Understanding Sickle Cell Hemoglobin Sequence Impacts
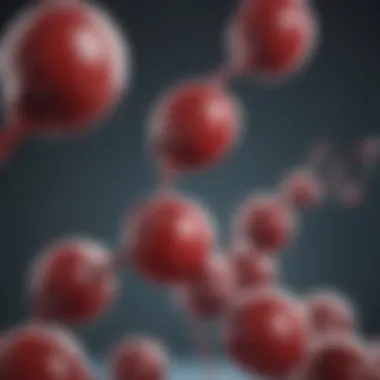
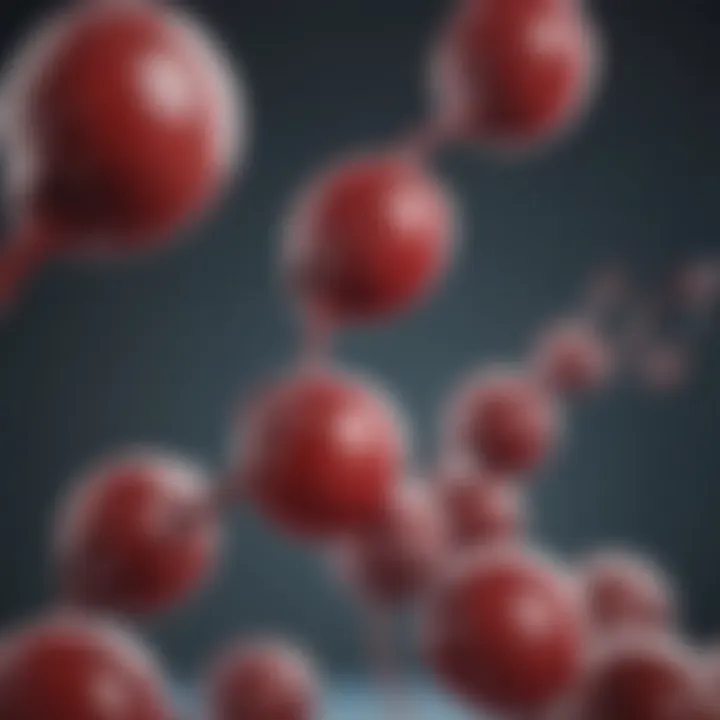
Intro
Sickle cell disease is a genetic disorder caused by mutations in the hemoglobin molecule. This disease leads to various complications due to the altered shape and function of red blood cells. Understanding the amino acid sequence of sickle cell hemoglobin is pivotal in elucidating the disease's pathogenic mechanisms. This article aims to explore these amino acid alterations, their functional consequences, treatment implications, and future therapeutic avenues.
Research Overview
Summary of Key Findings
Recent studies have highlighted that the primary mutation in sickle cell hemoglobin involves the substitution of valine for glutamic acid at the sixth position of the beta-globin chain. This seemingly minor change triggers a cascade of events leading to the characteristic sickling of red blood cells.
- The distorted red blood cells can become lodged in small blood vessels, causing pain and organ damage.
- The study of the amino acid sequence reveals how sickle cell hemoglobin behaves differently under low oxygen conditions compared to normal hemoglobin.
- The mutation influences the overall stability of the hemoglobin molecule, impairing its ability to carry oxygen effectively.
Importance of the Research
Understanding the molecular genesis of sickle cell disease is crucial for several reasons. First, it underpins the development of targeted therapies that address the root cause rather than just managing symptoms. Second, insights from the amino acid sequence can guide genetic counseling and testing for at-risk populations. Moreover, it can inform public health strategies aimed at reducing the prevalence and impact of the disease in communities.
"The exploration of sickle cell hemoglobin at the molecular level sheds light on potential therapeutic targets and innovative treatment approaches, paving the way for advancements in patient care."
Methodology
Study Design
The approach to studying sickle cell hemoglobin often involves structural biology techniques, such as X-ray crystallography and nuclear magnetic resonance (NMR) spectroscopy. These methods allow for detailed visualization of the hemoglobin structure, enhancing our understanding of how specific amino acid sequences influence function.
Data Collection Techniques
Data collection typically encompasses:
- Gene sequencing to identify mutations in the beta-globin gene.
- Biochemical assays to evaluate hemoglobin function under various conditions.
- Clinical investigations to correlate genotypic data with phenotypic outcomes in patients.
This methodological framework provides robust insights into the significance of sickle cell hemoglobin and the myriad effects it has on health outcomes.
Preface to Hemoglobin
Hemoglobin is a critical protein found in red blood cells, responsible for the transport of oxygen from the lungs to the tissues and facilitating carbon dioxide removal. Understanding hemoglobin's structure and function is vital, especially in the context of sickle cell disease, a condition that stems from molecular changes in this protein. The link between hemoglobin structure and disease pathology can provide insights for enhancing treatment strategies. This section will explore the basics of hemoglobin, emphasizing its significance in the pathophysiology of sickle cell disease.
Overview of Hemoglobin Function
The primary function of hemoglobin is to carry oxygen. Hemoglobin can bind oxygen molecules in the lungs due to its unique structure, which features four iron-containing heme groups. Each of these groups can hold one oxygen molecule. When oxygen binds, hemoglobin changes shape, facilitating its release in oxygen-poor tissues.
Moreover, hemoglobin plays a role in managing blood pH by balancing bicarbonate and carbon dioxide levels. The interactions between oxygen and hemoglobin contribute to the efficient delivery of oxygen, highlighting the importance of this protein in human physiology. In sickle cell disease, the normal functioning of hemoglobin is compromised, leading to severe health complications.
Composition of Hemoglobin
Hemoglobin is synthesized as a tetramer consisting of two alpha and two beta globin chains. The precise arrangement of amino acids within these chains influences hemoglobin's overall stability and functionality. The amino acid sequence is critical; any alteration can result in significant changes. In individuals with sickle cell disease, a single substitution in the beta-globin chain—where glutamic acid is replaced by valine—disrupts normal hemoglobin structure, transforming it into hemoglobin S (HbS).
This variant exhibits less solubility under low oxygen conditions, leading to sickling of red blood cells. Such a transition illustrates how minute changes at the molecular level can manifest in profound health consequences. Understanding these compositional details is essential for researchers and healthcare professionals working to develop better interventions and therapies for sickle cell disease.
"The entire physiology of blood oxygen transport hinges on the delicate structures formed by hemoglobin's amino acid composition, underlining the significance of each amino acid in maintaining health."
As we progress through the article, we will clarify how these foundational elements relate specifically to the amino acid sequence of sickle cell hemoglobin and its broader implications for both health outcomes and therapeutic advancements.
Understanding Sickle Cell Disease
Understanding sickle cell disease (SCD) is crucial for comprehending the broader implications of sickle cell hemoglobin's amino acid sequence. SCD is a hereditary form of anemia, characterized by the production of abnormal hemoglobin, known as hemoglobin S. This disease significantly impacts the quality of life for those affected and poses various health challenges. Grasping its essential elements helps in understanding the genetic, clinical, and societal dimensions of this condition.
Definition and Characteristics
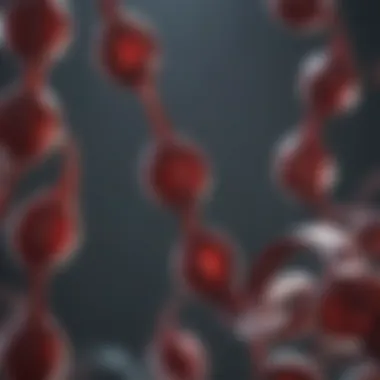
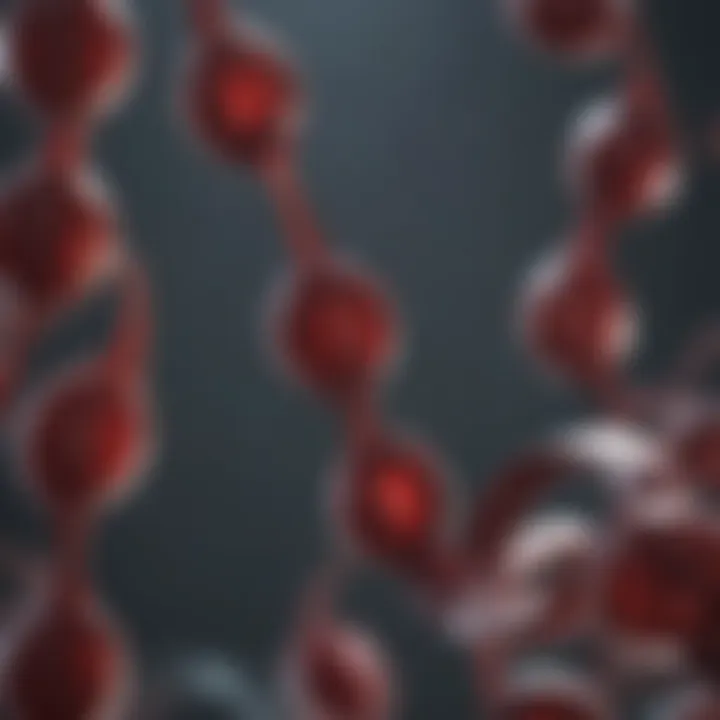
Sickle cell disease arises from a mutation in the HBB gene, which encodes the beta-globin component of hemoglobin. This genetic alteration substitutes the amino acid valine for glutamic acid at the sixth position of the beta-globin chain. The result is the formation of hemoglobin S instead of normal hemoglobin A.
People with SCD can experience a range of symptoms, including severe pain episodes known as sickle cell crises. These occur due to the sickle-shaped cells obstructing blood flow in small vessels. Other characteristics include increased susceptibility to infections, delayed growth, and complications from organ damage. Recognizing these features is vital for effective management and treatment strategies.
Epidemiology of Sickle Cell Disease
The distribution of sickle cell disease reveals important trends in public health. SCD is most prevalent in regions where malaria is or was common, particularly in Sub-Saharan Africa, parts of India, and the Mediterranean. In the United States, SCD affects around 100,000 people, primarily among African Americans. The following points summarize key epidemiological insights:
- Inheritance: Sickle cell disease follows an autosomal recessive inheritance pattern. A child must inherit two copies of the mutated gene to express the disease.
- Global Impact: Millions carry the sickle cell trait globally, while an estimated 300,000 babies are born with SCD each year.
- Public Health Considerations: Awareness and screening programs are essential for early diagnosis. Health systems must address the specific needs of affected populations.
Understanding the epidemiological aspects of sickle cell disease aids in allocating resources and formulating interventions to improve health outcomes.
The Amino Acid Sequence of Sickle Cell Hemoglobin
Understanding the amino acid sequence of sickle cell hemoglobin is crucial for grasping the molecular basis of sickle cell disease. The structure of hemoglobin significantly influences its ability to transport oxygen and maintain red blood cell shape. This sequence determines how hemoglobin interacts with other molecules and how these interactions are impacted by genetic alterations. Thus, the examination of the amino acid sequence not only sheds light on the functional deviations seen in sickle cell hemoglobin but also has implications for treatment and therapeutic advancements.
Defining the Amino Acid Structure
The amino acid structure of sickle cell hemoglobin is fundamentally altered from that of normal hemoglobin due to a single point mutation. This mutation occurs in the beta-globin gene, where adenine is replaced by thymine, leading to the substitution of valine for glutamic acid at position six of the beta chain. This seemingly minor change has profound effects on hemoglobin's properties, including solubility and stability.
Normal adult hemoglobin, known as hemoglobin A, has a sequence that allows for flexible interactions with oxygen. In contrast, the sickle variant, hemoglobin S, arises from the altered sequence resulting in a proclivity for polymerization under deoxygenated conditions, which causes red blood cells to adopt a sickle shape. This configuration compromises their ability to navigate through small blood vessels, leading to a myriad of complications associated with sickle cell disease.
Comparative Analysis of Normal and Sickle Cell Hemoglobin
A detailed comparative analysis reveals striking differences between normal hemoglobin A and sickle cell hemoglobin S. These differences can be summarized as follows:
- Solubility: Hemoglobin A remains soluble even in the lower oxygen states, while hemoglobin S tends to precipitate, forming fibers. This precipitation results in the distortion of red blood cells into a sickle shape.
- Oxygen Affinity: Hemoglobin S has a different oxygen-binding affinity due to the valine substitution. It generally has a lower affinity for oxygen compared to hemoglobin A, which exacerbates the sickling process during deoxygenation episodes.
- Stability: Hemoglobin A is stable and does not form aggregates. However, hemoglobin S incorporates into aggregates under low oxygen conditions, contributing to the rigid sickle cell morphology.
Implications of the amino acid sequence can be seen not only in the clinical presentation of disease but also in potential therapeutic interventions.
These molecular characteristics have direct implications for patients. For instance, understanding these differences is essential for developing targeted therapies, like gene editing or novel drug formulations, aimed at modifying hemoglobin's properties to maintain a normal red blood cell shape and function.
Molecular Mechanisms Underlying Sickle Cell Disease
The study of molecular mechanisms underlying sickle cell disease is essential. Understanding these mechanisms sheds light on the physiological consequences of sickle cell hemoglobin. Sickle cell disease originates from a single point mutation in the beta-globin gene, leading to notable transformations in the hemoglobin molecule. The alterations at the molecular level are crucial to grasp how sickle cell disease causes various health complications.
Pathophysiology of Sickle Cell Hemoglobin
Sickle cell hemoglobin (HbS) presents with unique pathophysiological characteristics. Unlike normal hemoglobin (HbA), the modified structure of HbS causes red blood cells to deform into a sickle shape under conditions like low oxygen saturation. This sickling of red blood cells results in several consequences:
- Vascular occlusion: Sickled cells can block small blood vessels, leading to ischemia and pain.
- Hemolysis: The abnormal shape makes these cells more susceptible to destruction, resulting in chronic hemolytic anemia.
- Reduced oxygen transport: The compromised ability of sickled cells to carry oxygen contributes to fatigue and other symptoms.
These pathological features underscore the relevance of the amino acid sequence, specifically the substitution of valine for glutamic acid at position six of the beta-globin chain. Such a seemingly minor change has profound effects on the entire hemoglobin structure, emphasizing the need for further studies on these molecular dynamics.
Impact of Mutations on Hemoglobin Function
The mutations present in sickle cell hemoglobin significantly impair its function. The introduction of valine alters the hydrophobicity, promoting polymerization of HbS. Hence, when oxygen levels drop, hemoglobin aggregates into long, rigid polymers. This polymerization process leads to the aforementioned sickling phenomenon. The consequences of these mutations include:
- Decreased oxygen affinity: HbS has a lower affinity for oxygen compared to HbA, which can further compromise oxygen delivery to tissues.
- Increased sickling tendency: With higher concentrations of hemoglobin, sickling becomes increasingly frequent, especially during exertion or in environments with low oxygen levels.
- Complications in management: Understanding the mutations helps in developing targeted therapies. Advances in gene editing, like CRISPR technology, aim to correct the mutation at the genomic level.
Understanding the molecular mechanisms of sickle cell disease provides essential insights into its treatment and management strategies. As research advances, the hope is that these insights can lead to innovative therapies and improved patient outcomes.
Genetic Aspects of Sickle Cell Disease
Understanding the genetic underpinning of sickle cell disease is vital for both research and clinical applications. Genetic aspects provide insights into how the disease is inherited, which can inform prevention and management strategies. The focus on genetics reveals the molecular basis of the disease and opens doors to targeted therapies. In this section, we will explore inheritance patterns and the population genetics linked to sickle cell trait.
Inheritance Patterns
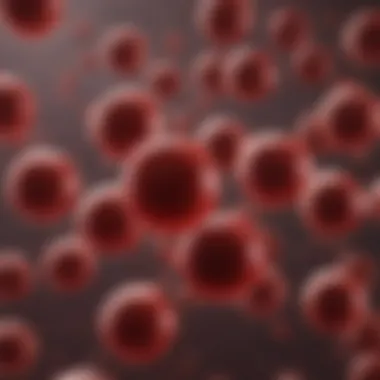
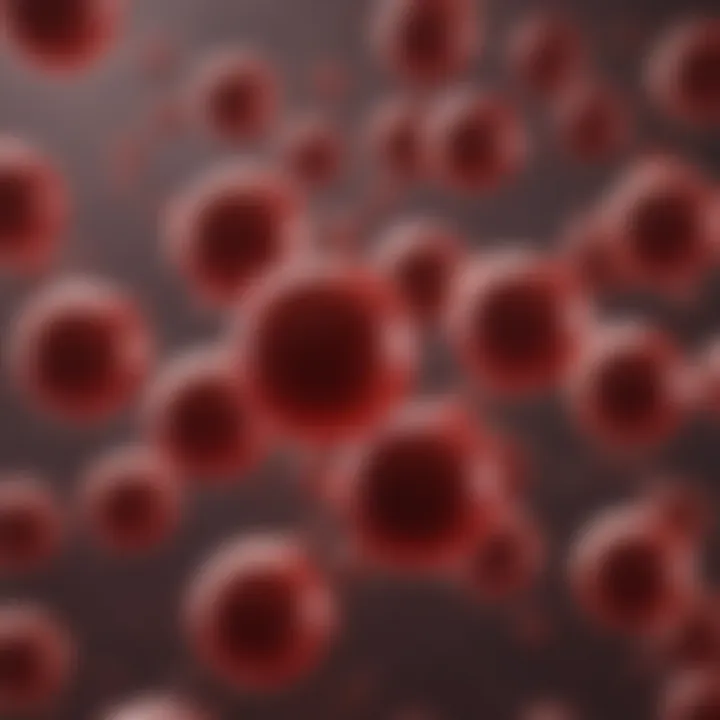
Sickle cell disease primarily follows an autosomal recessive inheritance pattern. Individuals must inherit two copies of the sickle cell gene, one from each parent, to exhibit symptoms of the disease. If a person receives only one copy of the mutated gene, they will be a carrier, often referred to as having sickle cell trait.
This genetic arrangement has significant implications:
- Carrier Status: Understanding carrier status is crucial for family planning. Both parents must be aware of their sickle cell trait status to assess the risk of having an affected child.
- Genetic Counseling: Genetics can guide counseling efforts, aiding families in making informed reproductive choices based on the likelihood of passing on the disease.
The prevalence of sickle cell disease varies by geographic region, influenced by factors such as evolutionary pressures, particularly in malaria-endemic areas. In some regions, such as Sub-Saharan Africa, high incidence rates of the sickle cell trait exist, providing a survival advantage against malaria.
Population Genetics of Sickle Cell Trait
Population genetics dives into the distribution and frequency of sickle cell alleles within different populations. The sickle cell gene is not uniformly distributed globally, primarily due to historical selective pressures. Here are some key points:
- Geographic Distribution: Areas with a high prevalence of malaria show a corresponding rise in sickle cell trait carriers, illustrating a classic example of natural selection. This results in a higher evolutionary fitness of those who carry as it reduces malaria morbidity.
- Genetic Drift: In isolated populations, genetic drift can enhance or diminish allele frequencies, thus affecting the local incidence of sickle cell trait.
- Implications for Public Health: Understanding the population genetics helps in developing targeted public health initiatives. For instance, educational programs in high-prevalence regions can help inform potential carriers about the risks of having children affected by sickle cell disease.
"The knowledge of genetic principles is key to addressing diseases like sickle cell and managing their impact across populations."
Diagnostic Techniques for Sickle Cell Disease
The significance of diagnostic techniques in the management of sickle cell disease cannot be overstated. Timely and accurate diagnosis is critical for optimizing treatment and improving patient outcomes. Various methods have been developed to diagnose this condition, which are vital not only for patients but also for public health initiatives.
Methods of Diagnosis
Several methodologies are utilized to diagnose sickle cell disease. The primary methods include:
- Hemoglobin electrophoresis: This is the most commonly used test. It separates different types of hemoglobin present in the blood. By analyzing the patterns, clinicians can determine the presence of sickle hemoglobin (HbS) and other variants.
- Complete blood count (CBC): A CBC helps assess the overall health of a patient and detect various disorders, including anemia, which is common in sickle cell patients. Low hemoglobin levels may indicate sickle cell disease.
- Newborn screening: In many regions, screening for sickle cell disease occurs at birth. This is crucial for early intervention and management, thereby reducing complications.
- Genetic testing: This can identify mutations in the HBB gene, which encodes the beta globin subunit of hemoglobin. Genetic testing provides definitive diagnosis and can also determine carrier status in family members.
These diagnostic methods are important. They not only confirm the presence of sickle cell disease but also guide treatment decisions and patient management.
Role of Genetic Screening
Genetic screening plays a pivotal role in managing sickle cell disease. Its importance lies in several key aspects:
- Early Detection: Genetic screening can identify carriers of the sickle cell trait. This information is crucial for family planning and prenatal counseling.
- Tailored Treatment Plans: Understanding a patient's specific genotype allows healthcare providers to tailor treatment strategies. For instance, individuals with the HbSC genotype may require different management compared to those with HbSS.
- Public Health Insights: Genetic screening can also provide data for epidemiological studies. This contributes to understanding the prevalence of sickle cell trait and disease within specific populations.
- Community Education: Knowledge of sickle cell trait within communities can lead to increased awareness and understanding of the disease, promoting preventive measures and testing.
Genetic screening is an invaluable tool that enhances both individual patient care and broader public health initiatives.
Clinical Implications of Sickle Cell Hemoglobin
Sickle cell hemoglobin is at the core of understanding the broader clinical implications of sickle cell disease. The changes in the amino acid sequence of hemoglobin precipitate various health challenges. Identifying these complications allows healthcare professionals to tailor effective strategies for management, improving patient outcomes.
Health Complications Related to Sickle Cell Disease
Sickle cell disease manifests through a range of health complications that significantly impact patients' lives. Most prominent among these is vaso-occlusive crisis, where red blood cells become rigid and obstruct blood flow. This blockage can lead to severe pain and organ damage. Other complications involve anemia due to the rapid breakdown of sickled cells, which leads to fatigue and weakness.
Infections pose another significant risk, as individuals with sickle cell disease often have compromised spleen function. This leaves them vulnerable to bacteria and other pathogens. Additionally, there are acute chest syndromes, stroke risk, and increased incidences of kidney impairment. Enumerating these complications aids in understanding how deeply sickle cell disease influences patients' overall health.
- Vaso-occlusive crisis
- Anemia
- Increased risk of infections
- Acute chest syndrome
- Stroke risk
- Kidney impairment
More than physical health, psychological wellbeing also suffers. Constant pain and medical visits can lead to anxiety and depression, further complicating the disease's management.
Management and Treatment Strategies
Effective management of sickle cell disease requires a multi-faceted approach. Current strategies range from preventative measures to acute care interventions. The choice of treatment is influenced by the severity of symptoms, age, and overall health of the patient.
- Pain Management: This is crucial during a vaso-occlusive crisis. Opioids and non-opioid analgesics are used to alleviate pain. Hospitals may administer hydration therapy.
- Hydroxyurea: This medication can reduce the frequency of crises and the need for blood transfusions. Hydroxyurea works by increasing fetal hemoglobin levels, which decreases sickling tendency.
- Blood Transfusions: These can be lifesaving in acute scenarios. Regular transfusions may prevent stroke in high-risk patients.
- Antibiotics and Vaccinations: Preventive antibiotics help reduce infection risk, while vaccinations target prevalent pathogens like pneumonia and meningitis.
- Gene Therapy: Emerging as a promising avenue, this could provide long-term solutions. Clinical trials are ongoing.
Regular follow-up and health screenings are essential to monitor complications and treatment efficacy. Education on lifestyle modifications and adherence to treatment regimens plays a tremendous role in managing sickle cell disease effectively.
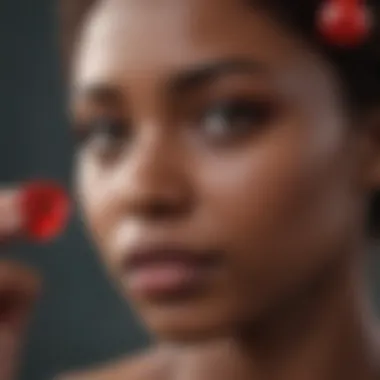
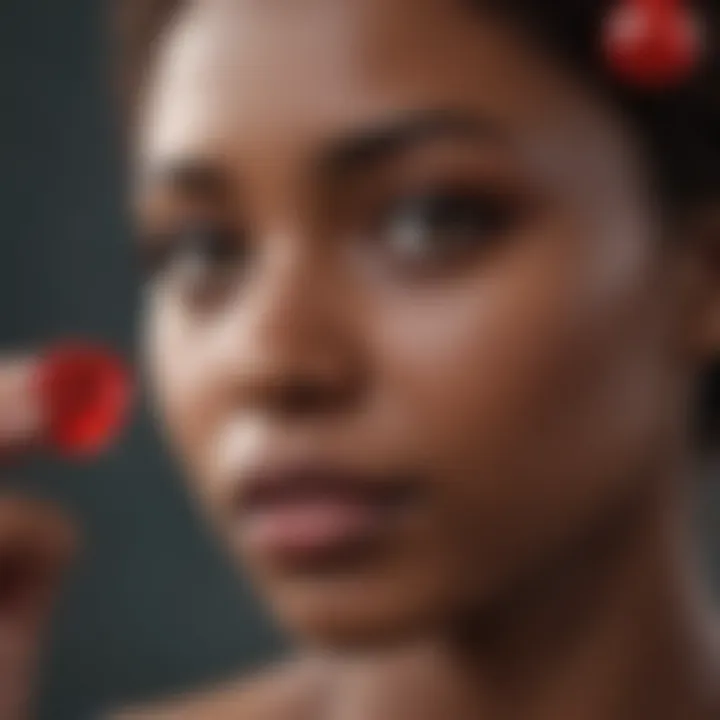
Overall, understanding the clinical implications of sickle cell hemoglobin not only enhances patient care but also informs ongoing research in seeking better treatment modalities.
Promising Research and Future Directions
Research into the amino acid sequence of sickle cell hemoglobin has significant implications for both understanding and treating sickle cell disease. As scientists investigate this fundamental genetic disorder, multiple opportunities arise for innovative therapies that could transform patient outcomes. The significance of continued exploration in this area cannot be overstated. Advancements in gene therapy approaches may hold the key to reversing the detrimental effects caused by mutations in the hemoglobin structure. By enhancing our understanding of the molecular mechanisms at play, researchers can pave the way for effective interventions that address the root causes of the disease.
One primary element to consider is the potential for ex vivo gene editing. This technique allows scientists to modify a patient’s own stem cells outside the body before reintroducing them. Such advancements could lead to long-term management and possibly a cure for sickle cell disease. The prospect of editing genes precisely, targeting the specific mutation responsible for sickle hemoglobin, could lead to a synthesis of normal hemoglobin, alleviating the sharp, debilitating effects of sickle cells on the body.
Advancements in Gene Therapy
Recent progress in gene therapy, particularly regarding sickle cell disease, has garnered significant attention. The application of viral vectors to deliver corrective genes can potentially alter the trajectory of the disorder. For instance, a prominent case study involves the use of lentiviral gene therapy, which introduces a functional gene copy that encodes healthy hemoglobin. This significant development indicates a leap in the ability to provide personalized treatments catered to individual genetic profiles.
Several clinical trials have yielded promising results, suggesting that patients can achieve sustained production of normal hemoglobin, markedly reducing the severity of their symptoms. These advancements emphasize the transformative potential of tailored therapies in managing sickle cell disease and highlight the importance of continuous research into genetic interventions.
Additionally, understanding the long-term effects and possible side effects of these therapies remains a priority. As we push forward, considerations around delivery methods, cost, and accessibility will be crucial.
Future Research Priorities
As the research landscape evolves, future priorities in sickle cell studies should focus on both broadening the understanding of disease mechanisms and refining therapeutic approaches. Key areas for upcoming investigation include:
- Efficacy of Combination Therapies: Exploring the use of gene therapy in conjunction with existing treatments, such as hydroxyurea, could harness complementary mechanisms to enhance patient outcomes further.
- Longitudinal Studies on Patient Outcomes: Continuous assessment of patients post-gene therapy will provide invaluable insights into long-term effects and effectiveness of treatments, informing further innovations.
- Patient Demographics and Diversity: Investigating how genetic backgrounds affect treatment responses can help tailor therapies for various populations, ensuring equitable healthcare advancements.
- Collaboration with Computational Models: Utilizing bioinformatics tools can identify potential genetic targets and predict the effects of mutations on hemoglobin function, streamlining the research process.
By focusing on these research priorities, the scientific community can not only enhance the understanding of sickle cell hemoglobin but also foster the development of groundbreaking treatments that directly improve patients' lives.
"Advancements in gene therapy represent a crucial frontier in the battle against sickle cell disease, with the potential to transform the lives of countless individuals."
Exploring the interplay of genetics, innovative therapies, and patient-centered research will undoubtedly pave the way for future breakthroughs, impacting public health strategies and, ultimately, the global fight against sickle cell disease.
Societal Impacts of Sickle Cell Disease
The societal impacts of sickle cell disease extend beyond individual health complications, reaching into broader realms of public health, economics, and education. Understanding these impacts is essential for creating effective policies and initiatives aimed at supporting those affected by the condition. Sickle cell disease is not just a medical issue but also a challenge that affects families, communities, and healthcare systems.
Public Health Strategies
Public health strategies are vital for managing the impact of sickle cell disease in populations. These strategies often focus on screening, prevention, and management of health complications related to the condition. Here are some relevant components of effective public health strategies:
- Universal Screening: Implementing routine newborn screening for sickle cell disease can lead to early diagnosis. This is essential for starting treatment promptly and improving health outcomes.
- Access to Care: Enhancing access to specialized care through comprehensive sickle cell centers can improve patient management. These centers can offer interdisciplinary services that address the multifaceted nature of the disease.
- Preventive Care: Focusing on preventative measures, such as vaccinations and antibiotics, can ward off infections that are more likely to affect individuals with sickle cell disease.
- Crisis Management Protocol: Developing clear guidelines helps manage pain crisis effectively. Training healthcare professionals about the specifics of the disease ensures proper intervention when crises occur.
Public health strategies are often strengthened by community involvement and multi-sector collaboration. It is notable to emphasize that well-structured public health initiatives also reduce economic burdens by preventing complications and hospitalizations.
Educational Initiatives for Awareness
Education plays a critical role in raising awareness about sickle cell disease among the public, healthcare providers, and policymakers. Awareness is key to combating stigma and ensuring those affected receive the support they need. Effective educational initiatives address various aspects:
- Community Outreach Programs: Engaging with communities through workshops and seminars can disseminate information about sickle cell disease, its inheritance, and its impact. This helps reduce misconceptions and fosters support networks.
- Training for Healthcare Providers: Educating healthcare professionals on the latest management protocols and treatment options is paramount. This ensures timely and appropriate care, significantly influencing patient outcomes.
- Incorporation of Curriculum in Schools: Including information about sickle cell disease in school curriculums can foster understanding from a young age. Children educated about the condition are more likely to show empathy and understanding towards their peers who are affected.
- Online Resources: Providing accessible online education materials can reach a wider audience. Platforms like Wikipedia and Britannica offer comprehensive overviews that are beneficial for self-education.
Ultimately, educational initiatives encourage community partnership, which can lead to improved health outcomes and quality of life for individuals living with sickle cell disease. This easier access to information can play a substantial role in shaping public perception and promoting a supportive environment.
The End and Summary of Findings
Understanding the significance of the amino acid sequence of sickle cell hemoglobin is crucial in numerous domains of biomedical research, genetics, and public health. The focus on sickle cell hemoglobin links genetic mutations to a wide array of clinical outcomes. This relationship is vital for developing effective treatment strategies and for advancing research into new molecular therapies.
Recapitulation of Key Points
- Amino Acid Sequence Importance: The specific alterations in the amino acid sequence of hemoglobin result in the polymerization that characterizes the sickle shape of red blood cells, leading to complications in patients with sickle cell disease.
- Molecular Mechanisms: The pathophysiology of sickle cell disease is closely tied to how mutations in the hemoglobin gene affect red blood cell structure and function, which in turn affects oxygen transport in the body.
- Clinical Implications: Recognizing these mutations enables healthcare providers to implement targeted management strategies, including blood transfusions and hydroxyurea treatment, which can significantly improve patient outcomes.
- Public Health Strategies: Integrating knowledge from genetics and population studies allows for better public health interventions aimed at screening and educating affected populations.
- Future Directions: Continued research into gene therapy holds promise for altering the sickle hemoglobin mutations at the molecular level, which may lead to groundbreaking therapeutic options.
Final Thoughts on Sickle Cell Hemoglobin Research
Research surrounding sickle cell hemoglobin is not just limited to understanding the disease itself but extends into broader implications for genetic disorders. The intricate relationship between genetic variability and clinical manifestations reflects the complexity of human biology. By analyzing sickle cell hemoglobin's amino acid alterations, we are also learning valuable lessons about gene expression, mutation impacts, and potential new therapies applicable to other disorders.
Furthermore, the societal impacts of sickle cell disease cannot be overstated. Awareness and educational initiatives should work hand-in-hand with scientific advancements to create a comprehensive response to sickle cell disease. This dual approach ensures that insights from research translate into real-world benefits for those diagnosed. The insights gathered from studying sickle cell hemoglobin also drive innovation in healthcare, potentially informing approaches for other hemoglobinopathies and genetic disorders.
Understanding the amino acid sequence of sickle cell hemoglobin is crucial for future advances in treatments and health management strategies.