymerase chain reaction (dPCR) represents a critical evolution in the landscape of molecular biology. Traditional PCR has functioned as a cornerstone of genetic analysis for years. However, dPCR builds upon this foundation, enabling enhanced accuracy and quantification of nucleic acids. This makes it a topic worth exploring for researchers and professionals in the field.
In this article, we will cover key aspects of dPCR. From its methodology to its applications and limitations, we aim to provide a thorough understanding of this advanced technique. This discussion is particularly valuable for students, educators, and researchers aiming to expand their knowledge in molecular diagnostics and therapeutic development.
Research Overview
Summary of Key Findings
The exploration of dPCR reveals several significant findings:
- High Sensitivity: dPCR provides greater sensitivity in detecting low-abundance nucleic acids compared to traditional methods.
- Quantification Precision: This method achieves precise quantification without the need for standard curves.
- Reproducibility: The technology demonstrates high reproducibility across various platforms, making it suitable for clinical and research applications.
- Diverse Applications: From oncology to infectious disease diagnosis, dPCR is versatile and applicable in many fields.
These findings underscore how dPCR can dramatically enhance molecular biology research.
Importance of the Research
Understanding dPCR is essential for several reasons:
- Advancements in Diagnostics: dPCR can identify genetic mutations that are critical in cancer diagnostics.
- Enhanced Research Capabilities: The accuracy provided by dPCR fosters more reliable research outcomes.
- Therapeutic Innovations: Insights gained through dPCR can lead to more effective therapies in the medical field.
Thus, knowledge of dPCR is crucial for anyone involved in molecular biology.
Methodology
Study Design
The methodology of dPCR entails a refined approach to amplifying specific DNA sequences. This involves dividing the sample into numerous individual reactions, leading to partitioning of the target nucleic acids. Each partition undergoes PCR, allowing precise counting of the molecules that amplify. This design contrasts with traditional PCR, where the results can be affected by the initial template quantity.
Data Collection Techniques
Data collection in dPCR employs specialized instruments such as dedicated digital PCR systems. These machines capture fluorescence signals emitted during the amplification process. The key data collection techniques include:
- Droplet Digital PCR (ddPCR): This method uses water-oil emulsions to create thousands of droplets, each serving as an independent reaction chamber.
- Chip-based dPCR: This format uses microfluidic chips to facilitate reactions in a scalable manner.
Both techniques provide high-throughput capabilities and ensure that the quantification of nucleic acids is both accurate and efficient.
"Digital PCR represents a leap forward in molecular techniques, facilitating better insights into genetic material than ever before."
Finale
Digital polymerase chain reaction stands at the forefront of molecular biology advancements. The detailed focus on methodology, applications, and implications of dPCR exemplifies its potential as a transformative tool. This article sets the stage for a more in-depth examination of how dPCR is reshaping scientific perspectives across multiple disciplines.
Prolusion to Digital Polymerase Chain Reaction
Digital Polymerase Chain Reaction (dPCR) represents a significant advancement in the field of molecular biology, enhancing the capabilities of traditional PCR methodologies. This section emphasizes the critical role of dPCR in precise nucleic acid quantification. The importance of this topic is manifold, given that dPCR provides high sensitivity and reproducibility, leading to more reliable data for research and diagnostics. Furthermore, it allows researchers to explore rare genetic variations and to understand complex biological systems with greater precision.
The benefits of dPCR are especially relevant as they align with the increasing demand for accurate genomic analysis. The method's ability to precisely partition samples enables quantification even in samples with low concentrations of nucleic acids. Such capabilities are critical in various domains, including clinical diagnostics, environmental monitoring, and oncological research. In this article, we will delve into the multifaceted aspects of dPCR, from its underlying principles to its growing applications, while also examining its limitations and the future directions that research may take.
Definition and Overview
Digital PCR is a refined approach that adapts the traditional polymerase chain reaction principles to allow more quantitative results. At its core, dPCR is about partitioning a sample into thousands of individual reactions, where each partition can either amplify a target DNA molecule or not. This process results in a count of positive reactions that can be translated into the initial quantity of target nucleic acids in the sample.
In contrast to traditional PCR, which provides relative quantification and is sensitive to the variability in amplification efficiencies, dPCR focuses on absolute quantification. This allows for much greater accuracy in detecting variations in gene expression or copy number variations, crucial in precision medicine and genetic research.
Historical Context and Evolution
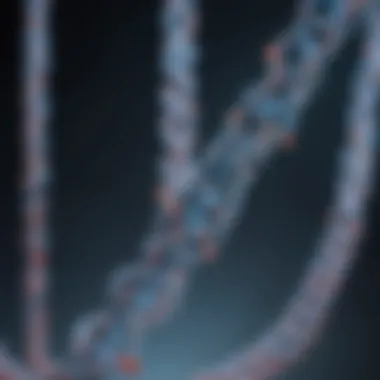
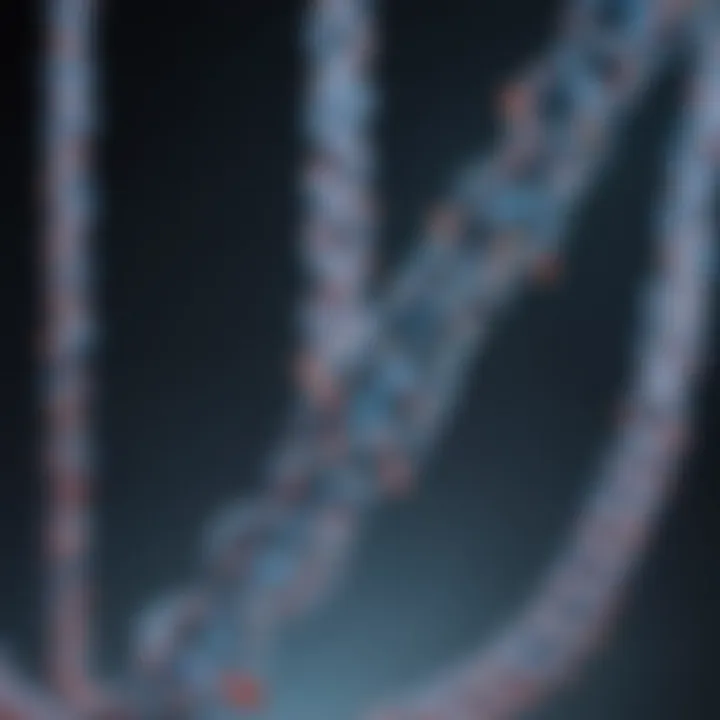
The roots of digital PCR can be traced back to developments in traditional PCR technology during the late 1980s, pioneered by Kary Mullis. However, the transition from the qualitative nature of PCR to the quantitative precision of dPCR began in earnest in the early 2000s. Researchers sought new ways to improve quantification, leading to innovations in microfluidics and droplet technology.
With the advent of digital systems, the method gained traction. By partitioning DNA into small volumes and amplifying in individual compartments, researchers were able to overcome significant challenges related to sensitivity and specificity. Notably, the introduction of microfluidic devices and digital droplet platforms by companies like Bio-Rad Laboratories allowed for the scalable application of dPCR, making it more accessible for researchers worldwide.
In summary, the evolution of digital PCR from traditional methods represents a significant leap forward in molecular biology. It addresses limitations of past techniques and opens new avenues for research and clinical applications, underscoring the need for continued investigation into its capabilities and implications.
Principles of Digital PCR
Understanding the principles of digital polymerase chain reaction (dPCR) is critical to appreciating its innovations and implications in the field of molecular biology. dPCR is not merely an extension of traditional PCR; it represents a fundamental shift in how nucleic acids are quantified and analyzed. This section delves into the core components and features of dPCR, illuminating its significance in research and clinical applications.
The Basic Mechanism
Digital PCR operates on a principle similar to that of traditional PCR but enhances the process of quantification. In digital PCR, the sample is partitioned into numerous smaller reactions, each containing either 0 or 1 copies of the target DNA sequence. These tiny reactions can take place in microfluidic chips or via droplet-based systems. By performing PCR on each individual partition, one can precisely measure the number of positive reactions, thus translating the results into an exact quantification of the target nucleic acid.
The process can be simplified into several key steps:
- Sample Partitioning: The initial sample is separated into thousands of individual compartments. This ensures that the target molecules are distributed evenly across the partitions.
- Amplification: PCR amplification occurs within each compartment. A positive outcome in a partition indicates the presence of the target sequence.
- Data Analysis: The results are analyzed to determine the concentration of the target sequence in the original sample based on the number of positive amplifications.
This discrete amplification process allows for extremely high sensitivity and precision, making dPCR a powerful tool in various applications.
Comparison with Traditional PCR
When comparing digital PCR and traditional PCR, several inherent differences and advantages become evident.
- Quantification: Traditional PCR provides a semiquantitative measurement through endpoint analysis or real-time monitoring, where the results are often influenced by the efficiency of the PCR process. In contrast, digital PCR offers absolute quantification, reducing variability.
- Sensitivity: Digital PCR demonstrates higher sensitivity, particularly when dealing with low-abundance targets. The partitioning technique helps overcome issues related to low target copies in the initial sample.
- Reproducibility: In digital PCR, the process is less affected by variations in PCR efficiency. The partitioning method standardizes reactions, enhancing reproducibility and reliability in results.
- Applications: While traditional PCR is suitable for broad applications, digital PCR finds its niche in areas requiring precise quantification, such as rare allele detection or viral load measurement.
"Digital PCR enables scientists to unlock insights and perform analyses that were previously unattainable with traditional PCR methodologies."
In sum, understanding these principles lays the groundwork for recognizing the value of digital PCR in advancing molecular diagnostics, genetic research, and other scientific fields. By leveraging the clarity and precision brought forth by dPCR, researchers can explore new frontiers in genomics and therapeutic developments.
Technical Advancements in Digital PCR
The advancement of digital polymerase chain reaction (dPCR) has led to significant technical innovations. Understanding these advances is crucial for grasping the full potential of dPCR in molecular biology. One of the primary benefits of these innovations is the increased precision in quantifying nucleic acids. This has a direct impact on various fields, particularly in clinical diagnostics, research, and biotechnology. The ability to conduct assays with high sensitivity and reproducibility enhances the reliability of results.
Microfluidics and Digital PCR
Microfluidics plays a pivotal role in the evolution of dPCR. This technology allows for the manipulation of small volumes of fluids at the micro-scale. In the context of dPCR, microfluidic systems enable highly efficient reactions by minimizing reagent consumption and enhancing control over PCR conditions.
The main advantages of using microfluidics in dPCR include:
- Reduced Costs: Less reagent use translates to lower costs for experiments.
- Increased Throughput: Microfluidics enable a greater number of reactions to be conducted simultaneously, which streamlines experiments.
- Enhanced Sensitivity: Smaller reaction volumes help in detecting rare nucleic acid targets more effectively.
Microfluidic devices also reduce the risk of contamination, which is often a concern in traditional PCR methods. As these systems evolve, their integration into routine laboratory practices will be crucial for improving the robustness of digital PCR assays.
Integration with Next-Generation Sequencing
The integration of dPCR with next-generation sequencing (NGS) ushers in a new realm of possibilities in genetic analysis. While NGS allows for the sequencing of millions of DNA fragments simultaneously, dPCR facilitates precise quantification of these fragments. The combined use of dPCR and NGS can significantly enhance the interpretation of genomic data.
Key benefits of this integration include:
- Accurate Quantification: Researchers can quantify specific mutations or gene expressions accurately alongside sequencing efforts.
- Enhanced Data Interpretation: The data produced from dPCR can guide the selection of sequences in NGS, allowing for a more focused analysis based on quantifiable insights.
- Streamlined Workflow: The complementary nature of these technologies can simplify workflows in genomics laboratories, improving efficiency.
As researchers continue to explore the synergies between dPCR and NGS, the breadth of applications in various fields is expanding. From oncology to genetic research, this integration promises to unearth insights that previously remained elusive.
"The convergence of dPCR and NGS represents a transformative shift in molecular diagnostics, driving advancements in personalized medicine and beyond."
Application Areas of Digital PCR
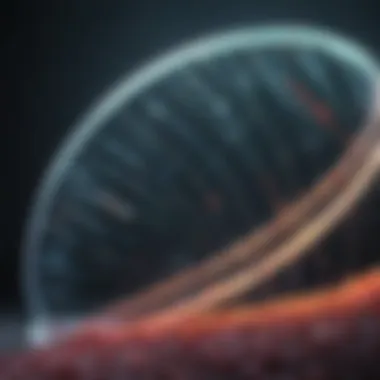
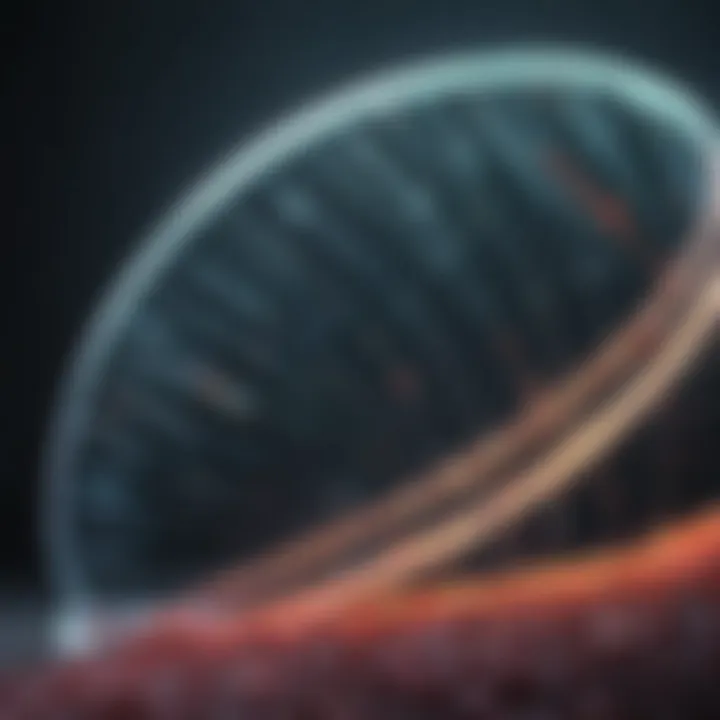
Digital polymerase chain reaction (dPCR) has rapidly evolved into a critical technique within molecular biology. Its unique capabilities offer significant advantages across various fields, impacting clinical diagnostics, environmental monitoring, agricultural biotechnology, and oncology research. Understanding these application areas allows for a better appreciation of dPCR's role in advancing science and improving outcomes in numerous sectors.
Clinical Diagnostics
In clinical diagnostics, digital PCR plays an important role due to its precision. This technique effectively quantifies viral load in patients with infections such as HIV or hepatitis. It provides a more accurate assessment compared to traditional PCR, as it allows for the detection of rare mutations or low-abundance targets in biomolecular samples. Furthermore, dPCR's capacity to deliver results with high reproducibility enhances its reliability in a clinical setting. This reliability is crucial for understanding disease progression and the effectiveness of treatment regimens.
Environmental Monitoring
Digital PCR is increasingly utilized in environmental monitoring, specifically for assessing water quality and detecting pathogens in various habitats. Its sensitivity allows for the detection of environmental DNA, including microorganisms at low concentrations. This is beneficial for monitoring ecosystems, as it can help in identifying bio-indicators of pollution or ecosystem health. In addition, dPCR aids in detecting genetically modified organisms in agricultural runoff, ensuring compliance with environmental standards.
Agricultural Biotechnology
In agricultural biotechnology, digital PCR helps in the precise quantification of genetically modified organisms in crops. This application is vital for regulatory compliance, as accurate measurements are necessary for safety assessments and environmental impact studies. Moreover, dPCR can assist researchers in evaluating plant traits and genetic markers associated with yield and disease resistance. Such innovations support the development of crops that thrive under challenging environmental conditions or are less susceptible to diseases.
Oncology and Genetic Research
Oncology and genetic research greatly benefit from the application of digital PCR. Researchers use dPCR to analyze circulating tumor DNA, allowing for the detection of specific mutations associated with different cancer types. This sensitivity is crucial for early cancer diagnosis and monitoring treatment responses. Additionally, dPCR aids in rare variant detection, providing insights into genetic diseases that may have previously gone unnoticed. Researchers can investigate genetic disorders with precision, contributing to advancing personalized medicine.
"Digital PCR has revolutionized the way we perceive molecular diagnostics, enabling detection and quantification at unprecedented levels of sensitivity."
In summary, the application areas of digital PCR are diverse and impactful. Its use in clinical diagnostics, environmental monitoring, agricultural biotechnology, and oncology highlights its versatility. This evolving technology not only improves accuracy and reliability in results but also paves the way for future advancements in multiple scientific domains.
Limitations and Challenges of Digital PCR
Digital polymerase chain reaction (dPCR) has made significant contributions to molecular biology. However, it is not without its limitations and challenges. Understanding these aspects is crucial for researchers, educators, and professionals utilizing this technology. Identifying potential weaknesses ensures a more informed application of dPCR methods in various research settings. It can also circumvent common pitfalls that may skew results or lead to incorrect conclusions, thereby enhancing the overall efficacy of studies relying on dPCR.
Technical Constraints
One notable limitation of digital PCR lies in its technical constraints. These constraints often arise from the complexity of the equipment required. Unlike traditional PCR, dPCR generally demands more advanced machinery that can isolate and quantify individual reactions. This may be a barrier for laboratories with limited resources.
Moreover, the need for precise temperature control and uniformity in sample distribution can add further technical challenges. Variations in primer efficiency and reaction conditions can lead to discrepancies in results. Therefore, achieving consistent outcomes can be difficult in practice.
Some key technical constraints include:
- Equipment Costs: High-quality dPCR systems can be expensive. This raises issues of affordability for small labs or institutions.
- Sample Preparation: The necessity for meticulous sample preparation can introduce variability that affects data integrity.
- Limited Multiplexing: Digital PCR often struggles with the simultaneous amplification of multiple targets due to its inherent design.
Recognizing these challenges allows researchers to strategically approach their experiments. Careful planning and understanding can alleviate some issues associated with technical constraints.
Interpretation of Results
The interpretation of results in digital PCR presents another challenge. While dPCR offers enhanced sensitivity and precision, translating these results into meaningful data remains complex. Researchers must interpret the results with critical attention to detail, considering the context of their experiments.
One significant aspect is the potential for false positives or negatives. This can occur due to contamination or errors in the sample handling. Furthermore, understanding the statistical analyses involved in dPCR data is crucial. Inadequate statistical methods can lead to misleading conclusions.
Key considerations in interpreting dPCR results include:
- Threshold Setting: Determining appropriate thresholds for positive results can be subjective and may require optimization.
- Data Normalization: Comparing dPCR data across different experimental conditions necessitates careful normalization to ensure accuracy.
- Assay Reproducibility: Variability between assays can complicate the interpretation process, necessitating multiple independent replicates.
Ultimately, the complexity of dPCR data interpretation highlights the need for robust training and experience among users. Being aware of these interpretative pitfalls can enhance the reliability of conclusions drawn from dPCR studies.
"The success of digital PCR hinges not only on its innovative capabilities but also on addressing inherent limitations that can impact results."
In summary, acknowledging the limitations and technical challenges of digital PCR enhances its application in research. A thorough understanding fosters more accurate results and ensures confident advancements in the field.
Comparative Effectiveness of Digital PCR
Digital polymerase chain reaction (dPCR) has emerged with notable advantages over traditional PCR techniques. A comprehensive understanding of its comparative effectiveness is vital for researchers and practitioners looking to make informed decisions in molecular biology. This section focuses on two key elements: sensitivity and specificity, and reproducibility in research. These aspects illustrate why dPCR is becoming a preferred choice in diverse fields.
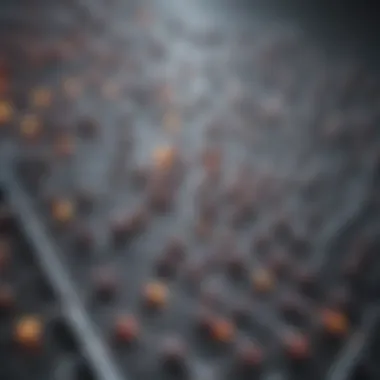
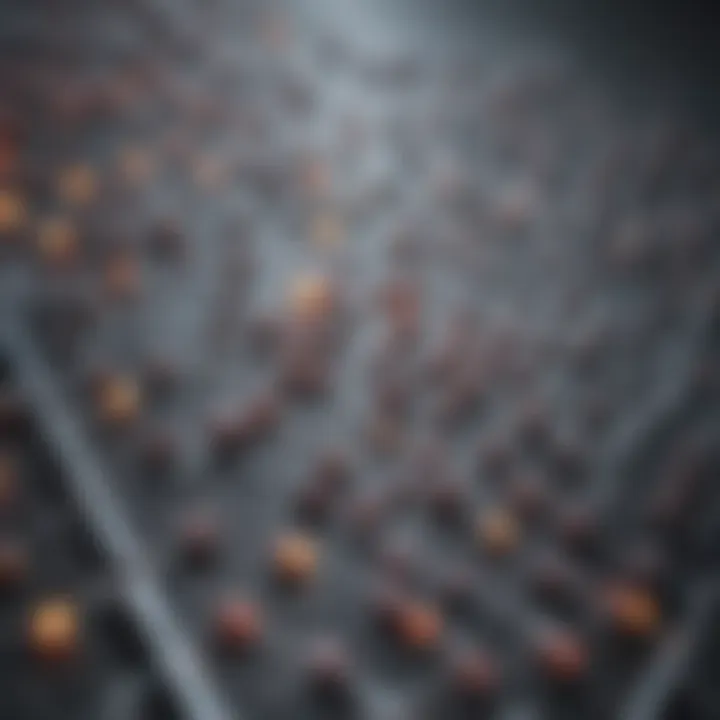
Sensitivity and Specificity
Sensitivity and specificity are critical parameters in assessing the effectiveness of any diagnostic tool. Sensitivity refers to a method's ability to correctly identify those with a condition, while specificity indicates its ability to correctly identify those without the condition. Digital PCR boasts heightened sensitivity, which allows for the detection of minute amounts of nucleic acids. This capability is particularly beneficial in clinical diagnostics and oncological research.
The precise quantification of nucleic acid molecules is fundamental in various applications. For instance, in detecting low-abundance mutations in cancerous tissues, dPCR can achieve detection limits significantly lower than traditional quantitative PCR methods. Furthermore, its digital nature minimizes the risk of contamination and provides more reliable results.
"Digital PCR's ability to detect rare genetic material can change diagnostic strategies, enabling earlier interventions in disease management."
In terms of specificity, digital PCR often shows superior performance due to its partitioning strategy. By compartmentalizing samples into numerous individual reactions, dPCR ensures that even if some compartments contain contaminating nucleic acids, they do not interfere with the overall analysis. This leads to fewer false positives and a clearer interpretation of results. The combination of high sensitivity and specificity offers researchers robust and trustworthy data.
Reproducibility in Research
Reproducibility is another pillar upon which the effectiveness of digital PCR stands. Scientific research relies heavily on the ability to replicate results across different experiments and institutions. The nature of dPCR facilitates this process, given its highly controlled conditions and digital readouts.
The precision in how reactions are conducted and analyzed allows laboratories to achieve consistent outcomes. This reliability is crucial, particularly in research settings where findings must be validated. Digital PCR provides a lower coefficient of variation compared to traditional methods, thus enhancing reproducibility.
Moreover, the robust software associated with digital PCR systems further aids in generating reproducible data. By standardizing analysis protocols and reducing manual errors, different research teams can arrive at similar conclusions based on the same data.
Future Directions in Digital PCR Research
The ongoing evolution of digital polymerase chain reaction (dPCR) signals a horizon rich with promise and potential. As the field of molecular biology advances, dPCR stands out due to its capacity for providing unprecedented accuracy and sensitivity in nucleic acid quantification. Understanding future directions in dPCR is crucial because it not only influences research but also impacts practical applications across various domains like clinical diagnostics, environmental monitoring, and agricultural biotechnology.
Research in dPCR aims to innovate technology that enhances overall performance. Future developments may address the efficiency of reactions, enabling researchers to achieve more in less time. Moreover, new methodologies could refine the quantification processes, making it possible to analyze even smaller samples with enhanced reliability. With these innovations on the horizon, the implications could be transformative.
Innovations on the Horizon
One notable direction for digital PCR is the integration of artificial intelligence and machine learning algorithms. These technologies can dramatically improve data analysis and result interpretation. By utilizing large datasets, AI can help in predicting outcomes and patterns that were previously unimaginable.
Further innovations may include improvements in microfluidic systems. Enhanced microfluidics could facilitate a reduction in reagent use and disposal costs, all while maintaining sensitivity and specificity. Additionally, developments in miniaturization could lead to portable dPCR devices ideal for on-field applications, making molecular diagnostics accessible beyond traditional laboratories.
Potential advancements also lie in increasing multiplexing capabilities. The ability to detect multiple targets in a single reaction would save time and resources. This would be particularly beneficial for applications in oncology where various biomarkers are often monitored together.
"The integration of AI with digital PCR technology represents a paradigm shift in how we can process and interpret large volumes of genetic data efficiently."
Expanding Accessibility in Research
To harness the full potential of dPCR, making the technology accessible to a broader range of researchers is essential. Currently, the high costs of dPCR systems can be prohibitive. Companies are now exploring more cost-effective alternatives that maintain quality, thereby democratizing access to this vital technology.
Training and resources are equally important to promote understanding and application. Collaboration between universities, research institutions, and industry can foster workshops and online courses. This will equip researchers with the necessary skills to use dPCR effectively.
Furthermore, enhancing open-access databases and sharing platforms could facilitate collaborative research efforts. Data generated from dPCR could be made more widely available for the scientific community, encouraging transparency and collaboration.
In summary, the future of digital PCR research not only promises significant technical advances but also seeks to broaden accessibility. The combined efforts in innovation and outreach will likely lead to a deeper integration of dPCR into mainstream research practices, ultimately enriching the landscape of molecular biology.
Closure: The Impact of Digital PCR
The integration of digital polymerase chain reaction (dPCR) into molecular biology represents a significant advancement with far-reaching implications. The unique attributes of dPCR, such as its ability to provide precise quantification of nucleic acids, have transformed various applications ranging from clinical diagnostics to research settings. In this conclusion, we will summarize key findings and offer insights into the future trajectory of molecular diagnostics.
Summary of Findings
Digital PCR is distinguished by its high sensitivity and superior accuracy compared to traditional PCR methods. This technology enables researchers to detect low-abundance targets in complex samples, making it indispensable in fields like oncology and infectious disease diagnostics. The primary benefits discussed include:
- Enhanced Sensitivity: dPCR's ability to detect rare genetic variants contributes significantly to the early diagnosis of diseases.
- Reproducibility: The digital nature of the technique provides consistent results, enhancing the reliability of data in scientific studies.
- Versatile Applications: From agricultural biotechnology to environmental monitoring, dPCR presents a wide array of use cases that extend beyond conventional boundaries.
Furthermore, the integration of microfluidics and automation with dPCR systems offers researchers the opportunity to reduce costs and improve efficiency without sacrificing performance.
Final Thoughts on the Future of Molecular Diagnostics
The future of molecular diagnostics is poised for transformative change, largely due to innovations in digital PCR. As technology continues to mature, we anticipate several key trends:
- Increased Accessibility: The ongoing development of user-friendly dPCR systems will make this technology available to a broader range of laboratories, including smaller research institutions.
- Advancements in Data Analysis: Enhanced bioinformatics tools will streamline the interpretation of dPCR results, aiding researchers in extracting meaningful insights from their data.
- Regulatory Approval and Clinical Adoption: As dPCR proves beneficial in clinical contexts, we expect it to gain traction in regulatory frameworks, ultimately leading to greater acceptance by healthcare professionals.
The impact of digital PCR on molecular diagnostics is both profound and inevitable. As it evolves, it will open new pathways for understanding complex biological systems and improving patient outcomes.
In summary, digital PCR is more than just an incremental improvement over traditional methods; it is a paradigm shift that paves the way for future advancements in molecular biology and diagnostics.