Sambrook's Molecular Cloning: Key Insights and Methods
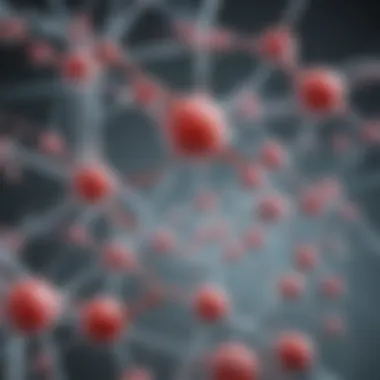
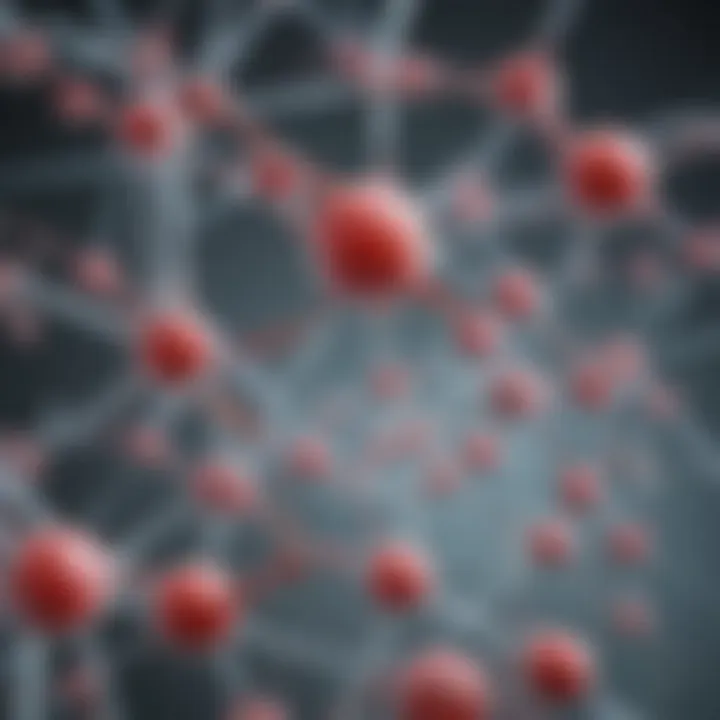
Intro
Molecular cloning serves as a cornerstone of modern biology, allowing scientists to manipulate DNA for various purposes. In this realm, Sambrook and Russell's manual stands out as a definitive guide. The manual meticulously details approaches and principals in cloning, evolving alongside advances in technology and research needs. Understanding this content is vital for students, researchers, educators, and professionals who seek to navigate the complexities of molecular biology.
Research Overview
Summary of Key Findings
Sambrook's work emphasizes the crucial components involved in molecular cloning. This includes the use of vectors, the role of restriction enzymes, and key methodologies. Notable findings suggest that the choice of vector significantly impacts the cloning efficiency and expression of the inserted gene. Moreover, the exploration of restriction enzymes reveals their indispensable role in DNA manipulation. Through various protocols, the manual illustrates essential steps for successful cloning.
Importance of the Research
The implications of molecular cloning extend far beyond the laboratory. Sambrook's manual not only provides practical insights but also showcases the profound impact cloning has on genetics, medicine, and biotechnology. Molecular cloning allows for the production of recombinant proteins, gene therapy, and development of genetically modified organisms. As a result, this work has inspired countless advancements across multiple disciplines.
Methodology
Study Design
The methodology outlined in Sambrook's manual incorporates both traditional and contemporary techniques. By offering a foundation in the principles, the manual allows users to adapt and innovate. Key techniques include transformation, ligation, and screening of transformed cells. Each step is methodically explained, making it easier for readers to comprehend complex procedures.
Data Collection Techniques
Essential data collection methods used in molecular cloning involve the application of various assays. Assessing cloning efficiency typically requires validating the presence of inserted DNA through techniques like PCR and gel electrophoresis. These methods allow researchers to collect reliable data on their experiments, ensuring that cloning processes yield the desired results.
"Molecular cloning not only expands our understanding of genetic material but also enables innovations in disease treatment and agricultural advances."
Epilogue
In summary, Sambrook's Molecular Cloning: A Laboratory Manual serves as an invaluable resource for anyone engaged in molecular biology. The combination of detailed methodologies, clear instructions, and practical applications solidify its position as a key text in the field. Becoming familiar with these principles allows researchers to contribute meaningfully to scientific understanding and address real-world challenges.
Preamble to Molecular Cloning
Molecular cloning stands as a cornerstone of modern biology, offering techniques that allow for the precise manipulation of genetic material. Understanding the principles underlying molecular cloning is crucial for a variety of fields, including genetics, microbiology, and biotechnology. By learning the methodologies and frameworks presented in works like Sambrook and Russell's Molecular Cloning: A Laboratory Manual, researchers and practitioners can gain insights into the reproducible strategies that define contemporary genetic experimentation.
Molecular cloning encompasses a broad spectrum of techniques that enable the isolation and replication of specific DNA fragments. This process contributes not only to basic scientific research but also to applied fields such as medicine and agriculture. The benefits of mastering molecular cloning are substantial. It facilitates gene identification, the construction of recombinant DNA molecules, and the subsequent expression of these molecules in host systems. Each of these steps is integral to understanding gene function and developing biotechnological applications.
Definition and Purpose
Molecular cloning can be defined as the process of creating multiple copies of a specific DNA segment by inserting it into a vector and introducing it into a host organism. This definition simplifies a highly intricate series of procedures that can include the digestion of DNA with restriction enzymes, ligation into a suitable vector, and transformation into competent cells. The purpose of these operations is manifold; primarily, it allows for the analysis of gene functions, the production of proteins, and the creation of DNA sequences for further study or use in various applications.
Through this defined process, researchers can gain valuable insights into genetic materials that may hold the keys to understanding disease mechanisms, developing new therapeutics, or engineering crops with enhanced traits. The versatility of molecular cloning lies in its ability to support a wide array of scientific inquiries, reinforcing its position as a pivotal technique in both academic and commercial laboratories.
Historical Background
The historical evolution of molecular cloning demonstrates its rapid advancement since its inception. Early techniques in recombinant DNA research emerged in the 1970s, primarily based on the discovery of restriction enzymes and the ability to manipulate DNA. These advancements were significantly influenced by pioneers such as Paul Berg, who is often credited with creating the first recombinant DNA molecules. By the 1980s, the techniques had matured, leading to the publication of protocols and standardized methods in the scientific literature.
The first edition of Sambrook and Russell's Molecular Cloning: A Laboratory Manual was published in 1989, marking a significant moment in the formalization of cloning protocols. This manual became a comprehensive resource for researchers, providing clear instructions and illustrations that greatly facilitated the learning process. Subsequent editions have introduced updates to protocols and included advances in technology, ensuring that the manual remains relevant to evolving scientific practices.
As the techniques of molecular cloning continue to develop, so too do the applications of its methods. From the Human Genome Project to CRISPR technology, molecular cloning has laid the groundwork for breakthroughs that extend far beyond the laboratory. The historical insights into the evolution of these techniques underscore the important role that foundational knowledge plays in shaping modern biological research.
Sambrook and Russell's Contribution
Sambrook and Russell's work has greatly shaped the landscape of molecular biology, particularly in the field of molecular cloning. The manual they produced is not just a guide; it is a vital resource that has facilitated numerous breakthroughs in genetics and biotechnology. With its comprehensive nature, it serves as a foundational text for anyone embarking on experimentation in molecular cloning. This text details protocols, techniques, and methodologies that have become standard procedures in laboratories worldwide. The contributions from Sambrook and Russell extend beyond mere instructions; they provide insight into the reasoning behind various techniques, thus educating readers on the principles driving molecular cloning.
The influence of their work can be seen across various applications, from academic research to commercial biotechnology. Their manual has supported advancements in gene therapy, recombinant DNA technology, and even the development of genetically modified organisms. This widespread impact illustrates the manual's significance in enhancing scientific knowledge and its application in diverse fields. The detail and clarity afforded by Sambrook and Russell's instructions help ensure that even those new to the field can navigate complex protocols successfully.
Overview of the Manual
The manual "Molecular Cloning: A Laboratory Manual" provides a meticulous examination of various techniques in the field. Initially published in the 1980s, it has since become a cornerstone of molecular biology practices. The book contains step-by-step protocols, which simplify complex processes like DNA extraction, amplification, and cloning. Readers will find that each protocol is accompanied by clear explanations detailing the purpose and importance of specific steps.
The structured organization of the manual allows users to easily follow along with experiments. It details all necessary equipment and reagents while addressing possible troubleshooting tips in case something goes wrong. Thus, the manual not only acts as a set of instructions but also as an educational tool offering a deeper understanding behind the processes involved in molecular cloning.
"The manual has guided countless scientists in their research, ensuring accuracy and reproducibility in molecular cloning experiments."
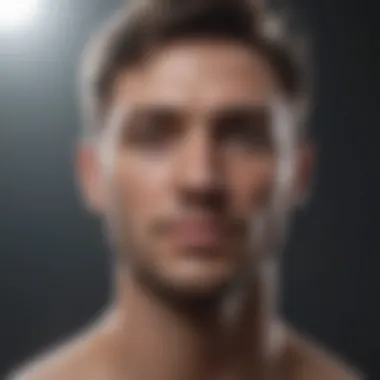
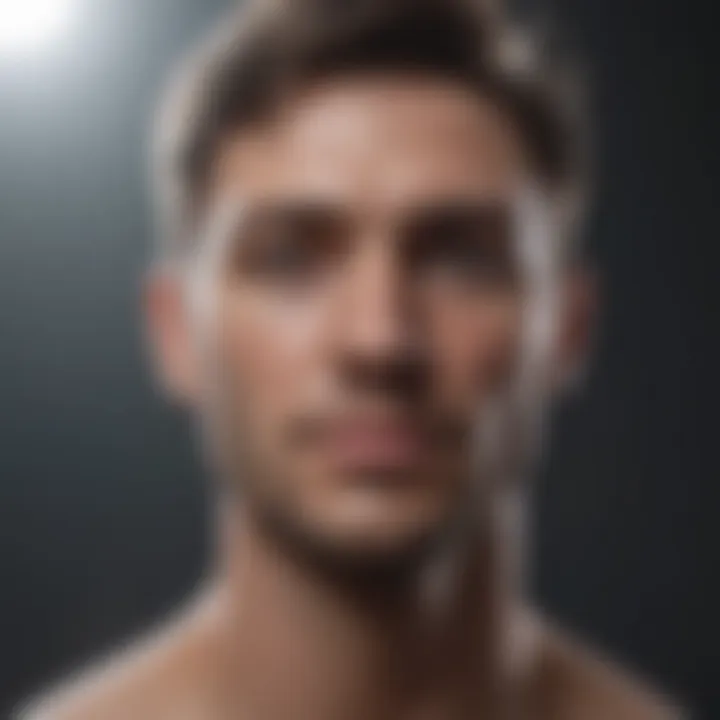
Key Editions and Updates
Over the years, Sambrook and Russell's manual has gone through several editions, each aimed at improving clarity and integrating new advancements in the field of molecular biology. The latest editions have incorporated changes reflecting the rapidly evolving landscape of genetic engineering and molecular techniques.
Key updates often include:
- New cloning strategies that have emerged from ongoing research.
- Insights into novel cloning vectors and their specific applications.
- Detailed descriptions of updated protocols to increase efficiency and success rates.
These revisions ensure that the manual remains relevant and useful for modern scientists. Each new edition reflects both the advancements made since the last and the collective experiences of scientists who have utilized the manual in their work. As a result, it provides an up-to-date repository of knowledge that continues to inform and educate.
Cloning Vectors
Cloning vectors are crucial tools in molecular cloning. They serve as vehicles that transfer genetic material into host cells. Selected properly, they facilitate the replication and expression of inserted DNA. Understanding cloning vectors enriches the cloning process. The type of vector chosen can impact yield, efficiency, and specificity of experiments. It is essential for any researcher to grasp these important elements to ensure successful cloning.
Types of Vectors
There are several types of cloning vectors, each with its unique features and applications. Some commonly used vectors include:
- Plasmids: Small, circular pieces of DNA that replicate independently within bacterial cells. They are widely used due to their ease of manipulation and efficient transformation processes.
- Bacteriophages: Viruses that infect bacteria. They can carry larger DNA inserts compared to plasmids and are useful for constructing genomic libraries.
- Cosmids: Hybrid vectors that combine features of plasmids and bacteriophage lambda. They can hold larger DNA fragments than typical plasmids, which increases the versatility in various applications.
- Artificial Chromosomes: Such as yeast artificial chromosomes (YACs) and bacterial artificial chromosomes (BACs), these vectors permit the cloning of very large DNA fragments, making them suitable for genomics.
Each type of vector has its advantages and potential limitations. For instance, plasmids are generally easy to work with, while YACs allow insertion of longer DNA sequences, which can be critical for complex genomic work.
Selection of Appropriate Vectors
Selecting the right vector is a decisive step in the molecular cloning process. Key considerations include:
- Size of insert: The size of the DNA fragment to be cloned can greatly influence the choice of vector. For larger inserts, BACs or YACs may be necessary.
- Host organism: It is vital to choose a vector that is compatible with the host organism. Different vectors are tailored for use in distinct hosts. For example, certain plasmids may only function in E. coli.
- Vector features: Specific characteristics, such as the presence of selectable markers and replication origins, should match the goals of the experiment. This can help in identifying successful clones after transformation.
- Purpose of cloning: If the goal is to generate a protein or study gene expression, vectors with appropriate promoters and regulatory sequences should be selected.
The choice of vector ultimately affects the overall efficiency and outcome of the cloning project. Therefore, careful deliberation is necessary to align vector selection with experimental objectives.
"The right vector is essential for the success of any molecular cloning experiment, influencing factors from reproducibility to the clarity of results."
Understanding cloning vectors aids in advancing genetic research and developing new biotechnological applications. Incorporating well-chosen vectors enhances the capabilities of scientists to manipulate and explore genetic material.
Restriction Enzymes
Restriction enzymes, also known as restriction endonucleases, represent a crucial element in molecular cloning. They facilitate the manipulation of DNA by cutting it at specific sequences, thus allowing the insertion, deletion, or modification of genetic material. This ability to cut DNA precisely is fundamental for cloning processes, as it enables researchers to create recombinant DNA and expand our understanding of genetics.
The importance of restriction enzymes cannot be overstated. By allowing targeted cuts, they make it possible to isolate specific gene fragments necessary for research and application in biotechnology. Moreover, their precision reduces the chances of unwanted mutations, which is critical for ensuring the integrity of the cloned DNA.
Function and Mechanism
Restriction enzymes function by recognizing specific nucleotide sequences within a DNA molecule, which are often palindromic. Once these sequences are detected, the enzyme cleaves the DNA at these points. The mechanism typically involves three key steps:
- Recognition: The enzyme scans the DNA until it finds its specific target sequence.
- Binding: After recognition, the enzyme binds to the DNA to form a stable complex.
- Cleavage: Finally, the enzyme catalyzes the breaking of phosphodiester bonds in the DNA backbone, resulting in DNA fragments.
The specificity of these enzymes to their target sequences means that they can be utilized in various applications, including cloning, genetic mapping, and mutagenesis.
Commonly Used Restriction Enzymes
There are several widely used restriction enzymes, each with unique recognition sites and cutting patterns. Some key enzymes include:
- EcoRI: Recognizes the sequence GAATTC and cuts between G and A. It is one of the most common enzymes used in cloning.
- HindIII: Recognizes AAGCTT and cuts between A and A. It is known for generating cohesive ends that facilitate ligation.
- BamHI: Cuts at the site GGATCC, also generating sticky ends that can be used for cloning.
- NotI: Recognizes the larger sequence GCGGCCGC, advantageous for larger DNA constructs.
These enzymes each contribute uniquely to various cloning strategies, highlighting the versatile application of restriction enzymes in molecular cloning processes.
"Restriction enzymes are indispensable tools in molecular biology, enabling precise cutting and pasting of genetic material for diverse applications."
In summary, restriction enzymes are fundamental in molecular cloning as they provide the means to manipulate DNA with precision. Understanding their function and the application of commonly used enzymes enhances the overall comprehension of molecular cloning techniques.
DNA Ligation Techniques
DNA ligation is a critical step in the process of molecular cloning. This technique is essential for combining DNA fragments, allowing scientists to create recombinant DNA molecules. Understanding the nuances of DNA ligation techniques is important because they directly impact the efficiency and effectiveness of cloning experiments. By mastering these methods, researchers can ensure the successful manipulation of genetic material for various applications.
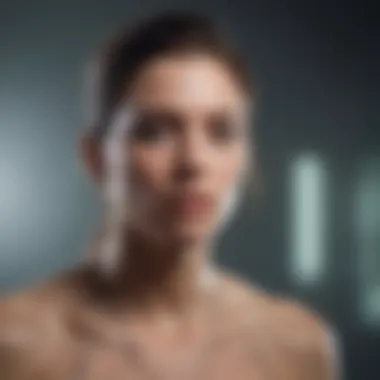
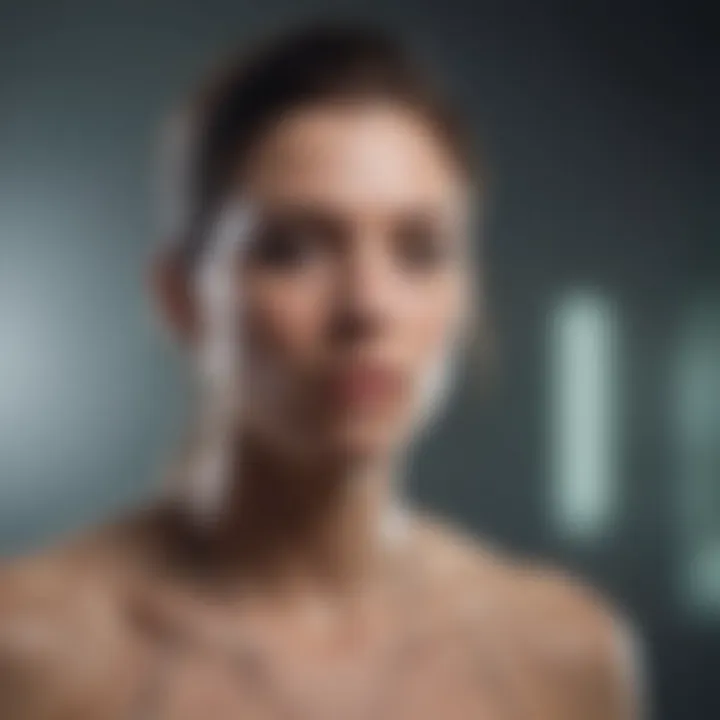
Ligase Enzymes
Ligase enzymes are the key players in the DNA ligation process. Their primary function is to join the phosphodiester backbone of two DNA strands. Among the commonly used ligases, T4 DNA Ligase is prevalent in laboratory settings. It requires ATP as a cofactor and is known for its versatility in ligating blunt and sticky ends. This adaptability allows for greater flexibility in experimental designs. Another example is E. coli DNA Ligase, which is useful for specific applications involving bacterial systems.
Ligases operate under particular conditions. Temperature, pH, and ionic strength all influence their activity. Careful optimization is critical. In appropriate conditions, ligases can catalyze the formation of covalent bonds efficiently.
Methods of Ligation
Several methods exist for performing DNA ligation, each with unique considerations. The choice of method often depends on the specific requirements of the cloning project.
- Standard Ligation: This method involves mixing the DNA fragments with a ligase enzyme and allowing the reaction to proceed at room temperature or at a temperature conducive to ligase activity. The classic T4 ligation protocol falls into this category.
- Quick Ligation: This technique utilizes a variant of T4 DNA ligase that operates at elevated temperatures, enabling faster reactions. This can greatly reduce the time required for cloning experiments, making it a favored option for high-throughput settings.
- In Vivo Ligation: In certain cases, ligation can occur inside the host organism. This method takes advantage of the natural cellular machinery for DNA repair. By introducing linearized plasmids into competent cells, the cell's endogenous ligation processes can facilitate the joining of DNA fragments.
These methods collectively offer various approaches to DNA ligation, allowing researchers to select the most suitable one to align with their experimental goals. By knowing these methods well, scientists can streamline their cloning workflows and enhance the overall yield of recombinant DNA.
Transformation Methods
Transformation is a crucial step in molecular cloning, enabling the introduction of foreign DNA into host cells. This process is essential for genetic manipulation, allowing scientists to explore gene functions and produce notable proteins or other products. Understanding transformation methods not only speaks to the technical prowess required in molecular biology but also highlights the innovation in genetic engineering practices.
Two prominent methods are commonly employed: electroporation and heat shock. Each of these techniques has its peculiarities and advantages, setting the stage for manipulating genetic materials across various applications.
Electroporation and Heat Shock
Electroporation utilizes an electrical field to increase the permeability of the cell membrane, thereby facilitating DNA uptake. Cells are suspended in a medium containing DNA, and a quick electrical pulse is applied. This creates temporary pores in the membrane, allowing the DNA to enter.
Benefits of electroporation include:
- High Efficiency: It can achieve significant transformation efficiencies, particularly in bacterial and some mammalian cells.
- Versatility: Suitable for various cell types, including those difficult to transform via other methods.
- Scalability: Can be applied in both small and large-scale systems, making it ideal for industrial applications.
However, considerations must be made. Electroporation can damage cells if parameters such as voltage and pulse duration are not optimized, leading to cell death and thus decreasing yield.
On the other hand, heat shock is a more traditional technique, especially in the transformation of E. coli. This method involves incubating cells at a high temperature, usually around 42°C, followed by a rapid return to lower temperatures. The sudden temperature shift encourages DNA uptake by forcing the cell membrane to become temporarily more permeable.
Key characteristics of heat shock include:
- Simplicity: The procedure is straightforward and requires minimal specialized equipment.
- Cost-Effectiveness: Particularly beneficial for routine transformations in laboratories focused on bacterial cloning.
- Reproducibility: It offers consistent results for E. coli transformation, making it a go-to method in many settings.
Nonetheless, the efficiency of heat shock can be lower than electroporation for some plasmids, indicating a potential limitation in more complex cloning tasks.
Natural Transformation Mechanisms
Natural transformation mechanisms refer to the process through which certain bacteria can uptake naked DNA from their surroundings without the need for artificial methods. This phenomenon is particularly fascinating since it occurs naturally in some species, such as Streptococcus pneumoniae and Bacillus subtilis. Understanding these mechanisms can provide insight into bacterial evolution and gene exchange within populations.
Considerations around natural transformation include:
- Genetic Diversity: It plays a key role in aiding genetic variation among bacterial populations, impacting traits such as antibiotic resistance.
- Study of Pathogenicity: Understanding how pathogens acquire virulence factors can lead to insights into disease mechanisms and potential treatments.
- Applications in Biotechnology: Harnessing natural competence can facilitate gene editing and synthetic biology endeavors, promoting innovations in genetic manipulation.
In summary, the methods of transformation—specifically electroporation, heat shock, and natural mechanisms—play an integral role in molecular cloning. Each method presents unique advantages and considerations that can influence cloning success and efficiency. This knowledge is vital for researchers aiming to utilize molecular cloning in their studies, pushing the boundaries of genetic engineering and biotechnological advancements.
Screening and Selection of Clones
The screening and selection of clones is a pivotal step in molecular cloning, directly impacting the success of the overall project. This process ensures that only clones with the desired insert are propagated, eliminating those without the insert or with unwanted sequences. The importance of effective screening cannot be understated, as it lays the foundation for accurate analysis in subsequent stages of research.
When working with transformation methods, a multitude of transformed cells is generated. Among these, only a subset will successfully incorporate the foreign DNA segment. Thus, implementing robust screening techniques is crucial to identify and select those cells that maintain the desired genetic construct.
Antibiotic Resistance Markers
Antibiotic resistance markers serve as powerful tools for selecting transformed cells in molecular cloning experiments. Typically, bacterial vectors such as plasmids include an antibiotic resistance gene. This gene provides a clear means to distinguish between transformed and non-transformed cells. When a culture containing the plasmid is exposed to the corresponding antibiotic, only those cells that have successfully taken up the plasmid will survive.
Common antibiotics used in selection include:
- Ampicillin: Often used to select for plasmids containing the bla gene, which encodes beta-lactamase.
- Kanamycin: Selected with plasmids featuring the kan gene, allowing survival of cells that have gained kanamycin resistance.
- Tetracycline: Utilized for cells containing the tet gene, protecting them from tetracycline's inhibitory effects.
Selecting for antibiotic resistance not only streamlines the identification of clones but also provides insight into the efficacy of the transformation process. However, care should be taken in the design of experiments, as the use of multiple markers can complicate the screening process if not managed correctly.
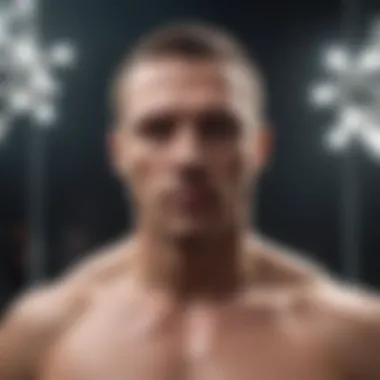
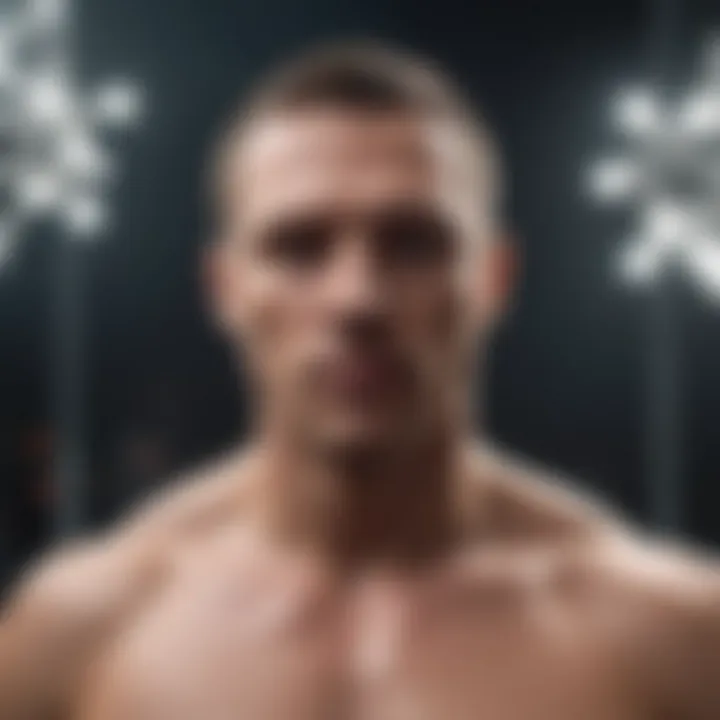
Blue-White Screening
Blue-white screening is another widely adopted technique employed to facilitate the selection of recombinant clones. This method relies on the activity of the lacZ gene, which encodes beta-galactosidase. When a plasmid vector containing this gene is used, successful ligation of an insert interrupts the lacZ gene, leading to the absence of the enzyme.
In this scenario, transformed bacterial colonies are plated on agar containing X-gal, a substrate for beta-galactosidase. Colonies that have taken up the vector without an insert will break down X-gal, producing blue colonies. Conversely, colonies harboring a recombinant plasmid that contains the insert will remain white due to the lack of beta-galactosidase activity.
"Blue-white screening provides a visually intuitive way to assess cloning success. It allows researchers to quickly identify colonies that could contain the desired insert."
Overall, both antibiotic resistance markers and blue-white screening are essential techniques for the effective screening and selection of clones. These methods enhance the efficiency and accuracy of molecular cloning, paving the way for successful genetic and biochemical explorations.
Applications of Molecular Cloning
Molecular cloning is a technique that has far-reaching applications across various fields, primarily in biological sciences. It allows researchers to isolate and manipulate specific DNA sequences, leading to advancements in gene therapy, protein engineering, and many other biotech innovations. Each application proves vital for scientific research and practical implementations, making this topic significant in the context of molecular biology.
Gene Identification and Characterization
Gene identification is a core aspect of molecular cloning. It involves pinpointing specific genes within a genome and understanding their functions. By employing cloning techniques, scientists can extract genes from organisms, analyze their sequences, and characterize the proteins they encode. This process is crucial in identifying genetic diseases. For instance, discovering mutations in human genes can lead to better diagnostic methods and therapeutic strategies.
Furthermore, molecular cloning facilitates the study of gene regulation and expression. Researchers use various vectors to introduce cloned DNA into host cells, allowing them to observe how specific genes behave under different conditions. This can illuminate the roles of genes in development, disease, and other biological processes.
Protein Production and Analysis
Another major application of molecular cloning is in the production and analysis of proteins. Cloning allows for the expression of recombinant proteins, which are proteins produced from genetically modified organisms. By inserting human genes into bacterial vectors, researchers can synthesize proteins essential for therapeutic applications. This is particularly useful in the production of hormones, antibodies, and enzymes, which can be used as treatments for various medical conditions.
The analysis of these proteins takes place after their production. Techniques such as SDS-PAGE and Western blotting are commonly employed to verify the size and purity of recombinant proteins. Understanding protein structure and function is critical for developing new medications and enhancing existing treatments.
Biotechnology and Genetic Engineering
Molecular cloning is foundational to biotechnology and genetic engineering. It allows scientists to create genetically modified organisms (GMOs) for agriculture, medicine, and research. For example, crops can be engineered to express traits like pest resistance or improved nutritional content. This application addresses challenges such as food security and sustainability.
In medicine, genetic engineering enables the development of gene therapies, where defective genes are corrected or replaced to treat genetic disorders. Such advancements highlight the importance of cloning techniques in addressing complex biological questions and societal challenges.
"The advancements in molecular cloning have revolutionized our approach to genetics, leading to practical solutions in medicine and agriculture."
Future Directions in Molecular Cloning
As we look to the future of molecular cloning, several pivotal trends are shaping its trajectory. Advancements in technology and new ethical debates highlight both opportunities and concerns in the field. This section aims to underscore the significance of future directions in molecular cloning, elaborating on emerging technologies and ethical considerations. Understanding these elements is essential for students, researchers, and professionals involved in genetic research and biotechnology.
Emerging Technologies
Emerging technologies have the potential to redefine molecular cloning practices. Techniques such as CRISPR-Cas9 genome editing, synthetic biology, and advanced sequencing methodologies are at the forefront of these developments.
- CRISPR-Cas9: This technology has revolutionized gene editing. By allowing precise modifications, it enhances the possibilities for genetic engineering. Researchers can efficiently insert, delete, or modify genes in various organisms.
- Synthetic Biology: This field fuses engineering principles with biology. It enables the design and construction of new biological parts, devices, and systems. Molecular cloning is a backbone of synthetic biology, providing the necessary tools for creating custom organisms.
- Next-Generation Sequencing (NGS): NGS allows rapid sequencing of DNA. It supports the understanding of genetic material on a larger scale, enabling researchers to analyze clones with greater efficiency and depth. This technology integrates seamlessly with cloning techniques.
These technologies represent just a portion of the advancements occurring in molecular cloning. They promise to enhance the precision and efficiency of cloning processes, leading to innovative applications across various scientific disciplines.
Ethical Considerations
With new technologies come ethical considerations that cannot be ignored. The power of molecular cloning and gene editing raises important questions about safety, morality, and the implications of manipulating genetic material. Several ethical issues are particularly relevant:
- Biosafety: As cloning techniques become more advanced, ensuring the safety of new organisms and products is paramount. Scientists must consider potential ecological impacts before releasing genetically modified organisms into the environment.
- Germline Editing: Editing genes in human embryos brings forth debates about the long-term consequences. Ethical implications of such edits, including unintended consequences and societal impacts, require careful consideration.
- Access and Equity: As molecular cloning technologies advance, accessibility becomes critical. Questions arise about who can utilize these technologies. Ensuring equitable access to cloning advancements is an ongoing concern.
In summary, while emerging technologies in molecular cloning offer substantial benefits, they also necessitate careful ethical scrutiny. Discussions among the scientific community, regulatory bodies, and the public are essential to navigate these complex issues. By addressing both innovations and the accompanying ethical challenges, we can foster responsible advances in molecular cloning.
The End
Molecular cloning is foundational to the life sciences. It allows researchers to manipulate DNA, leading to significant advancements in genetics, biotechnology, and medicine. In this article, we explored the diverse methodologies and principles encapsulated in Sambrook and Russell's authoritative work.
Summary of Key Points
The article delves into several critical aspects of molecular cloning:
- Introduction to Molecular Cloning: We defined molecular cloning and discussed its historical context, tracing its evolution in the scientific landscape.
- Sambrook and Russell's Contribution: The manual's updates over editions highlight its role as a cornerstone for lab practices.
- Cloning Vectors: The section outlines various types of vectors and emphasizes choosing the right one for particular experiments.
- Restriction Enzymes: Understanding their functions and the most commonly used enzymes is crucial for effective cloning.
- DNA Ligation Techniques: We considered ligase enzymes and the methods of ligation, critical for successful gene recombination.
- Transformation Methods: This section covers electroporation and natural transformation, which are key for introducing DNA into host cells.
- Screening and Selection of Clones: Techniques such as antibiotic resistance markers and blue-white screening were examined for their roles in identifying successful clones.
- Applications of Molecular Cloning: From gene characterization to protein production, this lays out its vast potential in various fields.
- Future Directions in Molecular Cloning: We touched on emerging technologies and ethical considerations that shape the future of cloning research.
Overall, these points underscore the importance of mastering molecular cloning techniques and continuously updating knowledge with advancements in the field.
The Impact of Molecular Cloning on Science
The impact of molecular cloning extends beyond individual experiments. It catalyzes significant breakthroughs in understanding genetic functions and interactions. The manipulation of genetic material fosters innovations in areas like gene therapy, which holds promise for treating genetic disorders.
Furthermore, molecular cloning is instrumental in vaccine development and agriculture, contributing to food security through genetically modified crops. These applications illustrate how the principles established in Sambrook and Russell's manual can influence both fundamental and applied sciences.