Bioenergy: Sustainability, Developments, and Challenges
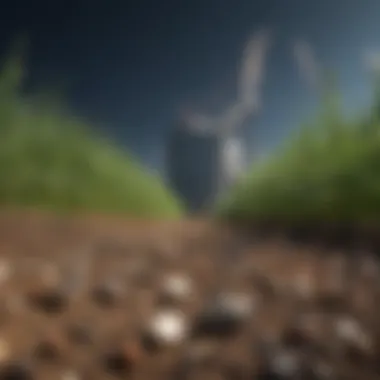
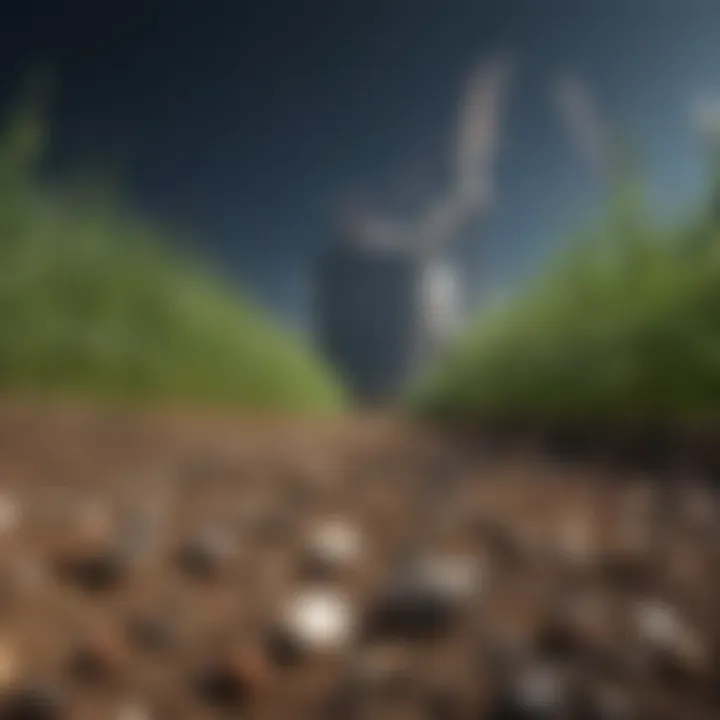
Intro
Bioenergy has emerged as a significant player in the energy landscape, representing an alternative to fossil fuels that aligns with sustainability goals. This field incorporates various forms of energy derived from biological materials, tailoring itself as societies look for greener, more renewable sources of energy. With the ongoing climate crisis, the exploration of bioenergy offers numerous possibilities that, if tapped wisely, can be pivotal in addressing both environmental and economic challenges.
The abundance of biomass – from agricultural waste to forestry residues – provides a treasure trove of resources that can be converted into energy. As technology advances, the production methods and efficiency of bioenergy conversion have also evolved, making the sector more appealing to investors and researchers alike.
In this article, we turn the spotlight on bioenergy; exploring its fundamentals and developments, and examining the implications it holds for our energy future. Knowledge is power, and understanding bioenergy could provide crucial insights necessary to drive the transition towards more sustainable energy practices.
Prolusion to Bioenergy
Bioenergy is a pivotal component of renewable energy strategies and embodies a promising avenue towards sustainable energy solutions. This section aims to shed light on bioenergy's importance, reflecting on its varied benefits and considerations. As the world steers into a future heavily reliant on reducing carbon emissions, understanding bioenergy becomes key. It not only serves as an alternative avenue for energy but also aids in waste management, promotes rural economies, and helps in carbon dioxide reduction.
Advancing our knowledge about bioenergy directs us to innovative practices in energy production, turning what many consider waste into valuable resources.
Defining Bioenergy
Defining bioenergy can seem straightforward, yet it carries layers of complexity. At its core, bioenergy is derived from organic materials, commonly known as biomass. This encompasses a vast array of sources, including plant materials, agricultural and forestry residues, and organic waste. Discussing bioenergy evokes thoughts of alternative fuels derived from renewable sources, which can be utilized for heating, electricity, or as biofuels for transportation. It's not merely an abstract concept but a practical solution towards alleviating some of our pressing energy concerns.
When we break it down, bioenergy taps into the sun’s energy captured by plants through photosynthesis. This process embodies the cyclical nature of ecosystems, where plant matter is regenerated, thereby sustaining the energy flow. In contemporary times, bioenergy solutions such as ethanol made from corn or biodiesel from vegetable oils have gained prominence, proving that harnessing and converting biological materials can yield significant energy outputs.
Historical Context
To appreciate the evolution of bioenergy, we must step back in time. The history of bioenergy dates back to ancient civilizations that relied on wood and other organic materials for heat and food preparation. The use of biomass was not merely practical but woven into the cultural fabric of societies around the world.
As we glide into the industrial revolution, the use of bioenergy saw fluctuations. The advent of fossil fuels changed the landscape dramatically, pushing bioenergy to the backburner. However, interest resurged during the oil crises of the 1970s, where the world began to recognize the volatility of fossil fuels and the implications of their consumption.
Now, with climate change at the forefront of global discussions, bioenergy stands once again as a viable alternative. Governments and policymakers are increasingly looking towards bioenergy as a strategic element of energy independence and sustainability. It’s fascinating to see how a resource that once flickered on the edge of extinction could now illuminate our path toward a cleaner and greener future.
"Bioenergy renews the intimate connection between the planet's ecosystems and our energy needs, prompting a necessary reevaluation of our environmental practices."
As bioenergy continues to evolve, it embodies the challenge and promise of modern energy production, advocating for the responsible management of our planet's resources.
Types of Bioenergy
Understanding the types of bioenergy is crucial in grasping its potential impact on sustainable energy practices. Each type comes from a unique source and caters to specific energy needs. This diversity not only enhances the energy portfolio but also helps in reducing dependency on fossil fuels. As we dive deeper, it becomes apparent that solid biofuels, liquid biofuels, and biogas play significant roles in shaping the future of renewable energy.
Solid Biofuels
Solid biofuels primarily include biomass like wood pellets, chips, and agricultural residues. These materials are advantageous for several reasons. First off, they are often by-products from other industries, making them relatively inexpensive and easy to source. Moreover, solid biofuels can be used for both heating and electricity generation, providing a dual benefit.
One notable aspect of solid biofuels is their carbon neutrality. When burned, they release carbon dioxide that was previously absorbed by the plants during their growth. Thus, the lifecycle results in no net increase of carbon in the atmosphere, which is a big win for climate change mitigation. However, the sourcing of solid biofuels also raises some concerns. Overharvesting forests or using food crops for fuel can lead to biodiversity loss and food insecurity.
"Sourcing solid biofuels must be balanced with ecological and community needs to ensure a truly sustainable approach."
While solid biofuels present a promising option, it’s important to approach their production and use with caution.
Liquid Biofuels
Liquid biofuels, like bioethanol and biodiesel, are derived from various feedstocks such as sugarcane, corn, and even waste cooking oil. Their importance lies in their ability to directly replace conventional fuels in transportation. With the transportation sector being a major contributor to greenhouse gas emissions, the integration of liquid biofuels offers a pathway toward reducing this impact.
Bioethanol, often used in gasoline mixtures, burns cleaner than regular gasoline, which means fewer carbon emissions and better air quality. Biodiesel, on the other hand, can be used in diesel engines without modifications. The flexibility afforded by liquid biofuels is a significant consideration when discussing energy diversification.
However, some challenges exist. The competition for land between food and fuel production poses a serious dilemma. If not managed well, the production of liquid biofuels may inadvertently lead to higher food prices and land use changes that affect ecosystems and communities.
Biogas
Biogas is generated through anaerobic digestion, where organic matter, such as food scraps or animal manure, is broken down by microorganisms in the absence of oxygen. This process produces a mixture of gases, primarily methane, which can be utilized for various energy needs, including heating and electricity generation.
The significance of biogas lies in its waste-to-energy potential. By utilizing waste products, we not only produce energy but also manage waste effectively, reducing landfill contributions. Furthermore, biogas system facilities can enhance local economies by creating jobs in waste management and energy production. There are also added benefits to soil quality and reduced reliance on chemical fertilizers when digestate is used as an organic fertilizer.
Nevertheless, biogas production can have its hurdles. Initial investments in infrastructure can be high, and the efficiency of energy extraction needs to be improved for widespread adoption. Additionally, community acceptance can be an issue if not properly communicated and managed.
Through these three types of bioenergy—solid biofuels, liquid biofuels, and biogas—the potential to utilize renewable resources presents a pathway towards a sustainable energy future. However, scrutinizing these options further is necessary to strike a balance between energy production, environmental impact, and social responsibility.
Sourcing Bioenergy
When exploring bioenergy, understanding the sourcing aspects is crucial. The way bioenergy is produced directly relies on the feedstocks that are harvested from various sources. These feedstocks are not only pivotal for biofuel production but also dictate the sustainability and economic viability of bioenergy solutions. Sourcing bioenergy effectively involves a balance of utilizing resources that are abundant yet ensuring that the environmental impact is minimized. Several key elements come into play here, including types of feedstocks, sustainability considerations, and how this sourcing can align with overall energy goals.
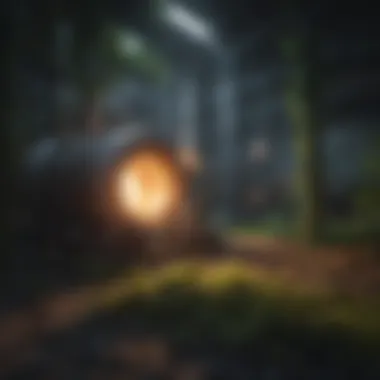
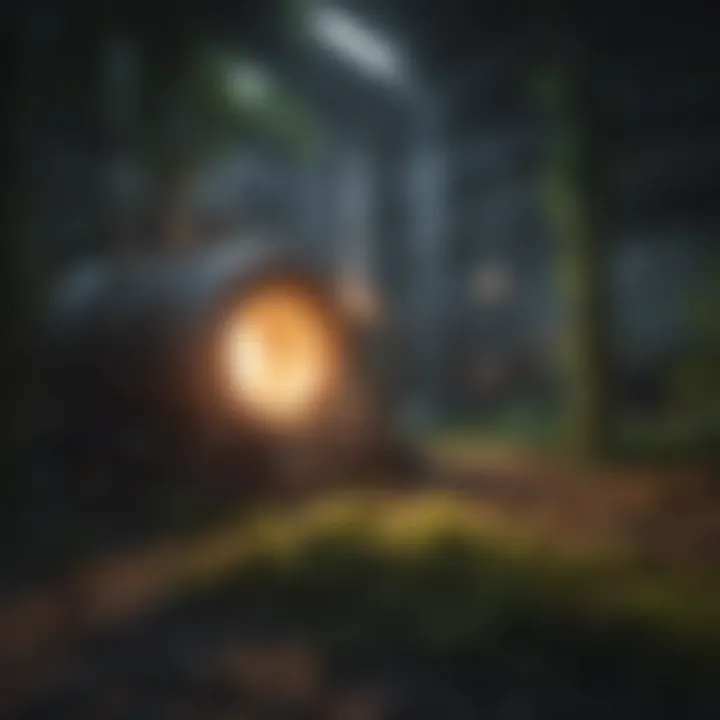
Feedstock Types
Different types of feedstocks feed into bioenergy production, offering unique characteristics and benefits that can contribute significantly to the bioenergy landscape.
Crop-based Feedstocks
Crop-based feedstocks include a variety of agricultural products such as corn, soybeans, and sugar cane. These crops are often chosen for their high yield and energy content. The main feature of crop-based feedstocks is their direct contribution to energy production, making them a popular choice in many biofuel scenarios. The advantage of these feedstocks lies in their relatively stable and predictable supply, which is essential for large-scale production. However, there are some downsides, such as competition with food supply, impacting food security in regions that heavily rely on these crops. This aspect can raise ethical concerns about land use and resource allocation, which schools of thought actively debate in the bioenergy sector.
Waste Materials
Waste materials encompass a wide range of organic matter that would otherwise end up in landfills, such as food waste, agricultural residues, and municipal solid waste. The key characteristic of using waste materials is their ability to mitigate waste disposal problems while simultaneously generating energy. This dual benefit makes waste materials a highly beneficial option, aligning waste management with energy production goals. A unique feature of this raw material is its low cost since it is typically considered waste, but it can also be inconsistent in quality and availability. Therefore, the processing methods need to be robust to effectively convert this feedstock into usable energy.
Forestry Residues
Forestry residues are another important type of feedstock for bioenergy sources. These include leftover wood materials from logging operations and wood processing industries. The primary advantage of forestry residues lies in their abundance and the fact that they can utilize by-products from timber operations that would otherwise go to waste. This makes them a wise ecological choice, as they enhance the overall efficiency of forest management. Yet, extracting this type of feedstock can be somewhat more contentious, mainly when it relates to forest health and biodiversity. Overharvesting can lead to negative impacts on ecosystems, emphasizing the need for well-managed sourcing strategies.
Sustainability Considerations
The sourcing of bioenergy inherently calls for sustainability considerations, tying back into how effectively bioenergy can serve as a renewable energy source. Understanding the implications of feedstock sourcing, from their cultivation to potential environmental impacts, is essential. Sustainable sourcing practices are vital in ensuring that bioenergy contributes positively to energy security without compromising natural ecosystems or food supplies. As global energy demands continue to rise, incorporating sustainability into sourcing practices remains a critical point of discussion in the bioenergy sector.
Bioenergy Production Processes
The production processes of bioenergy are pivotal to transforming raw materials into usable energy, laying the groundwork for the entire bioenergy sector. By understanding these processes, one can appreciate how bioenergy contributes to sustainable energy solutions and the ways in which it interacts with environmental and economic factors. Effective bioenergy conversion techniques can also influence the overall efficiency, viability, and acceptance of biofuels and biopower.
Anaerobic Digestion
Anaerobic digestion is a biological process that breaks down organic matter in the absence of oxygen. This process is particularly well-suited for managing waste materials, making it a practical option for the treatment of agricultural residues, food waste, and animal manure. The microorganisms involved convert these materials into biogas, primarily composed of methane and carbon dioxide, alongside digestate, which can be utilized as a nutrient-rich fertilizer.
Benefits of anaerobic digestion include:
- Waste Reduction: Significant reduction in the volume of waste, alleviating pressure on landfills.
- Renewable Energy Generation: The biogas produced can be harnessed to generate electricity and heat, or further processed into biomethane for use in vehicles or as pipeline gas.
- Soil Enrichment: The byproduct digestate enhances soil health, thus supporting regenerative agricultural practices.
Efforts to improve efficiency in anaerobic digestion processes can lead to higher yields of biogas and optimized management of feedstock. However, challenges remain, such as the initial investment costs and the need for technical expertise in operating and maintaining digestion facilities.
Fermentation
Fermentation is another cornerstone of bioenergy production, specifically for liquid biofuels like ethanol. Through this process, microorganisms (typically yeast or bacteria) convert sugars derived from biomass into alcohols and carbon dioxide. The sugars can be sourced from a range of materials including corn, sugarcane, and even agricultural waste.
Key aspects of fermentation include:
- Flexibility of Feedstocks: Fermentation can utilize a variety of carbohydrate sources, promoting the use of waste or low-value materials.
- Ethanol Production: The main product, ethanol, serves as a renewable gasoline substitute and can be blended with fossil fuels.
- Low Carbon Emissions: When compared to traditional fossil fuel production, fermentation-based processes can emit substantially lower carbon levels, propelling decarbonization efforts.
Nonetheless, fermentation isn't without its obstacles. The efficiency of converting sugars to ethanol can be hindered by various factors, such as competition with other processes occurring in biomass, leading to reduced yields.
Gasification
Gasification transforms biomass into syngas through a high-temperature process, wherein organic material is partially oxidized in a controlled environment. This syngas, consisting mainly of hydrogen and carbon monoxide, can be further processed into liquid fuels, chemicals, or electricity.
Features of gasification include:
- Versatility: Gasification can accommodate diverse types of feedstocks, including agricultural waste and forestry residues, making it less vulnerable to supply chain disruptions.
- High Efficiency: When optimized, gasification can achieve greater energy conversion efficiency than many other processes.
- Production of Valuable Products: Beyond energy, the syngas can be converted to a plethora of chemicals, broadening the economic potential of the technology.
However, gasification technology is challenged by high operational costs and complexity. Effective management of gasification systems and data-driven optimizations are essential for overcoming these hurdles and unlocking its full potential as a bioenergy production method.
Technological Advancements in Bioenergy
In the ever-evolving landscape of energy production, technological advancements in bioenergy play a pivotal role in enhancing efficiency and sustainability. The importance of these advancements cannot be overstated; they not only aim to increase yield but also strive to minimize the ecological footprint of bioenergy. As the world grapples with dwindling fossil fuel reserves and rising emissions, innovations in this sector offer promising avenues for a sustainable future.
Improved Conversion Technologies
With the continuous pursuit of greater efficiency, improved conversion technologies are the cornerstone of modern bioenergy production. These processes convert raw biomass into usable energy forms more effectively than traditional methods. For instance, advancements in thermochemical conversion, such as pyrolysis and gasification, allow for higher energy densities and more flexible energy outputs. By transforming solid biomass into gas or liquid fuels, these processes simplify transportation and storage, making bioenergy more accessible.
The integration of enzyme technology in the enzymatic hydrolysis of biomass is another leap forward. This method breaks down complex carbohydrates into sugars, which can then be fermented into biofuels. Notably, the use of genetically modified organisms has revolutionized this aspect. Certain strains of yeast can ferment sugars more efficiently, leading to higher ethanol yields.
"Improved conversion technologies not only enhance efficiency but also reduce waste generation, contributing to a closed-loop bioenergy economy."
Moreover, real-time monitoring systems are being implemented to optimize conversion processes. These systems use data analytics to adjust conditions swiftly, ensuring maximum output. Such advancements demonstrate how technology can effectively bridge gaps between biomass availability and energy demand.
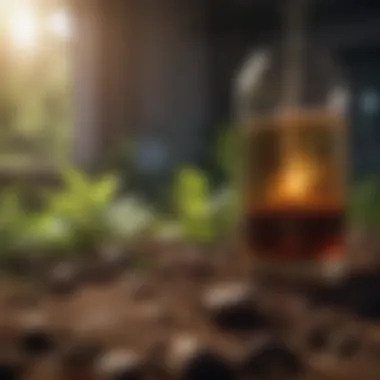
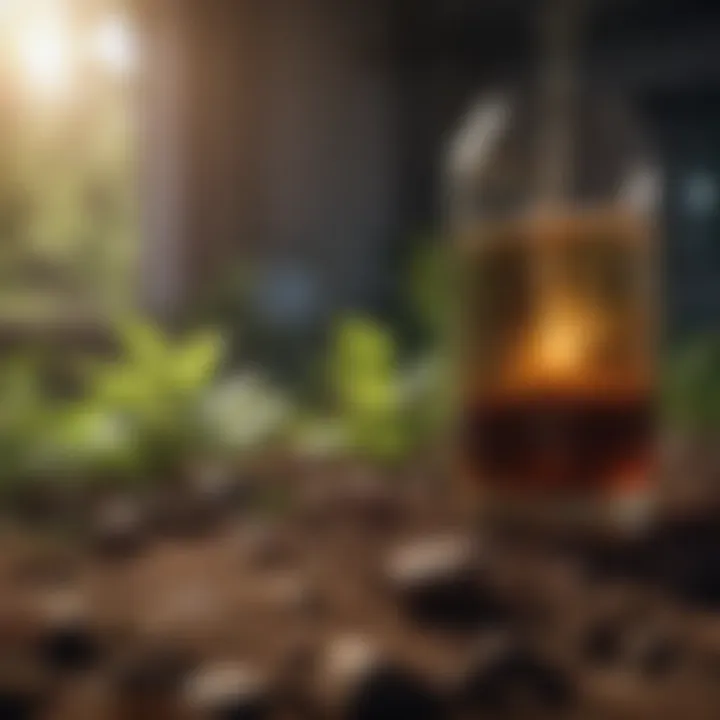
Biotechnology in Bioenergy
Biotechnology stands at the forefront of the bioenergy sector, fundamentally altering how we leverage biological materials for energy production. The utilization of biological processes in converting biomass into energy is nothing new; however, modern biotechnological methods have dramatically enhanced these processes, making them faster and more efficient.
Synthetic biology is a significant contributor, allowing scientists to engineer microorganisms to produce biofuels directly. By modifying the metabolic pathways of bacteria and algae, researchers can increase the production rates of fuels like biodiesel and bioethanol. For instance, researchers have successfully engineered algae that can directly synthesize biofuels from atmospheric CO2, presenting an intriguing solution for carbon remediation while producing energy.
Furthermore, the application of metagenomics allows for the exploration of uncharted microbial diversity in biomass breakdown, leading to the discovery of microbes with unique abilities to degrade complex lignocellulosic materials. This discovery aids in developing more efficient pretreatment processes that could yield higher amounts of fermentable sugars for biofuel production.
Environmental Impacts of Bioenergy
The environmental impacts of bioenergy are multifaceted and deserve careful consideration, especially as society grapples with the pressing need for sustainable energy solutions. Understanding these impacts becomes essential not only for policy makers but also for researchers and industry professionals. As bioenergy resources are tapped, the focus often narrows harmlessly to its renewable nature. However, a closer examination reveals a web of environmental considerations that can either strengthen or undermine the essence of bioenergy as a clean energy source.
Carbon Footprint Analysis
A critical aspect of assessing bioenergy's environmental footprint involves performing a carbon footprint analysis. The buzz around biofuel often highlights its potential to be carbon neutral, but reality is often more nuanced. Various factors can influence this balance. For instance, the entire lifecycle of bioenergy—from feedstock growth to production processes and final energy utilization—must be scrutinized. Here are a few key elements to keep in mind:
- Feedstock Selection: Different feedstocks generate varying levels of greenhouse gas emissions. For example, if you consider corn-based ethanol, the fertilizer inputs, land use changes, and transportation logistics contribute significantly to its overall carbon footprint.
- Land Use Changes: Converting forests or grasslands into bioenergy crops can result in substantial carbon release, negating the benefits of renewable sources. Sometimes, this shift can lead to "carbon debt," where the emissions from land use changes outweigh the benefits derived from bioenergies produced.
- Energy Use in Production: Processing biofuels can consume a great deal of energy, affecting the overall emissions profile. Advances in technology and better practices can reduce energy requirements, raising the stake for continuous innovation.
"The carbon footprint of bioenergy can fluctuate based on a multitude of factors, making continuous assessment crucial."
In essence, while bioenergy holds promise for reducing dependence on fossil fuels, a thorough carbon footprint analysis is vital to ensure that it delivers the environmental benefits it purports to.
Land Use and Biodiversity Effects
The interplay between bioenergy and land use is another significant concern that calls for rigorous examination. The shift towards bioenergy crops can have serious implications for land use and biodiversity. The following can shed some light on these impacts:
- Impact on Food Security: The competition for land between food crops and bioenergy production can raise red flags. If agricultural land is repurposed for energy crops, food production may get shortchanged, subsequently fueling food insecurity.
- Habitat Disruption: Converting biodiverse ecosystems into monocultures for bioenergy crops reduces biodiversity. When native habitats are altered, the flora and fauna that rely on those areas often suffer, leading to disrupted ecosystems.
- Soil Health: Continuous cultivation of a single crop can deplete soil nutrients, leading to increased reliance on fertilizers, which can in turn impact water quality and further exacerbate environmental issues.
Economic Implications of Bioenergy
Understanding the economic implications of bioenergy is crucial, as it directly intertwines with our energy future. The financial landscape for bioenergy is shaped by myriad factors including production costs, market potentials, and policy influences. By synthesizing these components, one gains insight into why bioenergy is not merely an environmental alternative but also a significant economic player.
Cost of Production
The cost of producing bioenergy is a labyrinth of variables. When assessing the cost, it’s essential to consider the different forms of bioenergy, as each presents unique production pathways that affect pricing.
- Feedstock Costs: The source material for generating biofuels has a direct impact on overall production expenses. For example, sourcing corn for ethanol might be less costly in an agricultural region compared to regions where sugarcane is more prevalent.
- Processing and Conversion: The technologies involved, such as anaerobic digestion or fermentation, have their inherent costs. Newer technologies often come with higher upfront investments, but they may yield better efficiencies in the long run.
- Transportation: Transporting feedstocks to processing facilities can be a silent killer of budgets. This is especially true in areas where farmers might be far from processing plants.
- Regulatory Costs: Navigating the regulatory landscape incurs expenses as well. Compliance with environmental standards or obtaining the necessary permits can add layers to production costs.
Cost-effective bioenergy production has been tantalizingly close yet just a tad out of reach for many. Industry players are constantly seeking innovations that may drive down these costs, and thus make bioenergy more competitive against fossil fuels.
Market Dynamics
Market dynamics for bioenergy are intricate and fluid, shaped by both external and internal influences. The adaptability of bioenergy markets reflects broader economic conditions, demand patterns, and technological advancements.
- Demand Growth: As global interest in renewable energy mounts, so does the demand for bioenergy. Institutions, including governments, are committing to decarbonization goals which translate into incentives for bioenergy production.
- Supply Chain Challenges: The bioenergy sector often faces supply chain hurdles, including logistics, availability of feedstock, and regional market access. Regions rich in biomass may find themselves at a competitive edge, but not all locales share this abundance, leading to uneven market conditions.
- Price Volatility: Like any commodity, bioenergy prices experience fluctuations. Factors such as crop yields, climate conditions, and global energy trends can sway prices heavily. This volatility can deter investment, as stakeholders may hesitate to commit resources when the financial landscape is unpredictable.
- Policy Impacts: Government policies such as subsidies or tariffs can distort market dynamics significantly. Favorable policies can spur growth and investment, while abrupt changes in regulation can leave the sector scrambling to adapt.
In summary, the economic implications of bioenergy encompass both costs of production and the fluctuating market dynamics. As we navigate this dynamic field, it’s clear that both challenges and opportunities exist. Engaging with these aspects can pave the way for a deeper understanding of bioenergy's vital role in our economy, aligning with broader sustainability goals.
Bioenergy in Policy Frameworks
When it comes to shaping the trajectory of bioenergy as a sustainable resource, policy frameworks play a pivotal role. The interplay between governance, regulation, and innovation influences the development of bioenergy technologies, the economic viability of production, and the social acceptance required for its proliferation. Thus, understanding the nuances of bioenergy in policy frameworks is essential in evaluating its future contributions to global energy needs.
Bioenergy policy encompasses a range of regulations and incentives designed to boost the production and use of biofuels, biogas, and other bioenergy forms. This can lead to multiple benefits including reduced greenhouse gas emissions, job creation in the renewable sector, and enhanced energy security. As such, these policies do not only reflect governmental priorities but also align with international commitments to combat climate change.
Global Policy Trends
On the global stage, we see an increasing emphasis on renewable energy driven by the recognition that fossil fuels deplete our natural resources while exacerbating environmental issues. Initiatives like the Paris Agreement have made it clear that governments around the world must diversify their energy sources. Consequently, many countries are adopting policies to promote bioenergy, each reflecting local contexts and priorities.
For example, in the European Union, the Renewable Energy Directive sets ambitious targets for member countries to increase the share of renewables in their energy mix. This includes incentives for biofuels and sustainability criteria that guide the sourcing and production of biomass. The goal is to ensure that bioenergy contributes to the reduction of emissions without compromising ecological integrity.
"Policies that promote bioenergy must balance environmental benefits with economic realities while accounting for societal impacts to be truly effective."
In contrast, countries like the United States have taken varied approaches based on regional needs. While the federal government supports biofuel production through the Renewable Fuel Standard, states like California have implemented their own more stringent policies aimed at reducing carbon emissions from transportation fuels. This differentiation allows for tailored responses that consider local resources and public sentiment.
Regional Case Studies
Diving deeper, let’s explore notable regional case studies that highlight the implications of policy decisions on bioenergy implementation:
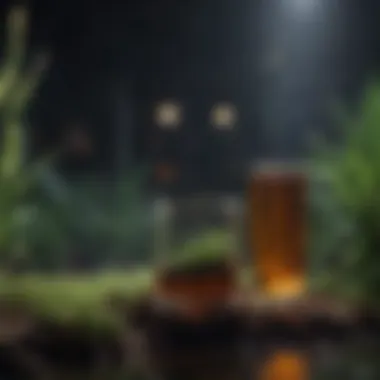
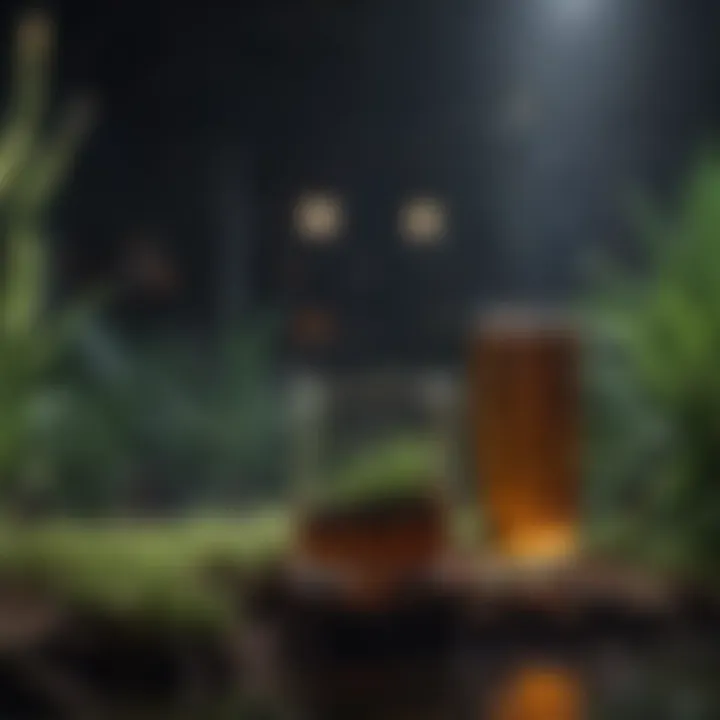
- Brazil: The evolution of Brazil's ethanol market offers an early model of government intervention. For decades, policies encouraging sugarcane cultivation have positioned the country as a leader in bioethanol production. This not only supports energy independence but also stimulates rural economies.
- Sweden: In Sweden, government policies have incentivized the use of wood pellets for heating, contributing significantly to their renewable energy goal. The coherent support for biomass has led to lower carbon emissions and a thriving market for biomass recovery industries.
- India: India’s bioenergy landscape is marked by diverse policies aiming to utilize organic waste and agricultural residues. The government's National Policy on Biofuels encourages the use of biofuels in transportation and seeks to achieve energy security while providing livelihood opportunities in rural areas.
These examples demonstrate how tailored policy can unlock the potential of bioenergy across different geographical landscapes. By examining varied strategies and outcomes, stakeholders can glean insights into best practices and possible pitfalls in advancing bioenergy agendas.
Bioenergy and Energy Security
The conversation surrounding bioenergy often shifts towards its worthiness in the arena of energy security. With growing concerns over fossil fuel dependence, bioenergy emerges as a shining beacon of hope. It plays a vital role in fortifying energy supply and mitigating vulnerabilities that can arise from geopolitical tensions or market fluctuations. As we continue to explore this complex relationship, it’s essential to delve into both the strategic advantages bioenergy offers and some considerations that come along with its integration into national energy policies.
Diversification of Energy Supply
One of the most compelling arguments for ramping up bioenergy production lies in its ability to diversify energy sources. When countries rely heavily on a narrow set of energy suppliers, they effectively place all their eggs in one basket. Bioenergy dilutes this risk by providing alternative avenues for energy generation. Bioenergy can be sourced from various feedstocks such as agricultural residues, forestry products, and municipal waste. This leads to a more resilient energy portfolio that can withstand external pressures.
Here are some specific benefits of diversification:
- Reduced reliance on fossil fuels: By integrating bioenergy into the energy mix, nations can decrease their dependence on oil and gas, which are often subject to price volatility and supply chain disruptions.
- Local energy production: Bioenergy encourages domestic energy production, which can lead to job creation in rural areas, thus contributing to regional economic growth.
- A buffer against geopolitical upheavals: When supply routes for fossil fuels are threatened, bioenergy serves as a reliable alternative, helping to maintain energy stability.
Resilience to Supply Shocks
Supply shocks happen for various reasons: natural disasters, political unrest, or economic downturns can all hamper traditional energy supplies. Bioenergy provides a safety net against these unpredictabilities. It’s often generated locally, meaning that disruptions in international markets have less impact on availability and prices. Moreover, the technological advancements in bioenergy production enhance its reliability.
- Distributed generation: Unlike traditional energy forms that rely on large, centralized plants, bioenergy can be produced on a smaller, localized scale. This decentralization means that even if one facility is impacted - say, by a storm - the overall energy production remains resilient.
- Sustainability of supply: With proper management of bioenergy resources, countries can ensure a continuous supply chain less sensitive to external shocks. For instance, using waste materials or dedicated energy crops can help build a reliable local supply.
Despite these advantages, it's crucial to note that bioenergy is not a silver bullet.
"Transitioning to bioenergy also requires us to confront issues like land use, resource availability, and public perception to foster a supportive ecosystem."
A holistic approach should be adopted to harness its potential effectively. This involves robust policies, community involvement, and technological innovations that can pave the way towards a more secure energy future.
In summary, bioenergy offers significant potential as a cornerstone for energy security. By diversifying energy supply and enhancing resilience to supply shocks, it not only provides a pathway towards reduced fossil fuel dependence but also contributes to energy independence, making it a vital subject for continued exploration.
Challenges Facing the Bioenergy Sector
In the realm of sustainable energy, the bioenergy sector stands at a crucial juncture. While bioenergy holds immense potential to transform our energy landscape, it faces several hurdles that can impede its growth and widespread adoption. This section casts a sharp focus on the challenges, emphasizing the technical barriers and public perception issues that currently shape the future dialogues around bioenergy.
Technical Barriers
When it comes to the technical barriers in bioenergy, one cannot overlook the complexities involved in its production and conversion. First off, the efficiency of conversion technologies like anaerobic digestion, fermentation, and gasification varies significantly. In many cases, these processes may not yield the energy returns that traditional fossil fuels provide, leading to skepticism regarding bioenergy's viability on a large scale.
Furthermore, scaling up production technologies to meet growing energy demands poses technical challenges. Many bioenergy facilities operate on a small scale and lack the capital needed for major advancements or expansions. Addressing these technical barriers requires hefty investments in research and development to improve the efficiency of conversion technologies, which could reshape market dynamics.
Additionally, the inconsistency in feedstock quality can pose significant problems. Different crops or waste materials boast varying compositions, impacting energy yields and thus the overall feasibility of energy production from these sources. This variability encourages a need for rigorous standardization, which, in itself, complicates the supply chain and production processes. These technical hiccups not only impact production but also raise questions regarding reliability and consistency in bioenergy supplies.
"Solving technical barriers in bioenergy is not just about improving yields; it's about building a resilient industry that can stand the test of time."
Public Perception Issues
While technological advancements are crucial, the public perception of bioenergy is equally important. Many folks view bioenergy as less clean or efficient compared to other renewable sources like wind or solar. These perceptions are often fueled by misinformation or a lack of understanding of the technology behind bioenergy.
Moreover, the perception that biofuels directly compete with food production creates a significant barrier. This viewpoint is not without merit, given that certain feedstocks, like corn and sugarcane, have been criticized for their implications on food prices and availability. The societal impacts of land use for bioenergy crops instead of conventional crop farming raise ethical questions, making the public particularly sensitive to these issues.
There’s also a hesitance regarding the environmental impacts of bioenergy. While bioenergy is often marketed as a green alternative, some studies suggest that its production and use can still lead to deforestation, loss of biodiversity, and greenhouse gas emissions. Addressing these concerns through clear communication can prove vital in reshaping public opinion and building trust in the bioenergy sector.
Overall, the challenges facing the bioenergy sector are multifaceted. It’s essential for stakeholders to engage in open dialogues, providing transparent information, to help bridge the gaps in understanding. Tackling these challenges requires not only technical innovations but also a concerted effort to reshape public perception to foster an environment conducive for bioenergy to flourish.
Future Prospects for Bioenergy
Considering the current global energy landscape, the future prospects for bioenergy hold significant promise as we seek cleaner and more sustainable alternatives to fossil fuels. As nations grapple with climate change and the implications of energy dependency, bioenergy offers a versatile solution. By utilizing a diverse array of organic materials, bioenergy can play a crucial role in achieving energy independence and mitigating negative environmental impacts.
Research Directions
The advancement of bioenergy largely hinges on targeted research initiatives that address both efficiency and sustainability. In this realm, some promising research avenues include:
- Feedstock Innovation: Scientists are looking at optimizing existing feedstock options and exploring new ones, such as algae and non-food crops. This could potentially reduce competition with food production while enhancing yield.
- Conversion Technologies: Improving the efficiency of conversion processes—be it through anaerobic digestion, gasification, or fermentation—remains a key focus. Developing catalysts and enhancing enzymatic processes can significantly boost production rates, making biofuels more competitive.
- Carbon Capture Integration: Researchers are exploring ways to integrate carbon capture with bioenergy systems to further diminish carbon emissions during energy production. Such methods could create a negative carbon footprint, blending bioenergy with larger climate goals.
- Policy and Economic Models: Investigating how different policies affect bioenergy adoption can also provide insights. Researching policies that incentivize sustainable practices and trialing new financial models could help build better markets for bioenergy products.
The future is not without challenges, however. Ensuring the scalability of these innovations while maintaining ecological balance requires ongoing investigation.
Market Forecasts
Market forecasts indicate a gradual but definitive uptick in bioenergy adoption around the globe. According to several industry reports, the biofuels market is expected to reach substantial figures, influenced by a blend of factors:
- Government Mandates: Many countries are increasing their renewable energy targets, which will likely propel bioenergy to the forefront of energy discussions. For example, the EU's Renewable Energy Directive mandates specific incorporation rates for biofuels.
- Technological Costs: As technologies advance and become more affordable, small-scale bioenergy systems could emerge, empowering local communities to produce their own energy. This could change the dynamics of bioenergy consumption fundamentally, pushing it toward a decentralized future.
- Investor Interest: With shifting social sentiment towards sustainability, there’s burgeoning investor interest in renewable energy. Bioenergy projects are finding new backing, which bodes well for future innovations and market expansions.
- Impact of Climate Change Mitigation Policies: As more entities commit to net-zero emissions, bioenergy’s role expands, serving not just as an energy source but also as a crucial strategy in carbon management.
"The trajectory for bioenergy production shows a promising convergence of technology, policy, and market forces, setting the stage for a more sustainable energy paradigm."
Overall, the forecasts paint a picture of a dynamic sector that is poised to evolve rapidly, offering both opportunities and challenges as it integrates more deeply into global energy systems. Understanding this landscape is essential, as bioenergy stands at a nexus of environment, economy, and energy security.