Understanding Permafrost: Characteristics and Impacts
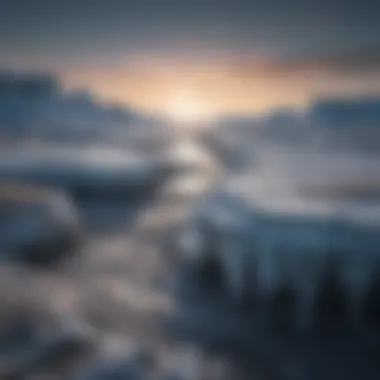
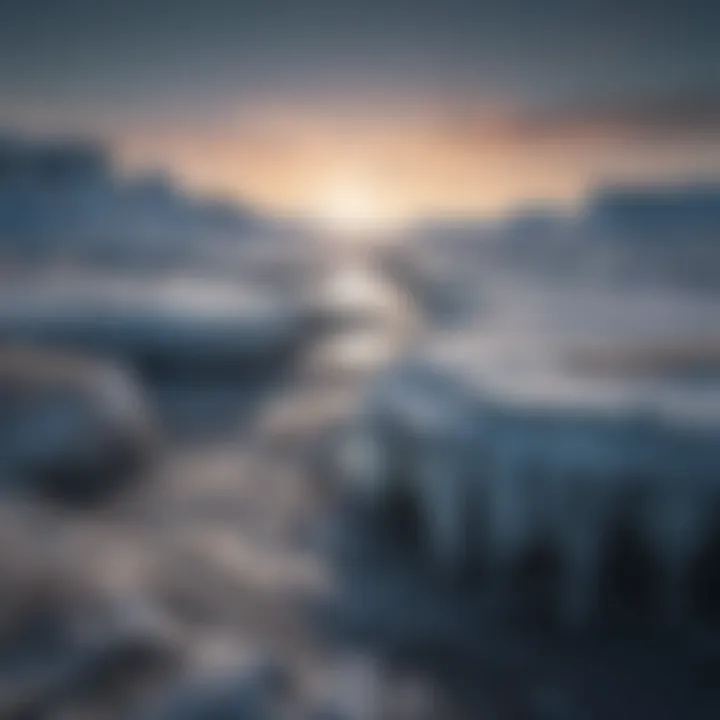
Intro
Permafrost is a critical topic in today's discourse on climate change. Understanding its characteristics and implications is essential for both ecological health and infrastructure stability. This article covers the depth of permafrost, a frozen layer of soil that remains at or below 0°C for two or more consecutive years. Its significance stretches from the northern tundras to alpine regions of the globe. As climate change accelerates, thawing permafrost presents a myriad of environmental challenges.
The investigation into permafrost reveals not only its biological and geological features but also the cascading effects of its degradation. Thawing releases greenhouse gases, which are potent contributors to global warming. In addition to ecological concerns, the impacts extend toward socio-economic factors affecting communities living in regions with extensive permafrost coverage.
This article will explore the characteristics of permafrost, the consequences of its thawing, and innovative approaches to monitor and manage permafrost dynamics. Through this detailed examination, readers will gain greater insight into the vital role permafrost plays in Earth's climate system.
Research Overview
Summary of Key Findings
Research indicates that permafrost occupies about 24% of the Northern Hemisphere's land surface. Various studies have shown that this vast reserve of carbon, which is trapped in frozen organic matter, poses significant risks when thawed. It could release between 1,000 to 1,500 billion tons of carbon dioxide and methane into the atmosphere.
Additionally, the rate of thawing can vary extensively based on geographical region, depth of the permafrost, and climate conditions. Regions like Siberia are experiencing some of the fastest rates of thawing, contributing to increased erosion and instability of ecosystems.
"The release of greenhouse gases from thawing permafrost can significantly affect the global climate system, exacerbating climate change."
Importance of the Research
The urgency of studying permafrost is underscored by its role in climate regulation. Understanding permafrost dynamics can inform policy-making and help in developing mitigation strategies. Furthermore, this research enables us to predict future climate scenarios and prepare adaptive measures for at-risk ecosystems and human communities.
Methodology
Study Design
Most studies on permafrost involve a combination of field observations and remote sensing technologies. By employing both methods, researchers can analyze temperature fluctuations, soil moisture, and vegetation changes over time.
Data Collection Techniques
Data on permafrost characteristics are collected using various techniques such as:
- Ground-penetrating radar for mapping
- Temperature sensors installed at various soil depths
- Satellite imagery for large-scale coverage of thawing zones
These methods facilitate comprehensive understanding and monitoring of permafrost as it responds to climate change also enabling real-time assessments.
Defining Permafrost
Understanding permafrost is crucial for a comprehensive view of ecological and geological processes, especially in the context of climate change. Permafrost refers to soil or rock that remains frozen for two or more consecutive years. It serves as a vital component of the Earth's cryosphere and has significant implications on global climate patterns, ecosystems, and even human infrastructure in polar and subpolar regions.
One of the essential elements of defining permafrost is its geographical and climatic context. Found primarily in high-latitude regions, such as the Arctic and Antarctic, permafrost is not merely localized ice. It represents a unique bond between the ground and the varied ecosystems it supports. Variability in its thickness and extent can lead to different environmental scenarios, affecting local flora and fauna.
Additionally, understanding permafrost encourages awareness of its hydrological role. As water interacts with the frost, it influences local waterways and can affect weather patterns in adjacent areas. Therefore, the study of permafrost is not just about frozen ground; it encapsulates a myriad of processes crucial for sustaining life and mediating climate.
Basic Characteristics
Permafrost has distinct characteristics that differentiate it from unfrozen soil. These include its temperature, longevity, composition, and depth. Typically, any soil that maintains a temperature below 0°C for at least two years is classified as permafrost. Its depth can range significantly, from less than a meter to over 1,500 meters, depending on local geography and climate.
The thermal regime of permafrost can affect surrounding areas. For instance, continuous permafrost remains perpetually frozen and is often found in tundra areas where there is minimal vegetation. On the contrary, discontinuous permafrost experiences seasonal thawing, making it sensitive to climatic variations.
Furthermore, permafrost can exhibit features such as ice wedges, frost boils, and sorted circles. These morphological traits enhance our understanding of soil formation processes and reveal the historical climate conditions of the regions they inhabit.
Composition and Structure
The composition and structure of permafrost are complex and encompass various materials. It comprises a mixture of soil, ice, sediment, and organic matter. The ice content can vary widely, often exceeding 90% in certain locations, which significantly influences the mechanical properties of the ground.
The structural arrangement of permafrost layers can include an active layer above the permafrost. This layer undergoes seasonal thawing and refreezing, contributing to local hydrology and ecological dynamics. The transition between the active layer and permafrost is marked by a clear thermal gradient which responds to external temperature changes.
It is essential to study the composition of permafrost not only for ecological reasons but for its role in global carbon cycles. Stored organic carbon may be released as greenhouse gases if thawing occurs, further impacting climate change dynamics.
In summary, defining permafrost is foundational for understanding diverse climatic and ecological implications. It encompasses a wide range of characteristics and compositions, warranting in-depth research into its impact on Earth’s systems.
Formation of Permafrost
The formation of permafrost is an important topic that highlights the conditions necessary for this unique phenomenon to arise. Understanding how permafrost develops helps clarify its longevity and ecological impacts. Permafrost influences not only local ecosystems but also broader climate dynamics due to the stored carbon and other compounds within it. Its existence is critical for certain habitats and can affect infrastructure, especially in the context of climate change.
Historical Context
The history of permafrost formation dates back thousands of years. During glacial periods, extensive ice coverage shaped the landscape. As these glaciers retreated, the underlying soil began to freeze, leading to the onset of permafrost. Researchers believe that permafrost can be divided into two main periods:
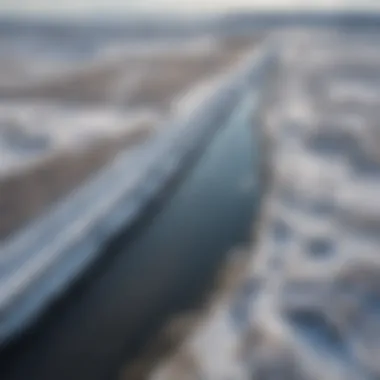
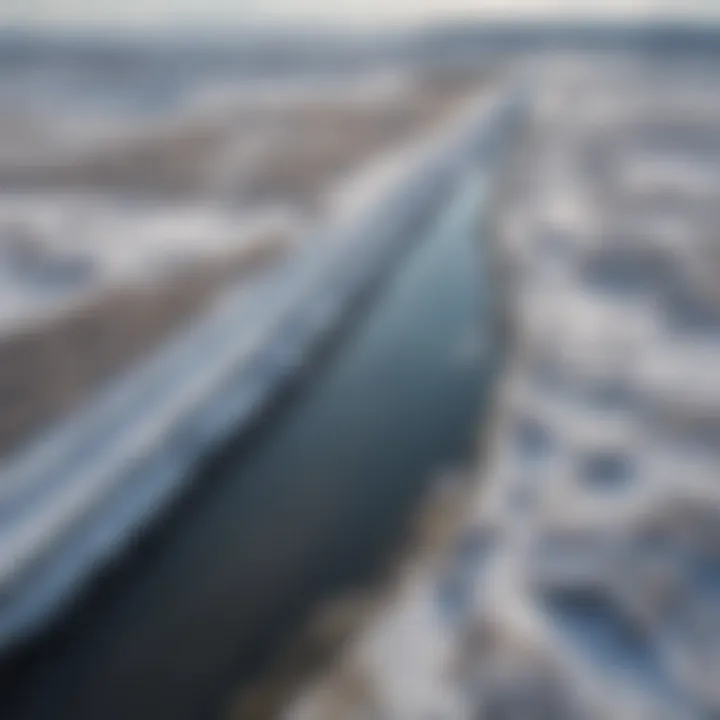
- Pleistocene Epoch: Most of today's permafrost formed during this time, under significantly cooler climates.
- Holocene Epoch: Following the last Ice Age, permafrost continued to exist but with changing conditions as temperatures began to rise.
The analysis of permafrost's ages holds relevance in understanding past climate conditions. Ice cores and sediment layers offer insights into historical temperature and moisture levels.
Climate Conditions Required for Formation
Various climate factors critically influence the formation of permafrost. Specifically, the following conditions are essential:
- Cold Temperatures: Regions must experience long winters with consistent subzero temperatures. Typically, this occurs in the Arctic and sub-Arctic zones.
- Precipitation Levels: While not all cold regions have permafrost, lower precipitation can aid in its formation. Excess moisture often leads to insulation effects, making it difficult for soil to freeze.
- Soil Properties: Certain soil types, such as silts and clays, promote the retention of moisture, enhancing the freezing process.
These climate-related factors create a delicate balance necessary for establishing and maintaining permafrost. Any fluctuations in climate, such as those induced by global warming, can directly affect permafrost stability.
Geological Factors Influencing Permafrost
Geology plays a crucial role in the formation and distribution of permafrost. Important factors include:
- Topography: Elevation and slope can affect how cold air moves across landscapes, with valleys often being colder than elevated regions.
- Soil Composition: The mineral composition of soil influences thermal conductivity. For example, silty soils generally freeze more effectively than sandy soils.
- Hydrology: Groundwater levels within a region can either promote or inhibit permafrost formation. Saturated soils have different freezing dynamics compared to drier soils.
These geological factors interact intricately with climate conditions, creating a complex environment where permafrost can develop or dissipate. Understanding these aspects is essential for comprehending the broader implications of permafrost in light of changing global climates.
Geographical Distribution of Permafrost
Understanding the geographical distribution of permafrost is crucial in this discussion about its characteristics and impacts. Permafrost covers a significant area of the Earth’s surface and influences both local and global ecosystems. This section explores where permafrost exists globally and highlights the regions where permafrost presence is most significant. Recognizing these geographical patterns helps grasp the broader implications of permafrost dynamics, especially in the context of climate change and its effects on the environment.
Global Distribution Patterns
Permafrost is typically found in high-latitude regions. Approximately 25% of the northern hemisphere is underlain by permafrost. The majority is located in Alaska, Canada, Russia, and parts of northern Europe and Asia. Its distribution is closely tied to the climatic conditions of these areas, predominantly characterized by cold temperatures and limited sunlight.
Some key factors influencing global distribution patterns include:
- Temperature: Regions with average annual temperatures below 0°C are more likely to have permafrost.
- Elevation: Higher elevations can experience cooler temperatures conducive to permafrost formation even in lower latitudes.
- Soil Composition: The presence of specific soil types can elevate the risk of permafrost formation and maintenance.
Understanding these patterns is vital, as permafrost plays a critical role in the Earth's climate system and can significantly influence local biodiversity.
Regions of Significant Permafrost Presence
The most prominent areas of permafrost include:
- Alaska: This state has extensive permafrost coverage, particularly in the northern and western regions.
- Canadian Arctic: Significant portions of Yukon, Northwest Territories, and Nunavut contain permafrost, with extensive impacts on local ecosystems.
- Siberia: Perhaps the largest stretch of continuous permafrost, Siberia affects atmospheric conditions and carbon cycles, making it a focal point for climate studies.
- Greenland: This region is witnessing changes in its permafrost dynamics as climate conditions shift, impacting glacial melt.
Understanding the geographical distribution of permafrost allows for better assessment of the effects of thawing, which can have profound impacts on global carbon cycles, infrastructure, and indigenous cultures that depend on stable land conditions.
Types of Permafrost
Understanding the types of permafrost is crucial for grasping the overall ecosystem dynamics in regions where it occurs. The classification of permafrost into continuous and discontinuous types reflects variations in climate, geological attributes, and ecological impacts. These distinctions play a significant role in how permafrost interacts with its environment, influences carbon storage, and affects local communities.
Continuous Permafrost
Continuous permafrost covers vast areas of the Arctic region. This type is characterized by a consistent layer of frozen soil, often remaining below 0°C regardless of seasonal changes. The presence of continuous permafrost impacts not only vegetation patterns but also water drainage and the geographical layout of ecosystems.
Continuous permafrost is generally found in areas with low average temperatures. When thawed, this permafrost releases greenhouse gases, mainly carbon dioxide and methane, into the atmosphere—contributing to climate change.
Key Features of Continuous Permafrost:
- Temperature Stability: It typically remains stable and deeply frozen throughout the year.
- Ecosystem Impacts: Limited biodiversity, as few plant species can thrive under such conditions.
- Geographical Extent: Makes up the majority of permafrost in regions like northern Canada, Alaska, and Siberia.
Overall, continuous permafrost represents a fragile balance in the ecosystem. Its thawing can have dire consequences, making this type a critical focus for research and monitoring.
Discontinuous Permafrost
Discontinuous permafrost, in contrast, occupies areas where permafrost is not consistently present. It exists in patches interspersed with unfrozen soil. The extent and depth of discontinuous permafrost can vary drastically, influenced by elevation and proximity to bodies of water.
The dynamics of discontinuous permafrost are complex. While some areas may support active ecosystems, others may face challenges as climate change leads to increased thawing. The consequences of thawing in these regions can lead to increased release of stored organic carbon, further aggravating global warming.
Characteristics of Discontinuous Permafrost:
- Spatial Variability: Varies greatly, with areas of both frozen and unfrozen soil.
- Biodiversity: Higher than in continuous permafrost areas, allowing for a variety of plant and animal life.
- Impact of Thawing: Thawing can lead to issues such as land subsidence, affecting infrastructure and ecosystems.
Recognizing the presence of discontinuous permafrost is vital for understanding local climate feedback effects and developing appropriate mitigation strategies.
Soil and Ice Interactions
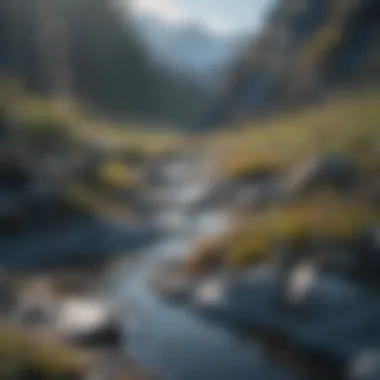
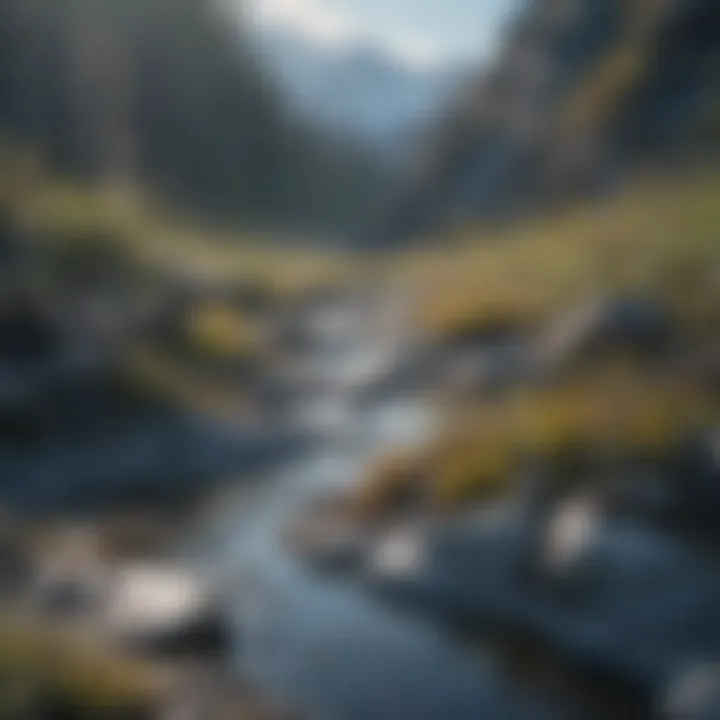
The interactions between soil and ice in permafrost regions are fundamental for understanding its role in climate processes. Soil composition, moisture levels, and temperature all influence how ice forms, melts, and persists in the permafrost layer.
When soil is saturated, ice can form and lead to ice-rich permafrost. This can create unique landforms such as ice wedges and thermokarst, which are critical for local biodiversity.
Significant Aspects of Soil and Ice Interactions:
- Hydrology: Water movement in soil affects permafrost stability and quality.
- Carbon Cycling: Ice formation can trap organic matter, impacting carbon storage.
- Terrain Changes: Thawing alters topography and affects plant and animal habitats.
The interactions between soil and ice present a complex system that requires careful study to predict future changes due to global warming.
Understanding the types of permafrost and their interactions is essential. As climate change accelerates, these regions face significant risks that can impact global climate systems.
Ecological Impacts of Permafrost
The ecological impacts of permafrost are profound and multifaceted. Understanding these impacts is essential not just for the ecosystems they affect, but also for humans who depend on these systems. Permafrost holds significant quantities of organic carbon, which when thawed, can influence global carbon cycles. This not only affects biodiversity but also the resilience of various habitats. The interplay between permafrost, climate change, and ecosystems creates a complex web of interactions demanding careful consideration.
Biodiversity and Habitat Formation
Permafrost plays a crucial role in shaping habitat types and biodiversity. In regions where permafrost is present, the ground conditions create unique ecosystems. These areas often support specialized flora and fauna adapted to extreme conditions. For instance, tundra ecosystems flourish in these settings, fostering species such as mosses and lichens which rely on the cold, stable conditions of permafrost.
When the permafrost thaws, the habitat dynamics change. Thawing can lead to increased water accumulation, creating new wetland areas. This may benefit some species while threatening others. The sudden influx of nutrients from decomposing matter can drive changes in plant composition, potentially favoring invasive species that adapt quicker to warmer conditions.
- Key Points on Biodiversity Impact:
- Unique species may become endangered due to habitat loss.
- Changes in plant communities can disrupt food webs.
- New wetlands may alter migration patterns of animals.
Carbon Storage and Release Dynamics
Permafrost acts as a significant carbon sink. It stores vast amounts of organic carbon that have accumulated over thousands of years. However, the dynamics of this storage are shifting as climate changes. Thawing permafrost releases greenhouse gases like carbon dioxide and methane into the atmosphere. This process can significantly accelerate climate change.
The relationship between permafrost and carbon is a feedback loop. As permafrost thaws, more carbon is released, enhancing warming, which in turn leads to further thawing. Understanding these dynamics is crucial. It highlights the importance of monitoring permafrost systems not just for ecological health, but also for broader climate implications.
"Thawing permafrost could potentially release more than 1,000 billion tonnes of carbon into the atmosphere, a staggering amount that could amplify global warming."
Considerations of Carbon Dynamics:
- Thawing Rates: Different regions experience varying thawing rates, impacting carbon release.
- Feedback Mechanisms: The interplay between carbon release and climate change requires urgent attention.
- Mitigation Strategies: Strategies to manage and monitor permafrost are essential to address these challenges.
Human Interactions with Permafrost
The relationship between human activities and permafrost is significant and multifaceted. Understanding how permafrost influences and is influenced by human endeavors is critical for sustainable development in affected regions. This section explores the challenges and implications arising from human interactions with permafrost, emphasizing their infrastructure challenges and the cultural significance of these frozen grounds for indigenous peoples.
Infrastructure Challenges and Risks
As climate change leads to permafrost thawing, the integrity of infrastructure in permafrost regions is at stake. Buildings, roads, and pipelines face various risks associated with changing ground stability. Thaw can cause ground subsidence, resulting in structural damage and costly repairs.
- Ground Instability: Thawing permafrost can weaken the ground, making it unable to support existing structures. For instance, roads that were once stable may crack or become uneven, complicating transportation and logistics.
- Flooding Risks: Thawing can increase surface runoff and flooding. This is problematic for communities and infrastructure that rely on stable ground conditions.
- Maintenance Costs: Ongoing maintenance becomes critical. Regular assessments and retrofitting efforts are essential to ensure infrastructure remains safe and functional, which increases financial burden.
In addition, sectors such as oil and gas, which operate in these remote areas, may face significant operational risks, including pipeline breaks and spills from collapsing infrastructure due to thawing. Such incidents have long-term environmental effects, which further complicates human interactions with permafrost.
Cultural Significance for Indigenous Peoples
For indigenous peoples, permafrost is more than a geological phenomenon; it intertwines with cultural identity and traditional practices. Indigenous communities rely on the land for their livelihood, including hunting, fishing, and gathering. The stability of permafrost affects their way of life significantly.
- Traditional Knowledge: Indigenous knowledge systems recognize the importance of permafrost in ecosystem dynamics. This knowledge guides sustainable practices for resource use and preservation.
- Cultural Heritage: Many indigenous traditions and stories are closely tied to the land. Thawing permafrost can disrupt geographical features that hold cultural significance, impacting practices passed down through generations.
- Adaptation Strategies: Indigenous communities are developing adaptation strategies to cope with these changes. This includes shifting hunting grounds and adjusting seasonal activities based on permafrost conditions.
"Understanding the effects of changing permafrost is essential for preserving cultural heritage and ensuring that communities can continue their traditional ways of life in a warming world."
In summary, recognizing the diverse human interactions with permafrost highlights the crucial balance between development and preservation. Addressing infrastructure challenges while respecting the cultural significance for indigenous peoples is vital in fostering resilience in the face of ongoing climate change.
The Effects of Climate Change on Permafrost
Permafrost is crucial in understanding climate dynamics. Its effects become more pronounced as global temperatures rise, complicating the interactions between the frozen ground and the environment. Thawing permafrost influences biodiversity, carbon emissions, and human infrastructure. As climate change progresses, these factors must be closely monitored to evaluate the long-term implications for ecosystems and society.
Thawing Dynamics
Thawing dynamics of permafrost are complex and multifaceted. As temperatures increase, the frozen ground begins to thaw, allowing for the release of stored greenhouse gases such as methane and carbon dioxide. These gases contribute significantly to climate change, creating a feedback loop. The release of these gases can accelerate the warming trend, leading to even more permafrost thawing.
- Factors Influencing Thaw: Thawing rates can vary based on location, soil types, moisture levels, and vegetation cover. In some areas, increased vegetation can insulate the soil, slowing down thawing. In others, the loss of insulating snow layers can expose the ground and speed up the melting.
- Impacts on Ecosystems: As permafrost thaws, the landscape changes. This alters habitats and could lead to the disappearance of certain species unable to adapt. It also impacts freshwater systems, as changing water levels can disrupt local aquatic life.
Feedback Mechanisms in the Climate System
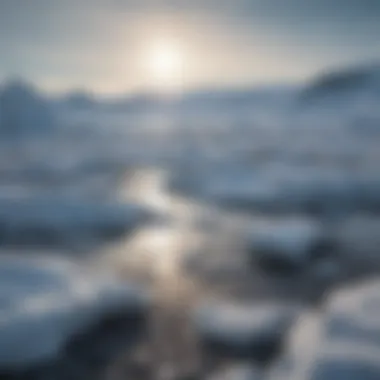
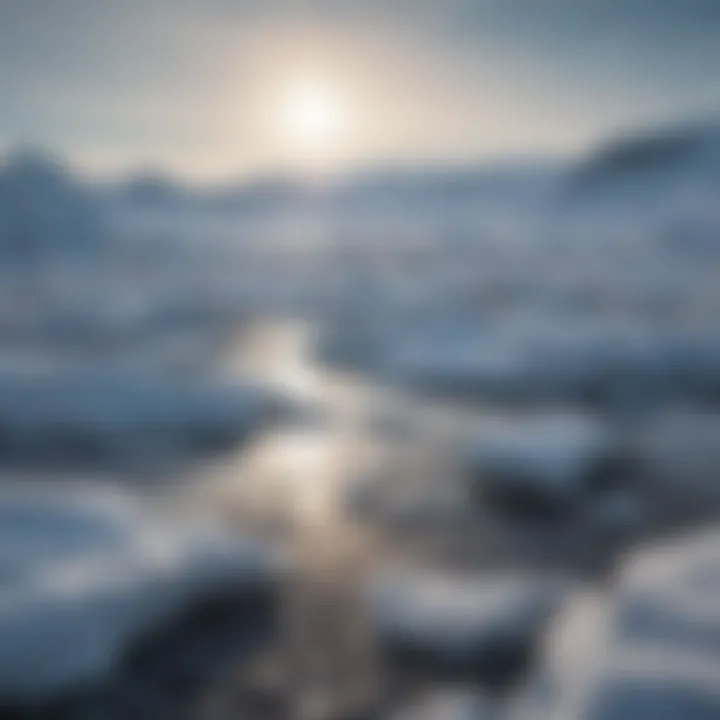
The feedback mechanisms involving permafrost are significant. When permafrost thaws, it releases not only carbon but also alters local climates. This can lead to changes in precipitation patterns, impacting vegetation and the overall climate system.
"The thawing of permafrost is a critical issue that influences the broader climate system, creating adjustments to weather patterns and changing precipitation."
Key feedback effects include:
- Altered Albedo: Thawing can expose darker surfaces, reducing the albedo effect and increasing heat absorption, which may lead to further warming.
- Water Dynamics: Changes in permafrost can affect groundwater levels, which alters both local and regional hydrology.
- Transportation Pathways: The thaw can weaken the structural integrity of the ground, which poses risks to infrastructure such as roads and buildings, making it essential for future planning.
In summary, the effects of climate change on permafrost are profound. Monitoring these changes helps in understanding future implications, emphasizing the urgent need for policies addressing climate-related challenges.
Monitoring and Research Innovations
Innovations in monitoring and research are critical to understanding permafrost. These advancements offer insights into how permafrost responds to changing climate conditions. Innovative approaches allow scientists to gather data that might not be visible through traditional methods. This information is essential not only for studying permafrost but also for predicting its future behavior in the context of global climate change.
Effective monitoring techniques are necessary to provide accurate data regarding the status and changes in permafrost. This data informs stakeholders from various sectors—government, research, and local communities—about potential risks and adaptation strategies. Researchers are employing various technologies to advance our understanding of permafrost systems, which can have widespread implications for environmental policy and natural resource management.
Remote Sensing Techniques
Remote sensing represents a pivotal development in permafrost research. This method allows researchers to evaluate large and often inaccessible regions. Satellites equipped with advanced sensors can capture images and data about the surface and subsurface of permafrost areas. This is especially useful in monitoring spatial distribution and identifying heat changes over time.
Some key benefits of remote sensing techniques include:
- Large area coverage: Remote sensing allows for assessment over extensive landscapes that would be difficult to survey on the ground.
- Time efficiency: Rapid data acquisition means scientists can monitor changes frequently, which is crucial given the rapid thawing of permafrost.
- Cost-effectiveness: With fewer field trips necessary, research costs can be reduced significantly.
Techniques such as Synthetic Aperture Radar (SAR) and thermal infrared imagery provide valuable insights into thermal dynamics. They help in understanding how temperature fluctuations affect the integrity of permafrost layers. Additionally, remote sensing can facilitate the study of vegetation changes in permafrost regions, as shifts in ecosystems often correlate with permafrost stability.
"Innovative remote sensing technologies empower scientists to monitor permafrost in real time, helping to predict its behavior in our changing climate."
Field Research Methods
While remote sensing is immensely helpful, field research remains a fundamental aspect of studying permafrost. Ground-based studies furnish detailed information that satellite data might not capture. Field research methods include soil sampling, temperature measurements, and ground-penetrating radar surveys.
Key elements of effective field research are:
- Direct Data Collection: In situ measurements provide accurate and specific data on soil composition, moisture content, and thermal properties.
- Longitudinal Studies: Establishing long-term observation sites allows researchers to track changes over time. These studies help in understanding the patterns that may not be evident through short-term observations.
- Interaction with Local Ecosystems: Field research enables scientists to assess direct impacts on flora and fauna, which may offer insights into broader ecological consequences.
Together, remote sensing techniques and field research create a nuanced picture of permafrost conditions. Such comprehensive approaches are essential for crafting effective mitigation strategies and enhancing our understanding of climate-related impacts.
Mitigation Strategies and Future Solutions
Mitigation strategies and future solutions are critical in addressing the challenges posed by permafrost thawing. As the global temperatures rise, the integrity of permafrost can become compromised, leading to a cascade of ecological and infrastructural challenges. This section focuses on the need for targeted interventions and proactive measures. Understanding the implications of permafrost deterioration helps inform effective policy and technological developments.
Policy Implications and Recommendations
Effective policy frameworks are vital for addressing the issue of permafrost loss. Policymakers must consider the long-term consequences of thawing and the associated greenhouse gas emissions, particularly methane and carbon dioxide. Some recommendations to guide policy include:
- Implementing regulations to reduce greenhouse gas emissions: Countries should commit to international treaties designed to limit overall carbon emissions.
- Funding scientific research: Governmental and non-governmental organizations must invest in research that focuses on permafrost dynamics and their implications.
- Encouraging local community input in decision-making: Engaging indigenous populations and local communities can provide valuable insights into the impacts of permafrost changes.
Research shows that localized knowledge is essential in creating effective mitigation strategies, as these communities often have historical awareness of environmental changes.
In addition, developing adaptable land-use policies can aid in minimizing infrastructural risks.
Technological Innovations for Climate Adaptation
Technological advancements are essential for monitoring and adapting to permafrost changes. Innovations can aid in data collection and analysis, allowing for timely responses to emerging issues. Some key technological innovations include:
- Remote sensing technology: This technology uses satellite imagery to monitor permafrost areas and assess thawing extent, which helps in creating predictive models.
- Ground-penetrating radar: This method provides insights into the subsurface conditions of permafrost, allowing researchers to assess changes in its structure over time.
- Climate modeling software: Advanced simulations can help predict future scenarios based on current thawing rates and temperature elevations.
Investing in these technologies not only enhances understanding but also allows for robust responses to climate challenges.
The End
The conclusion serves as a vital element in synthesizing the entirety of the discussion on permafrost. It encapsulates essential findings, emphasizes the significance of ongoing research, and highlights the path forward in understanding this critical component of our climate system.
Summary of Key Findings
In reviewing the complexities of permafrost, several key findings emerge:
- Composition and Characteristics: Permafrost displays unique characteristics due to its formation under specific climatic conditions. Knowing its structure and composition is crucial in understanding its role in the environment.
- Impact on Ecosystems: Thawing permafrost affects biodiversity, contributing to significant ecological changes. The release of stored carbon poses a risk to climate stability, making the study of permafrost even more imperative.
- Human Endeavors and Challenges: Human infrastructure must adapt to the challenges posed by thawing permafrost. This discussion reminds stakeholders of the importance of planning that incorporates these realities.
- Research Innovations: Advances in technology provide insights into permafrost dynamics. Methods like remote sensing and advanced modeling can aid in monitoring and addressing changes effectively.
"Permafrost is not just frozen ground; it is an integral part of our Earth’s climate puzzle, affecting everything from the atmosphere to ecosystems and human life."
Future Research Directions
Looking ahead, research on permafrost should prioritize several directions:
- Long-term Monitoring: Establish long-term observational studies to track changes and their implications systematically. This can help in developing models for better climate predictions.
- Impact Assessment: More studies are needed to assess the ecological and socio-economic impacts of permafrost thaw. Understanding these aspects can guide policy and adaptive measures.
- Mitigation Strategies: Research on technological innovations aimed at mitigating the impacts of thawing permafrost is essential. This encompasses both policy recommendations and practical applications.
- Interdisciplinary Collaboration: Encourage collaboration between scientists, policymakers, and indigenous communities. This can foster knowledge sharing and result in comprehensive strategies tailored to local needs.